Abstract
Background and purpose. To study the impact of the 4DCT imaging technique on radiotherapy planning for pancreatic carcinoma. To evaluate the possibility of IMRT/IGRT to increase the dose to PTV subvolume. Material and methods. Contrast-enhanced 4DCT scans of 15 patients (PTs) with unresectable pancreatic cancer were acquired. A 4DCT based PTV (4D-PTV) was created by the convolution of contours and then expanded for geometric uncertainties; a standard PTV (STD-PTV) was derived from a single CTV plus conventional margins. Two 3D conformal treatment (3DCRT) plans and one Helical Tomotherapy (HT) plan were generated with a prescription of 60 Gy. Regarding the 3DCRT plans, the 4D-PTV was considered as the target volume for one, and the STD-PTV for the other; the HT plans were performed only for 4D-PTV. Twelve of 15 PTs were admitted to a Phase I hypofractionated study (15 fractions). The prescribed dose was 44.25 Gy to the 4D-PTV and the PTV subvolume around vascular involvement was boosted from 50 to 55 Gy; before treatment, daily patient position was corrected using MVCT. Results. 4D-PTVs were smaller than STD-PTVs with a volume reduction equal to 37%. 3DCRT plans on 4D-PTV showed a significant sparing of most OARs, the use of IMRT allowed a further significant dose reduction. In the Phase I study the PTV subvolume received up to 55 Gy with modest increase in dose to OARs. Conclusions. The 4DCT procedure decreases the overlap between PTV and OARs. HT technique, compared with 3DCRT, allows efficient dose sparing in particular for the duodenum. The IMRT/IGRT approach allows a safe dose escalation to PTV subvolume.
Despite a number of new chemotherapeutic agents tested in combination with radiotherapy (RT), prognosis of pancreatic ductal adenocarcinoma (PDA) remains disappointing with a median survival ranging from 7.8 to 13.5 months and both local and distant control are lacking [Citation1–3].
Delivery of higher doses of standard fractionated RT or hypofractionation could provide a benefit in local control [Citation1,Citation3]. In both cases a more selective irradiation of tumor is mandatory to limit the risk of actinic toxicity. Previously reported data indicated that nodal recurrences were uncommon after “involved-field” RT and concurrent full-dose systemic therapy [Citation2], therefore a more recent trend has been to exclude the draining lymphatic from the clinical target volume (CTV) [Citation2,Citation4–6].
The accurate definition of abdominal diseases is problematic due to organ motion mainly related to the respiratory process. Geometric margins added to CTV in order to include motion and set-up uncertainties are usually derived from clinical experience using a population-based approach. In four-dimensional CT (4DCT) a reconstructed 3D data set is created for each phase and intrafractional organ mobility can thus be accurately quantified [Citation7]. Our procedure of contrast enhanced 4D-CT has been described in a recent paper [Citation8]; in a single CT scan session, high quality contrast enhanced CT images and motion information were obtained. This protocol yielded images allowing good differentiation between neoplastic tissues, normal tissues and regional vessels, and proved useful in defining accurate, personalized target volume.
In locally advanced PDA, a few sensitive organs at risk (OARs) are close to or overlapped with the planning target volume (PTV). Thus, recent innovations in the RT delivery, such as intensity modulated radiation therapy (IMRT), may guarantee a dose escalation with concomitant better sparing of OARs compared with three dimensional conformal radiation radiotherapy (3DCRT); with IMRT the toxicity profile is encouraging and local control is not compromised [Citation9–11]. Helical Tomotherapy (HT) is a dynamic IMRT unit; the gantry rotates as the couch advances slowly through the ring. Few studies have investigated the potential of HT to deliver acceptable dose distribution for complex lung, prostate and head and neck cases [Citation12–14], and to our knowledge no reports are available regarding the use of HT in pancreatic cancer.
HT utilizes an integrated on-line megavoltage CT (MVCT) unit that permits the verification of patient positioning; by means of daily MVCT the assessment of internal organ motion is feasible, and has proven useful in developing image guided radiotherapy (IGRT) [Citation15,Citation16].
Given the availability in our department of 4DCT and IMRT/IGRT technologies, a Phase I study was defined to treat locally advanced PDA using a (moderate) hypofractionated irradiation regime with concomitant chemotherapy.
This study investigated the following main points related to the reduction in the irradiated target volume and the feasibility of escalating the dose to tumor (sub)volume:
a) the impact of the 4D-CT imaging technique on RT planning and dosimetric comparison between 3DCRT and IMRT. “Conventional” and 4D defined PTV (STD-PTV and 4D-PTV) were created and two plans defined using 3DCRT technique; the plans were compared in terms of the sparing of healthy organs. In order to investigate the possibility of additional improvements in dosimetry using the IMRT approach, just for the 4D-PTV, HT plans were created for the same prescribed dose (60 Gy).
b) Definition of a moderately hypofractionated Phase I study. The contrast enhanced 4D-CT procedure allows optimized target volume definition. Within the 4D-PTV, a sub-volume was defined around the region of vessel infiltration. The possibility has been advanced that unresectable pancreatic tumors can be converted to resectable by means of chemoradiation and this option could be more effective in PTs with limited vascular involvement [Citation1,Citation6]. For PTs candidate to Tomotherapy treatment, we investigated the possibility of dose escalation in this area with an integrated boost approach. The accuracy of on-line positioning correction was evaluated by means of daily MVCT.
Material and methods
From March 2006 to May 2008, 15 patients with stage III PDA were considered in this study; all PTs underwent a contrast-enhanced 4DCT scan.
Twelve of 15 PTs were enrolled in a Phase I study based on hypofractionated RT and concomitant with continuous 5-fluorouracil infusion (250 mg/m2/day) or oral capecitabine (850 mg/m2; BID). The prescribed dose was 44.25 in 15 fractions to the PTV defined on 4DCT images; a second PTV was defined including infiltrated vessels and treated with a concomitant boost technique (50–55 Gy). PTs were irradiated using HT and, prior to each delivery session, correct PT positioning was verified by a MVCT. At our institution daily correction of patient set-up by MVCT using HT has been routinely performed since December 2004. The uncertainty of the automatic bone matching was investigated on appropriate phantoms and was found to be highly efficient and accurate.
4DCT data acquisition
Each patient underwent a WB PET/CT and 4DCT study performed using an integrated PET/CT system (D-STE, GE Medical System, Milwaukee, USA) equipped with a 16 slice MDCT scanner (Hi-Speed, GE Medical System, Milwaukee, USA). The patients were scanned in supine position within a personal body-fix pillow (BlueBAG BodyFix, Insight Technologies, Nevada, USA). During the scans, patients breathed freely, with the advice to maintain a breathing cycle as regular as possible. The details of the WB CT/PET and 4DCT study protocol have been indicated in a previous paper [Citation8]. For this study, four phases were used: maximum inspiration, maximum expiration and two intermediate phases, one on the descending part of the breathing cycle and one on the ascending. All CT images were imported into the treatment planning system (TPS, Eclipse, Varian Medical System, Palo Alto, CA, USA).
Delineation of targets and organs
Two different PTVs were generated: one obtained using the information provided by the 4DCT study, and thus called 4D-PTV, and one contoured using a standard procedure, called STD-PTV. The CTV consisted of the pancreatic or suspected tumor and regional lymph nodes considered radiologically involved and/or PET positive [Citation2,Citation4,Citation5]; no prophylactic nodal irradiation was planned. The 4D-PTV was obtained using the following contouring procedure: a CTV was manually contoured on each respiratory phase, resulting in four different CTVs; a 4D-ITV (“Internal Target Volume”) was created by the convolution of these four contours, using the Eclipse TPS, and then expanded by a margin of 5/5/7 mm to obtain a 4D-PTV. The standard PTV creation entailed the expansion of an intermediate phase CTV by 10/10/15 mm into the STD-PTV to account for mobility and set-up; the use of an intermediate phase reduces the contribution to the positioning error related to periodic motion of the tumor.
For the 12 PTs included in the Phase I study, a second 4D-PTV (4D-PTV-boost) was defined following the same procedure but considering only the area of tumor 1 cm around the infiltrated vessels. In order to avoid inter-observer variability in tumor delineation, all contours were drawn by the same physician.
On the simulation CT the following OARs were defined: liver, kidneys, stomach, duodenum and spinal cord.
Planning comparison: 3D vs 4D target definition; 3DCRT vs IMRT
3DCRT plans. Two 3DCRT plans were generated: one using the 4D-PTV as target volume and the other using the standard PTV.
The 3DCRT plans were created using Varian Eclipse TPS, 18 MV beam and MLC; the dose grid was 0.5 cm in the axial plane and 0.33 cm along the superinferior axis. Beam configuration was designed on the basis of our clinical experience to ensure acceptable PTV coverage and adequate protection of normal tissues; beam's eye view tools were used to select 3–5 beams with a 1-cm margin around PTV and the plans were optimized by means of beam energy, wedges and various beam weights. The 18 MV beam was used in 14 cases, a four field technique in 12 cases, a beam modifier (wedge) in three cases. Noncoplanar fields were not used. All dose calculations were performed using the pencil-beam algorithm with modified Batho heterogeneity correction.
Plans were developed with the primary aim of achieving good target coverage; the prescription was 60 Gy (30 fractions; the biological equivalent dose (BED) was 72 Gy when α/β= 10 Gy is assumed); in all cases the fraction of PTV receiving ≥ 95% of the prescribed dose was maintained ≥ 95%, and Dmax ≤ 110%. The maximal dose delivered to the spinal cord was maintained < 45 Gy.
IMRT plans. For each patient one IMRT treatment plan on the 4D-PTV was generated using HT inverse planning software based on superposition/convolution dose calculation.
For all plans, the “normal” dose grid setting was used (0.47 cm in the axial plane and 0.33 cm along the craniocaudal direction); a 2.5 cm jaw width was used; the mean pitch value was 0.287 and the modulation factor < 3. Plans were run with the goal of meeting both target volume and normal tissue constraints collected in . Plans were developed with the primary aim of achieving good target coverage; the prescription was 60 Gy; the fraction of PTV receiving ≥ 95% of the prescribed dose was maintained ≥ 95% with V105% < 5%. During iterative optimization processes, while maintaining tumor coverage as the highest priority, the volume of irradiated normal tissue was reduced to as great a degree as possible (i.e. to minimize overall DVH), even below the constraints indicated in .
Table I. Constraints used during IMRT plan optimization.
Planning evaluation. To compare plans, the DVHs of the PTV, duodenum, stomach, liver, kidney and spinal cord were calculated. Treatment plans were analyzed with regard to adequacy of target coverage and ability to conform to normal tissue dose limits. To assess the plan quality with respect to the tumor dose, mean dose (Dmean), dose distribution standard deviation (STD) and the percentage volume receiving more than 95% (V95%) and 105% (V105%) of prescribed dose were evaluated. To assess the implications of kidney and normal liver parenchyma irradiation, the mean dose was considered; regarding duodenum and stomach, both Dmax and Dmean were considered, and a comparison was made of DVHs at low and medium-high doses. For the other healthy tissues, a visual inspection of dose distribution on each CT slice was performed to guarantee an acceptable sparing.
Phase I study
Dosimetric analysis. Twelve PTs affected by stage III PDA were considered. Two PTVs were defined based on 4DCT images as previously described; the first included the entire tumor (4D-PTV) and the second only the disease within 1 cm around the infiltrated vessels (4D-PTV-boost). A dose equal to 44.25 Gy in 15 fractions was prescribed to the 4D-PTV, the biological equivalent dose (BED) was 57.30 when α/β= 10 Gy is assumed. On the 4D-PTV-boost the prescribed dose in 15 fractions was 50 Gy (in 6 PTs), 52 Gy (in 3 PTs) and 55 Gy (in 3 PTs) (BED = 66.67–75.17 Gy). During planning optimization the same criteria as those adopted in the planning comparison section were followed. Tumor coverage had the highest priority and the OAR constraints (summarized in ) were corrected for fractionation (with α/β=3 Gy for all OARs but spinal cord with α/β=2 Gy). To estimate the normal tissue complication probability (NTCP), the Lyman model with the Kutcher–Burman histogram reduction scheme was used [Citation17]. The model is based on three parameters including the dose giving 50% complication probability (TD50), volume dependence (n) and the steepness value of the dose–response curve (m). For the two considered OARs, the model parameters obtained using Emami et al. [Citation17,Citation18] clinical data were used; for the stomach TD50 = 65 Gy, m = 0.14 and n = 0.15 (“End point” = Ulceration/Perforation), for the duodenum TD50 = 55 Gy, m=0.16 and n = 015 (“Organ” = Small Intestine; “End point” = Obstruction/Perforation). NTCP calculations were performed using BIOPLAN [Citation19] software tool; each bin of the DVH was corrected for fractionation using the linear quadratic formula resulting in the normalized dose distribution.
Daily on-line correction through MVCT. Daily MVCT scans can be registered with planning KVCT images and positioning adjustments may be assessed. A two step matching strategy was followed: first, a fully automatic registration based on bony anatomy was performed. Subsequently, the matching was adjusted by a physician through direct visualization. As healthy and affected pancreatic tissue is not well visible on MVCT images, our procedure uses particular care in the overlapping of the 12th costo-vertebral joints and of inter vertebral spaces. Aorta, vena cava, and the origins of their main efferent or afferent vessels are further excellent reference points that should always be checked and overlapped. The final correction was automatically applied and the patient was then treated; the mean delivery time was around 400 s.
During the treatment of 12 PTs included in the Phase I study, 180 MVCT were acquired and the values of the shifts after bone matching were registered for the three main directions (left-right: LR; posterior-anterior: PA; cranial-caudal: CC); average shift (mi) and related standard deviation SD (σi) were calculated for each patient and for each direction. Differences between automatic matching and final accepted positioning were reported in terms of the global mean among mi (MΔ), the mean value of σi (σΔ) and the SD among mi (ΣΔ).
Statistical analysis
The various dosimetric parameters were analyzed by Statistica software (Release 4, Statsoft Inc). Volumes and dosimetric parameters were compared by the Wilcoxon matched pairs test. Differences were reported to be statistically significant at p ≤ 0.05.
Results
Results can be distinguished in:
a geometric evaluation of the target movements obtained from the 4DCT study of 15 PTs, and a volumetric comparison between the two different target definitions (4D-PTV vs STD-PTV).
a dosimetric evaluation of doses delivered to OARs (stomach and duodenum in particular) with respect to the two PTV definitions and to the two irradiation techniques (3DCRT vs IMRT) for a prescribed dose equal to 60 Gy.
the summary of plan results and set-up corrections through MVCT of 12 PTs included in the hypofractionated and dose escalated Phase I study.
Geometric evaluations
The intrafraction motion of pancreatic tumor was evaluated considering the center of mass in the two extreme phases (i.e. end of inspiration/expiration). As expected, the tumor moved mainly along the superior-inferior axis, and the mean displacement was equal to −0.5 ± 0.3 cm. Considering displacement within the space (3D vector), the mean value was 0.6 ± 0.3 cm; in one of 15 PTs the vector was > 1.0 cm.
The mean PTV volume is 295.3 ± 132.9 cm3 for the conventional procedure and 187.9 ± 93.1 cm3 for the 4D approach with a mean percentage reduction of approximately 37% (p<0.006); as an example, the two PTVs are indicated in for patient N 15.
Figure 1. Comparison of PTV-STD (259.5 cm3; azure color) and 4D-PTV (185.0 cm3; red color) for patient number 15 on axial (A) and sagittal (B) views.
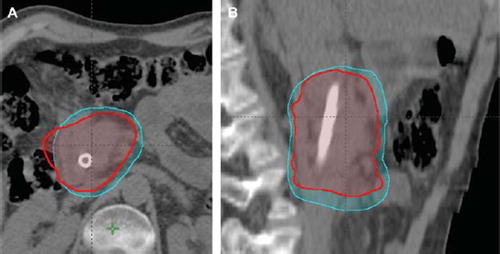
The amount of stomach included in the 4D-PTV and STD-PTV is 6.9 ± 6.8 cm3 and 17.6 ± 13.4 cm3 respectively, whereas for duodenum the overlap is 9.0 ± 9.7 cm3 and 15.9 ± 14.4 cm3 respectively; all differences were statistically significant (p<0.002).
Plan comparison
3DCRT: STD-PTV vs 4D-PTV. In the 3DCRT approach target coverage and target heterogeneity was very similar for the two defined PTVs (conventional and 4D based). The use of a smaller PTV guaranteed better OAR sparing and the differences were statistically significant for almost all the dosimetric indices detailed in . The mean percentage reduction in D-mean was approximately 15–23% for liver, duodenum, stomach and right kidney. The absolute volume receiving > 20–55 Gy decreased by 5–8 cm3 and 15–23 cm3 for duodenum and stomach respectively.
Table II. Helical Tomotherapy (HT) and 3D conformal radiotherapy (3DCRT) plans: Summary of dose statistics. Data presented as the mean, with the standard deviation in parentheses.
3DCRT vs HT. 4D-PTV coverage and dose heterogeneity were very similar for 3DCRT and HT. For duodenum, plans with HT produced lower Dmean, V20–V55 compared with 3DCRT and the differences reached statistical significance; the absolute volume in the high dose region (>50 Gy) decreased by 12 cm3 with HT. When considering the stomach, HT significantly reduced the dose in the 20–55 Gy range. The D-mean received by the liver was slightly higher with HT, but a significant reduction in V30 (around 9%) was obtained with HT. Similar behavior was demonstrated by the right kidney dosimetric data: HT resulted in higher Dmean and V20 values but not V30 (). The left kidney received low doses with both techniques.
Table III. Phase I study, dosimetric data (12 PTs). Summary of dose statistics for PTV, liver, spinal cord, duodenum, stomach, right kidney. Data presented as the mean, with the standard deviation in parentheses.
Phase I study: planning and MVCT data
The 4D-PTV-boost volume around infiltrated vessels had a mean volume equal to 87.2 ± 34.1 cm3, that is around 45% of total 4D-PTV. Both stomach and duodenum overlapped with 4D-PTV-boost around 2.0 cm3, with maximum value < 8 cm3.
The two 4D-PTV volumes and dose distribution are indicated in for patient N15.
Figure 2. Dose distribution obtained with HT; prescribed dose equal to 44.25 and 52 Gy for 4D-PTV (red color) and 4D-PTV-boost (98.6 cm3; azure color) respectively; patient number 15, axial (A) and sagittal (B) views. Isodose levels are indicated in Gy.
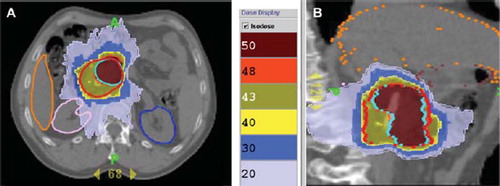
Although nearly 50% of 4D-PTV received a dose up to 25% higher than the prescribed 44.25 Gy, the mean dose to OARs () is similar to that reported in after dose scaling (44.25 instead of 60 Gy). Mean V45 was 5.2% (4.8 cm3) and 1.9% (4.9 cm3) for duodenum and stomach respectively; the dose of 45 Gy delivered in 15 fractions is equivalent to 48.75 Gy and 53.1 Gy with α/β equal to 10 Gy (“early effects”) and 3 Gy (“late effects”) respectively.
The mean NTCP values for late toxicities were < 1% for stomach and 10.7 ± 8.3% for duodenum; similar NTCP data were obtained with HT plans for a prescribed dose of 60 Gy (in 30 fractions) to 4D-PTV, < 1% and 12.8 ± 4.8% for stomach and duodenum respectively.
With respect to the evaluation of on-line positioning correction by means of MVCT, the deviation between bone matching and the final direct visualization was slight, with mean deviation around zero (MΔ), σΔ≤1.7 mm and ΣΔ≤1 mm; fewer than 5% of daily corrections of patient set-up by means of MVCT required a 3D shift ≥ 7 mm after bone matching ().
Table IV. MVCT data analysis. Percentage of shift and summary of systematic and random errors (relative to bony anatomy).
Discussion
Despite some progress has been achieved in the management of locally advanced unresectable pancreatic cancer, the final outcome remains disappointing in most cases [Citation1–3]. The administration of an higher radiotherapy dose to the tumor-vascular interface could convert patients to resectability with expected improved clinical outcome [Citation1,Citation6].
The current study investigated the possibility of reducing irradiated volumes with a 4DCT scan, and of escalating the radiation dose by boosting the PTV region close to the infiltrated vessels by means of IMRT/IGRT technology. The definition of this PTV subvolume derives from the assumption that the sterilization of this infiltrated region could convert PTs to surgical candidates with a possible survival benefit as surgery offers the only potential cure for pancreatic carcinoma [Citation3].
Four dimensional CT technologies now represent a primary imaging technique for the evaluation of tissue mobility, and several studies have evaluated abdominal organ movement [Citation7,Citation20–22]. Kim et al. [Citation20] reported (for 9 PTs with CT scan performed with neither specific instruction in breathing nor immobilization device) a mean 3D intrafractional motion of the pancreas of around 13 ± 7.5 mm. In the current study, the evaluated intra-fraction 3D mobility of pancreatic tumor (6.0 ± 2.9 mm) was similar to the data included in the recent paper by Van der Geld et al. [Citation21] where 3D mobility was 8.7 ± 2.8 mm, with PTs immobilized in the supine position, and 4DCT procedure performed during quite breathing.
4DCT permits the individualized assessment of tumor mobility, with the generated volume representing the entire volume occupied by the CTV during free respiration. With conventional 3DCRT, the treatment plan is designed using a single CT scan obtained during free breathing with margins derived from population-based studies that determine excessive irradiation of the normal tissue. Xi et al. [Citation22] compared 4D-PTV vs STD-PTV in case of 10 PTs affected by hepatocellular carcinoma; the margins used to defined conventional PTV, taking into account both mobility and set-up errors, were 0.8 cm in the left-right and anterior-posterior directions, but 1.0–2.5 cm in the superior-inferior direction as mobility in this direction was determined for each PT by means of fluoroscopic projection; with the 4DCT approach the PTV mean decease was around 78.6 cm3 (19%). In our study, conventional margins equal to 10/10/15 mm were used for all PTs; PTV volume decreased by 107.4 cm3 (37.4%) with 4DCT, with the related reduction in the overlap with PTV volume equal to 10.7 cm3 and 6.9 cm3 for stomach and duodenum respectively; the amount of stomach or duodenum included in the PTV had a great variability among patients related to the tumor position and healthy tissue shape.
Milano and coworkers [Citation11] published a dosimetric comparison of 3D conformal RT and IMRT in six PTs affected by pancreatic and cholangiocarcinoma malignancies with CTV encompassing the draining lymphatics in all PTs: IMRT reduced the mean doses in all critical structures with a statistically significant reduction only for the right kidney and small bowel; the differences were greater when considering volumes receiving more than the threshold doses (30 Gy for liver and small bowel, 22 Gy for right kidney). The inclusion of draining lymphatics in the target volume determined a higher radiation level to all OARs compared with our data with differences in the mean dose in the range 3–9 Gy.
Only a few studies have reported the use of 4DCT data for the definition of RT plans in pancreatic carcinoma; the study by Van der Geld et al. compared 3D conformal RT (15 MV beam energy) and IMRT (6 MV beam energy) plans defined for the same 4D-PTV [Citation22], and found significant OAR sparing with IMRT. Their data cannot be easily compared with our results due to the larger margin used (1.5 cm isotropic), the lower prescribed dose (50.4 Gy) and the same four-field beam configuration adopted for both 3D conformal RT and IMRT planning: similar reduction in the mean stomach dose (around 10–15%), differences in the sparing of right kidney whose mean dose was reduced by 45% and 25% with HT (our data) and IMRT respectively.
4DCT has enabled clinicians to determine the intrafractional mobility of pancreatic tumors during a single procedure; in order to minimize geographic misses, both set-up variations and interfractional motion must be considered. The daily use of MVCT for patient positioning can reduce both set-up errors and intra-fraction internal organ variation. Li et al. [Citation16] have reported intrafractional position and anatomic changes assessed by means of daily MVCT. The pancreas (and not lymphatic nodes) was contoured on the planning CT scan and on daily MVCT images, and interfractional anatomic changes were evaluated by means of the shift of the center of mass from the registered planning CT; the interfractional variations for the pancreas were significant, with movement in certain cases up to ± 20 mm.
The on-line correction strategy for pancreatic PTs treated with Tomotherapy reduces set-up errors and improves inter-fraction target localization, adjusting the final match by checking the structures close to the tumor; after bone-based matching of MVCT with simulation CT, the patient position is further modified with 3D shift > 7 m in nearly 5% of cases.
In our procedure the 4D-PTV comes from an expansion of the internal target volume (ITV, i.e. the overlapping of CTVs delineated on different respiratory phases) by a margin of 5/5/7 mm. Quite different approaches are followed in other radiotherapy departments, the PTV is defined as GTV plus a 2–3 cm margin. However in a few (institutional and multi-institutional) clinical studies, the GTV consisted of primary tumor plus enlarged adjacent lymph nodes (defined on the contrast-enhanced spiral CT scan) and the PTV was defined as the GTV plus a 1-cm margin in all directions without any evaluation of tumor movement and the daily position was verified by means of portal films or EPID [Citation2,Citation23,Citation24].
Our MVCT on-line correction strategy is able to correct for the set-up errors and to “reduce” the inter fraction variation of baseline position; the evaluation of the residual errors, mainly related to intrafractional target mobility and localization accuracy of tumor position on MVCT, will be a matter of future research.
Extracranial stereotactic radiotherapy has been proposed as an indication in inoperable pancreatic cancer, but the results are quite controversial. In our Phase I study, the prescribed dose at 4D-PTV is 44.25 Gy in 15 fractions in three weeks (BED=57.30 Gy), while 4D-PTV-boost receives 50–55 Gy (BED=66.7–75.2Gy); a dose of 60 Gy in standard 2 Gy fractions determines a BED=72 Gy without considering the role of overall treatment time. Several studies have suggested that, in lung and head-neck diseases, local control can be improved by shortening overall treatment time; in case of lung cancer the dose lost per day due to repopulation is estimated around 0.4 Gy [Citation25,Citation26] Considering OARs, dosimetric and biological indices are similar for Phase I data and standard treatment of 60 Gy to the 4D-PTV; however, the overall shortening of treatment time with a hypofractionated approach could result in an increase of acute toxicities.
A radiation (hypofractionated) dose escalation study in PTs with unresectable pancreatic cancer, using the previously described IMRT/IGRT methodology, is currently underway at our institution; toxicity and clinical outcome will be described in a further publication after an adequate follow-up period.
Conclusions
Contrast enhanced 4DCT permits patient-specific target volume definition, thus avoiding the use of standard margins that determine unnecessary normal tissue irradiation. For locally advanced pancreatic cancer, HT results in a better sparing of most OARs (mainly stomach and duodenum) compared with 3DCRT. Using IMRT/IGRT procedures, simultaneous additional dose escalation of target subvolume is technically feasible.
Declaration of interest: The authors report no conflicts of interest. The authors alone are responsible for the content and writing of the paper.
References
- Crane CH, Varadhachary G, Pisters PW, Evans DB, Wolff RA. Future chemoradiation strategies in pancreatic cancer. Semin Oncol 2007;34:335–46.
- Murphy JD, Adusumilli S, Griffith KA, Ray ME, Zalupski MM, Lawrence TS, . Full dose gemcitabine and concurrent radiotherapy for unresectable pancreatic cancer. Int J Radiat Oncol Biol Phys 2007:68:801–8.
- Russo S, Butler J, Ove R, Blackstock AW. Locally advanced pancreatic cancer: A review. Semin Oncol 2007;34:327–34.
- Huguet F, André T, Hammel P, Artru P, Balosso J, Selle F, . Impact of chemoradiotherapy after disease control with chemotherapy in locally advanced pancreatic adenocarcinoma in GERCOR Phase II and III Studies. J Clin Oncol 2007;20:326–31.
- Reni M, Cordio S, Milandri C, Passoni P, Bonetto E, Oliani C, . Gemcitabine versus cisplatin, epirubicin, fluorouracil, and gemcitabine in advanced pancreatic cancer: A randomized controlled multicentre phase III trial. Lancet Oncol 2005;6:369–76.
- Spalding AC, Jee KW, Vineberg JK, Jablonowski M, Fraass BA, Pan CC, . Potential for dose-escalation and reduction of risk in pancreatic cancer using IMRT optimization with lexicographic ordering and gEUD-based cost function. Med Phys 2007;34:521–9.
- Keall PJ, Mageras GS, Balter JM, Emery RS, Forster KM, Jiang SB, . The management of respiratory motion in radiation oncology report of AAPM Task Group 76. Med Phys 2006;33:3874–900.
- Mancosu P, Bettinardi V, Passoni P, Gusmini S, Cappio S, Gilardi MC, . Contrast enhanced 4D-CT imaging for target volume definition in pancreatic ductal adenocarcinoma. Radiother Oncol 2008;87:339–42.
- Ben-Josef E, Shields AF, Vaishampayan U, Vaitkevicius V, El-Rayes BF, McDermott P, . Intensity-modulated radiotherapy (IMRT) and concurrent capecitabine for pancreatic cancer. Int J Radiat Oncol Biol Phys 2004;59:454–9.
- Brown MW, Ning H, Arora B, Albert PS, Poggi M, Caphausen K, . A dosimetric analysis of dose escalation using two intensity-modulated radiation therapy techniques in locally advanced pancreatic carcinoma. Int J Radiat Oncol Biol Phys 2006;65:274–83.
- Milano MT, Chmura SJ, Garofalo MC, Rash C, Roeske JC, Connell PP, . Intensity-modulated radiotherapy in treatment of pancreatic and bile duct malignancies: Toxicity and clinical outcome. Int J Radiat Oncol Biol Phys 2004;59:445–53.
- Cattaneo GM, Dell'Oca I, Broggi S, Fiorino C, Perna L, Pasetti M, . Treatment planning comparison between conformal radiotherapy and helical tomotherapy in case of locally advanced stage NSCLC. Radiother Oncol 2008;88:310–8.
- Iori M, Cattaneo GM, Cagni E, Fiorino C, Borasi G, Calandrino R, . Dose-volume and biological-model based comparison between helical tomotherapy and (inverse-planned) IMAT for prostate tumours. Radiother Oncol 2008;88:34–45.
- Widesott L, Pierelli A, Fiorino C, Dell'Oca I, Broggi S, Cattaneo GM, . Intensity modulated proton therapy vs helicoidal tomotherapy in nasopharynx cancer. Planning comparison and NTCP evaluation. Int J Radiat Oncol Biol Phys 2008;72:589–96.
- Fiorino C, Di Muzio N, Broggi S, Cozzarini C, Maggiulli E, Alongi F, . Evidence of limited motion of the prostate by carefully empting the rectum as assessed by daily MVCT image guidance with Helical Tomotherapy. Int J Radiat Oncol Biol Phys 2008;71:611–7.
- Li AX, Qi XS, Pitterle M, Kalakota K, Mueller K, Erickson Ba, . Interfractional variations in patients setup and anatomic change assessed by daily computed tomography. Int J Radiat Oncol Biol Phys 2007;68:581–91.
- Burman C, Kutcher GJ, Emami B, Goitein M. Fitting of normal tissue tolerance data to an analytic function. Int J Radiat Oncol Biol Phys 1991;21:123–35.
- Emami B, Lyman J, Brown A, Coia L, Goitein M, Munzenrider JE, . Tolerance of normal tissue to therapeutic irradiation. Int J Radiat Oncol Biol Phys 1991;21:109–22.
- Sanchez-Nieto B, Nahum AE. BIOPLAN: Software for the biological evaluation of radiotherapy treatment plans. Med Dosim 2000;25:71–6.
- Kim YS, Park SH, Ahn SD, Lee JE, Choi EK, Lee SW, . Differences in abdominal organ movement between supine and prone positions measured using four-dimensional computed tomography. Radiother Oncol 2007;85:424–8.
- Van der Geld YG, van Triest B, Verbakel WF, Verbakel WFAR, van Sornsen de Koste JR, Senan S, . Evaluation of four-dimensional computed tomography-based-intensity-modulated and respiratory-gated radiotherapy techniques for pancreatic carcinoma. Int J Radiat Oncol Biol Phys 2008;72:1215–20.
- Xi M, Liu MZ, Deng XW, Zhang L, Huang XY, Liu H, . Defining internal target volume (ITV) for hepatocellular carcinoma using four-dimensional CT. Radiother Oncol 2007;84:272–8.
- Ceha HM, van Tienhoven G, Gouma DJ, Veenhof CHN, Schneider CJ, Rauws EAJ, . Feasibility and efficacy of high dose conformal radiotherapy for patients with locally advanced pancreatic carcinoma. Cancer 2000;89:222–9.
- Talamonti MS, Small W, Mulcahy MF, Wayne JD, Attaluri VA, Colletti LM, . A multi-institutional Phase II trial of preoperative full-dose gemcitabine and concurrent radiation for patients with potentially resectable pancreatic carcinoma. Ann Surg Oncol 2006;13:150–8.
- Abratt RP, Bogart JA, Hunter A. Hypofractionated irradiation for non-small cell lung cancer. Lung Cancer 2002;36:225–33.
- Fowler JF, Tomé WA, Fenwick JD, Mehtha PM. A challenge to traditional radiation oncology. Int J Radiat Oncol Biol Phys 2004;60:1241–56.