Abstract
Background and purpose. Cardiac disease and pulmonary complications are documented risk factors in tangential breast irradiation. Respiratory gating radiotherapy provides a possibility to substantially reduce cardiopulmonary doses. This CT planning study quantifies the reduction of radiation doses to the heart and lung, using deep inspiration breath-hold (DIBH). Patients and methods. Seventeen patients with early breast cancer, referred for adjuvant radiotherapy, were included. For each patient two CT scans were acquired; the first during free breathing (FB) and the second during DIBH. The scans were monitored by the Varian RPM™ respiratory gating system. Audio coaching and visual feedback (audio-visual guidance) were used. The treatment planning of the two CT studies was performed with conformal tangential fields, focusing on good coverage (V95>98%) of the planning target volume (PTV). Dose-volume histograms were calculated and compared. Doses to the heart, left anterior descending (LAD) coronary artery, ipsilateral lung and the contralateral breast were assessed. Results. Compared to FB, the DIBH-plans obtained lower cardiac and pulmonary doses, with equal coverage of PTV. The average mean heart dose was reduced from 3.7 to 1.7 Gy and the number of patients with >5% heart volume receiving 25 Gy or more was reduced from four to one of the 17 patients. With DIBH the heart was completely out of the beam portals for ten patients, with FB this could not be achieved for any of the 17 patients. The average mean dose to the LAD coronary artery was reduced from 18.1 to 6.4 Gy. The average ipsilateral lung volume receiving more than 20 Gy was reduced from 12.2 to 10.0%. Conclusion. Respiratory gating with DIBH, utilizing audio-visual guidance, reduces cardiac and pulmonary doses for tangentially treated left sided breast cancer patients without compromising the target coverage.
Radiotherapy following breast conserving surgery significantly reduces the risk of breast and axillary recurrence and results in long-term survival similar to that after mastectomy [Citation1,Citation2]. However, irradiating heart and ipsilateral lung tissue is often unavoidable. An increase in mortality from cardiac disease has been reported for left sided breast cancers, especially ten or more years after treatment [Citation3–5]. Pulmonary complications may also be induced by radiotherapy for these patients [Citation6]. In recent years respiratory gating has been introduced in radiotherapy as a possibility to reduce the cardiopulmonary doses.
During inspiration the distance between the breast and the heart is increased. Previously, research groups have presented convincing results using different respiratory gating systems and breathing techniques [Citation7–13]. By delivering the treatment during inspiration, the high dose region in the heart can be reduced, or avoided, and the relative volume of the ipsilateral lung exposed to irradiation decreased.
We have treated breast cancer patients in our department since 1999 with very low incidence of local recurrence [Citation14]. To further improve the quality of treatment, respiratory gating was implemented in clinical practice in 2006. With this technique, irradiation is performed during deep inspiration breath hold (DIBH). Utilizing audio coaching and visual feedback, referred to as audio-visual guidance, the reproducibility of the breathing amplitude is high [Citation15,Citation16], and hence the gating window can be kept narrow.
The purpose of this CT planning study was to quantify the reduction of cardiac and pulmonary doses for tangential left breast treatment with respiratory gating, utilizing DIBH with audio-visual guidance, compared to conventional free breathing (FB). For both techniques good and similar dose coverage of the planning target volume (PTV) was mandatory.
To our knowledge, this is the first CT planning study utilizing DIBH with audio-visual guidance for tangential breast treatment where constraints regarding PTV coverage are clearly defined.
Patients and methods
Patients
CT series of 17 patients who were referred for adjuvant radiotherapy after breast conserving surgery at Stavanger University Hospital between January and October 2006 were analyzed. The inclusion of patients was not consecutive, but based on logistics availability (staff and venues). Of these patients, 12 had left sided and five had right sided breast cancer, but for study purposes the left breast was defined as the target in all patients. The median age was 60 (range: 29–70) years, and the patients had to be able to hold their breath for 15–20 seconds. The study was approved by the regional ethical committee as a project for quality assurance in health care. The CT series were taken prior to the clinical implementation of the DIBH technique and except for one, the patients were not treated with respiratory gating.
Respiratory gating
The Varian RPM™ respiratory gating system, version 1.6, (Varian Medical Systems, Palo Alto, CA) was used for respiratory control. An infrared reflecting marker is placed on the patient, normally over the xiphoid process, and a video camera registers the anteroposterior motion of the marker, due to respiration. The CT scanner or accelerator is then controlled to acquire images or irradiate only in a preselected interval of the chest wall motion, i.e. the gating window (, ). In a clinical setting the position of the reflecting marker is drawn on the patient's skin to reproduce the position throughout the treatment course.
Figure 1. The patient was positioned in a standard breast immobilization device. The marker box was placed on the chest. With the RPM™-system the breathing position is visible for the patient as a yellow line on the binocular head mounted display. When the line is within the gating window (blue) the color turns green and the CT scan can be acquired or irradiation delivered on the accelerator.
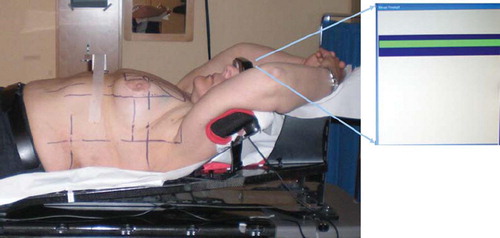
Figure 2. A DIBH breathing curve from CT scanning, with gaps of free breathing between each DIBH. The CT scan was acquired during one DIBH (dashed area) and the gating window (solid lines) was set to the mean amplitude ±1 mm.
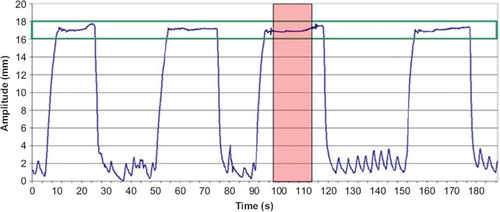
To establish a reproducible and stable breathing amplitude during DIBH, each patient underwent 30 minutes breathing training with the RPM™ system, prior to the CT study. The patients were placed in the treatment position with bilateral arm abduction above the head using an immobilization device (). In our department all breast cancer patients undergoing radiotherapy are placed in this position, independent of laterality and lymph node involvement.
Audio-visual guidance was used. The operator told the patient when to take a deep-breath and when to release the breath-hold, in addition to information about length of breath-hold, etc. The patient controlled the breathing amplitude by visual feedback on the binocular head mounted display. An example of a breathing curve is shown in . The gating window was individually set to the mean amplitude of the stable DIBH plateau ± 1 mm. The 2 mm width of the gating window was chosen to be of the same magnitude as the minimum mean breathing amplitude observed during FB CT scans of 14 breast cancer patients prior to this study.
CT scanning
For each patient two CT scans were acquired; the first during FB and the second during DIBH. Total patient time for each CT scan was approximately 30 minutes. The patients were positioned in a standard breast immobilization device (). The slice thickness was 3 mm and the image acquisition was in helical mode. For the DIBH scan, the respiration pattern of the patient was monitored by the table mounted RPM™ system, and the image acquisition was manually started when the breathing amplitude marker reached the gating window. The scanning time was approximately 20 seconds and most patients managed to complete the scan during one DIBH cycle. For those who did not, the CT had to be manually stopped when the marker left the gating window, and restarted once it re-entered.
Delineation of target and organs at risk
Remaining mammary glandular tissue was defined as clinical target volume (CTV) for whole breast radiotherapy after breast conserving surgery. The heart, LAD coronary artery, ipsilateral lung and the contralateral breast were considered organs at risk.
For consistency the delineation of CTV and the contralateral breast was performed by the same oncologist for all patients using the Eclipse treatment planning system, version 8.0 (Varian Medical Systems). The margin from CTV to PTV was 5 mm, except for superficial areas where PTV was never closer than 5 mm to the skin. The margins were the same for DIBH and FB. Due to the chosen 2 mm gating window the movement of the chest wall during the DIBH plateau was the same, or smaller, as compared to FB. Hence the margin to compensate for CTV motion did not have to be increased with the DIBH technique.
Heart, LAD coronary artery and lungs were delineated without margin by a radiation technologist. LAD coronary artery was delineated in the anterior interventricular groove down to the apex of the heart under supervision from a cardiologist. The delineation of the heart was verified by an oncologist.
Treatment planning
For each patient the treatment planning was performed by the same radiation technologist. The following restrictions were given:
Minimum 98% of the PTV should be covered by the 95% isodose line (V95% ≥ 98%).
The mean dose to PTV should be close to 100% of the prescribed dose and not above 101.5%.
Hotspots should not exceed 110% and preferably not 107%.
The dose to organs at risk should be kept as low as possible, without compromising the PTV dose, i.e. the criteria of PTV coverage should be fulfilled, even if the clinical limits for doses to organs at risks were exceeded.
For each patient only minor differences between the two plans, FB and DIBH, with respect to target dose coverage, dose conformity, maximum dose, beam energy and geometry, were accepted.
The prescription dose was 50 Gy in 2 Gy fractions. Two opposing 6 MV tangential conformal fields with multileaf collimator and wedges were used. For a proportion of the patients low weighted 15 MV fields, with the same geometry as the 6 MV fields, were added. The calculation algorithm was Eclipse pencil beam (PB) described by Storchi et al. [Citation17] with the modified Batho inhomogeneity correction [Citation18].
Statistical analysis
Dose-volume histograms (DVHs) were calculated and compared for the FB as well as DIBH plans. Doses to CTV, PTV and organs at risk were assessed. Volume size, mean and maximum doses were obtained from the DVH statistics. The relative volume Vx, irradiated to a minimum dose x (in Gy or %), e.g. V25 for the heart, V20 for the lung and V95% for PTV, were registered from the DVH graph. The maximum heart distance (MHD) and central lung distance (CLD) were measured in beams eye view.
Paired Wilcoxon test was used for statistical analysis of the differences with computer software SPSS version 15.0. Data were considered statistically significant for p < 0.05.
Results
All 17 patients complied well and were able to follow the audio-visual guidance.
The mean amplitude of the reflective marker movement during CT scanning, as measured with the RPM-system, was 3.0 mm during FB, with a range of 1.6–5 mm, and 18 mm (range 14.6–27 mm) during DIBH. With audio-visual guidance, reproducibility of the DIBH amplitude within the 2 mm gating window was feasible.
Volumes
shows the mean volume (±SD) for all delineated volumes in FB and DIBH, respectively. The mean lung volume increased to almost double size during DIBH, whereas the mean heart volume decreased 8.8% during DIBH (p =0.003). CTV and PTV volumes were somewhat smaller in the DIBH studies (mean relative volume 97.8% and 98.1%, p=0.013 and p = 0.017 respectively) as compared to FB. For the other delineated volumes no significant difference in size was found.
Table I. Mean volume, in cm3, for target volumes and organs at risk during free breathing (FB) and deep inspiration breath-hold (DIBH) for all 17 patients.
Planning
All plans fulfilled the criterion of dose coverage to the target as described in methods. A summary of the treatment planning data is given in . For both techniques the average mean PTV dose was 100.5%. The mean relative V95% for PTV was 99.1% (range 98.1–99.7) and 98.9% (range 98.2–99.7) for FB and DIBH, respectively (no significant difference). For CTV, the mean relative V95% was 99.6% (98.9–100.0) and 99.5% (98.7–99.9) for FB and DIBH, respectively.
Table II. Summary of treatment planning data for PTV and organs at risk, for the 17 breast cancer patients included in this study, with free breathing (FB) and deep inspiration breath-hold (DIBH). The prescription dose was 50 Gy in 2 Gy fractions.
There were only minor differences between the FB and DIBH plan for each patient in terms of conformity and beam geometry. However, the mean percentage weight of the 15 MV fields to the total field weight was slightly different. For ten patients 15 MV was used with a mean weight of 19% for DIBH and 14% for FB (p=0.007).
In , beam's eye views of the medial tangential field for a typical patient are shown for FB and DIBH, respectively. Due to the increased lung volume in DIBH, the breast is moved cranioventrally, and the heart caudally, resulting in less heart volume inside the beam portal, and in most cases no heart volume at all. The distance from PTV to LAD coronary artery is also increased, but due to its anatomical position, the LAD coronary artery is often within the high dose region. The corresponding DVHs are shown in , and demonstrate a dose reduction to the heart, the LAD coronary artery, and the ipsilateral lung.
Figure 3. Beam's eye views of the medial tangential field during free breathing (left) and deep inspiration breath-hold (right) for a typical patient. During inspiration the lung volume (light yellow) is increased, the breast (red) is moved cranioventrally and the heart (brown) caudally. In the shown case, the heart and the LAD coronary artery (blue) were not included in the beam portal (yellow lines) during DIBH.
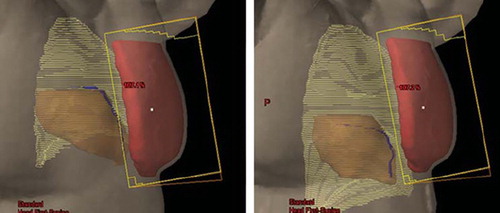
Cardiac doses
All treatment plans based on FB technique included heart tissue within the beam portals (). With the DIBH technique the heart was outside the beam portals for ten of the 17 patients (58.8%). The average MHD decreased from 1.3 cm (FB) to 0.3 cm (DIBH) and the average mean heart dose was reduced, from 3.7 Gy (range 3.2–20.1 Gy) to 1.7 Gy (range 2.2–10.1 Gy). Both of these differences were significant (p<0.001). The median relative heart volume irradiated to 25 Gy or more (V25) was 2.0% and 0.0% for FB and DIBH, respectively (p < 0.001). In four patients (23.5%), V25 was larger than 5% when using FB technique, compared to only one patient (5.9%) when using DIBH. Maximum V25 was 16.8% for FB and 6.3% for DIBH.
For the LAD coronary artery there was a significant mean dose reduction (p < 0.001) from 18.1 Gy (range 2.4–43.0 Gy) to 6.4 Gy (range 1.7–31.0 Gy) and median V25 was reduced from 28.8% with FB to 0.0% (p < 0.001) with DIBH. The maximum dose was reduced (p < 0.001) from 38.7 Gy (range 4.3–49.4 Gy) to 16.7 Gy (range 2.1–47.8 Gy).
Pulmonary doses
Average ipsilateral lung volume receiving 20 Gy or more, V20, was reduced (p = 0.002) from 12.2% (range 7.7–18.3%) using FB to 10.0% (range 6.1–13.7%) using DIBH (). Similarly, the average mean dose was reduced (p = 0.002) with DIBH, from 6.9 Gy (range 4.8–10.2 Gy) to 5.9 Gy (range 4.0–7.9 Gy). The mean CLD of the medial field increased from 2.1 cm with FB to 2.2 cm with DIBH (p = 0.011).
Contralateral breast doses
With the DIBH technique there was a small, non-significant increase in the average contralateral breast volume receiving 2 Gy, V2Gy, from 3.4% (FB) to 3.7% (DIBH). An example dose distribution is shown in .
Discussion
This study confirms the ability of the respiratory gating technique utilizing DIBH to reduce the radiation dose to the heart and ipsilateral lung tissue for tangential whole breast irradiation, as stated in earlier studies [Citation7–13]. However, there are two main differences in our study as compared to the previous ones. The demonstrated doses to organs at risk were obtained with strict constraints on PTV coverage and the gating window was kept very narrow with the use of audio-visual guidance.
Earlier studies of improved reproducibility of the breathing amplitude with audio-visual guidance [Citation15,Citation16] as compared to audio coaching were confirmed. Visual feedback enables a narrower gating window which reduces intrafractional motion of the target. We used a 2mm gating window, while in other studies, with audio coaching only, a 4 mm gating window has been used [Citation9–11,Citation13]. To our knowledge there is no study of respiratory gating for breast cancer patients utilizing visual guidance only. Audio coaching enables direct communication with the patient, has a calming effect and facilitates an optimum pace of the DIBHs with regard to beam-readiness for the treatment fields. This results in a high accuracy and high treatment time efficiency as the pace is kept by the operator by audio feedback and the DIBH amplitude by the patient through the binocular visual feedback. Audio-visual guidance therefore has advantages compared to audio or visual guidance only.
For tangential treatment of the breast only, with respiratory gating, CTV coverage between 90% and 110% has been reported in one study [Citation13]. The same CTV coverage is reported for target volumes including lymph nodes in addition to remaining breast tissue [Citation9,Citation10,Citation13]. The constraints on PTV coverage in our study, V95% ≥ 98%, were stricter.
We have similar target coverage in the DIBH and FB plans. When comparing dose to organs at risk, it is essential that the target coverage is as equal as possible.
The cardiac and pulmonary doses for both FB and DIBH were higher than previously demonstrated. Taylor et al. [Citation19] showed an average mean dose for FB of 2.3 Gy to the heart and 7.6 Gy to the LAD coronary artery for 50 patients treated with 2.67 Gy/fraction up to 40 Gy. Stranzl and Zurl [Citation11] demonstrated a mean heart dose of 2.3 Gy with FB vs. 1.3 Gy with DIBH for 22 patients with a prescribed dose of 50 Gy in 2 Gy fractions. For lung tissue, Nemoto et al. [Citation13] found a median V20 of 5.0% (FB) and 4.3% (DIBH) in 21 patients treated with 50 Gy in 2 Gy/fraction. The higher doses shown in our study were probably due to the stricter constraints on PTV coverage. Our study shows the cardiac and pulmonary doses as they would be, with optimal target coverage, which is the aim in radiotherapy, but not always achievable in clinical practice where compromising target dose against organs at risk is a common challenge. It should be noted that the PB algorithm overestimates the target dose as discussed below. However, as most previous studies used the same algorithm, if stated, the comparison of doses is relevant.
The volume of the heart was smaller with DIBH as compared to FB. When the lungs are inflated the intrathoracic pressure increases and the heart is compressed.
The CLD and hence the absolute irradiated lung volume increased when using DIBH as compared to FB. However, since the total lung volume increased considerably (), the relative irradiated lung volume decreased, also indicated by the lung parameters in . Hence the increasing risk of radiation induced pneumonitis with increasing CLD using FB [Citation20,Citation21] may not be valid with DIBH. A relation between CLD and cardiac disease has also been demonstrated [Citation22]. As shown in , MHD and cardiac doses decrease with DIBH indicating a decreased risk of cardiac disease despite increasing CLD.
There was a small, non-significant increase in the contralateral breast dose when using DIBH. Compared to FB, the lung density is decreased with DIBH. Furthermore, when utilizing DIBH, some tissue that would normally have been inside the beam portals in FB, such as heart tissue, is replaced by lung tissue in DIBH, which has a lower density. The photon beam transmission through lung tissue is increased. Hence, the relative dose in the medial and dorsolateral part of the irradiated volume increases, resulting in a small increase in dose to the contralateral breast ().
The effect of the increased photon beam transmission can be partly balanced by increasing the wedge angles and/or the beam energy. To limit the high dose in the medial and dorsolateral part of the irradiated volume, low weighted 15 MV fields were used in this study for both FB and DIBH plans. There was a small, but significant, increase in 15 MV weight in the DIBH plans compared to the FB plans.
We used the Eclipse PB algorithm with modified Batho inhomogeneity correction for dose planning. This algorithm has limitations in the approximation of lateral electron transport, especially in the borderline between tissues with large difference in density, such as lung–breast [Citation23,Citation24]. The dose gradient calculated with PB is too steep, i.e. the lung dose outside the field border is underestimated and the target dose close to the field border is overestimated. The lower the lung tissue density is, the larger is the deviation. The PTV coverage obtained in this study, especially with the decreased lung density for DIBH, is hence not entirely correct. A lower target dose is expected. A 2.7% difference in average PTV dose has been reported for 6 MV with FB [Citation23]. It would be preferable to apply a more advanced algorithm when using the DIBH technique. However, the 50% isodose line has been shown to be nearly independent of calculation algorithm [Citation23]. With a prescription dose of 50 Gy, the clinically relevant heart and lung parameters reported in this study, V25 and V20, either use this isodose line or are very close to it. Reported doses which are not at the 50% isodose line are more uncertain, but can be used to demonstrate the difference between DIBH and FB.
The reason for the small, but significant, difference in CTV and PTV size between DIBH and FB was not found. A similar difference was not seen for the contralateral breast. The CTV/PTV difference between DIBH and FB was too small to influence the result.
Of the 17 patients included in this study, only 12 had left sided breast cancer. With modern breast conserving technique very little tissue is removed in surgery and delineation of the left breast as CTV, for all patients, was therefore considered to be justified.
Mean cardiac and lung doses are usually moderate in low risk breast cancer patients treated with tangential fields to the left breast, featuring modern conformal techniques. However, the LAD coronary artery, due to its anatomical location, is more exposed and in many cases receives a considerable dose, as shown in this study. Considering the long expected survival of the majority of these patients, and the unknown long-term cardiac effects of adjuvant systemic treatment it is important to minimize the doses to organs at risk. The significant reduction of cardiac doses, especially to the LAD coronary artery, is therefore a major advantage of the DIBH technique as compared to conventional FB. An additional benefit is the dose reduction to lung tissue. Patients with breast cancer is one of the largest groups receiving radiotherapy world wide and a reduction in the risk of complications following treatment is of major importance.
IMRT has been favored as an alternative method to reduce cardiac doses. High dose regions in the heart can be avoided with this technique. However, the proximity between CTV and the heart remains, and the heart will be included in most of the beam portals. While reducing the high dose regions in the heart, the volume of low and medium cardiac, pulmonary and contralateral breast doses is increased with IMRT. With DIBH the high cardiac doses can be reduced or removed without these additional low and medium dose regions. On the contrary, respiratory gating with the DIBH technique leads to a significant reduction of heart volume receiving both high and low doses.
In our experience, respiratory gating with the DIBH technique for breast cancer patients is relatively easy to implement in clinical practice compared to other novel techniques in radiotherapy, e.g. IMRT. The dosimetry is, apart from the effect of decreased lung density, equivalent to conventional radiotherapy. At the accelerator each treatment field is normally delivered during one DIBH, and the total time per patient is approximately the same as without respiratory gating. We have not changed any positional verification after the implementation. Patient compliance is high, regardless of age, and the patients are motivated by the opportunity to improve the quality of their own treatment.
Conclusion
In clinical treatment planning it is often impossible to spare organs at risk without compromising PTV coverage. Respiratory gating with DIBH, utilizing audio-visual guidance, allows tangential treatment of left sided breast cancer patients with considerably reduced cardiopulmonary doses without compromising PTV coverage.
Acknowledgements
We thank the Norwegian Cancer Society for the financial support given towards this study and cardiologist Cord Manhenke for supervising delineation of the LAD coronary artery.
Declaration of interest: The authors report no conflicts of interest. The authors alone are responsible for the content and writing of the paper.
References
- Clarke M, Collins R, Darby S, Davies C, Elphinstone P, Evans E, . Effects of radiotherapy and of differences in the extent of surgery for early breast cancer on local recurrence and 15-year survival: An overview of the randomised trials. Lancet 2005;366:2087–106.
- Fisher B, Anderson S, Bryant J, Margolese RG, Deutsch M, Fisher ER, . Twenty-year follow-up of a randomized trial comparing total mastectomy, lumpectomy, and lumpectomy plus irradiation for the treatment of invasive breast cancer. N Engl J Med 2002;347:1233–41.
- Host H, Brennhovd IO, Loeb M. Postoperative radiotherapy in breast cancer–long-term results from the Oslo study. Int J Radiat Oncol Biol Phys 1986;12:727–32.
- Gutt R, Correa CR, Hwang WT, Solin LJ, Litt HI, Ferrari VA, . Cardiac morbidity and mortality after breast conservation treatment in patients with early-stage breast cancer and preexisting cardiac disease. Clin Breast Cancer 2008;8: 443–8.
- Horner M, Ries L, Krapcho M, Neyman N, Aminou R, Howlader N, . SEER Cancer Statistics Review, 1975–2006, National Cancer Institute. Bethesda, MD. Available from: http://seer.cancer.gov/csr/1975_2006/, based on November 2008 SEER data submission, posted to the SEER web site. 2009.
- Gagliardi G, Bjohle J, Lax I, Ottolenghi A, Eriksson F, Liedberg A, . Radiation pneumonitis after breast cancer irradiation: Analysis of the complication probability using the relative seriality model. Int J Radiat Oncol Biol Phys 2000;46:373–81.
- Sixel KE, Aznar MC, Ung YC. Deep inspiration breath hold to reduce irradiated heart volume in breast cancer patients. Int J Radiat Oncol Biol Phys 2001;49:199–204.
- Remouchamps VM, Letts N, Vicini FA, Sharpe MB, Kestin LL, Chen PY, . Initial clinical experience with moderate deep-inspiration breath hold using an active breathing control device in the treatment of patients with left-sided breast cancer using external beam radiation therapy. Int J Radiat Oncol Biol Phys 2003;56:704–15.
- Pedersen AN, Korreman S, Nystrom H, Specht L. Breathing adapted radiotherapy of breast cancer: Reduction of cardiac and pulmonary doses using voluntary inspiration breath-hold. Radiother Oncol 2004;72:53–60.
- Korreman SS, Pedersen AN, Nottrup TJ, Specht L, Nystrom H. Breathing adapted radiotherapy for breast cancer: Comparison of free breathing gating with the breath-hold technique. Radiother Oncol 2005;76:311–8.
- Stranzl H, Zurl B. Postoperative irradiation of left-sided breast cancer patients and cardiac toxicity. Does deep inspiration breath-hold (DIBH) technique protect the heart? Strahlenther Onkol 2008;184:354–8.
- Stranzl H, Zurl B, Langsenlehner T, Kapp KS. Wide tangential fields including the internal mammary lymph nodes in patients with left-sided breast cancer. Influence of respiratory-controlled radiotherapy (4D-CT) on cardiac exposure. Strahlenther Onkol 2009;185:155–60.
- Nemoto K, Oguchi M, Nakajima M, Kozuka T, Nose T, Yamashita T. Cardiac-sparing radiotherapy for the left breast cancer with deep breath-holding. Jpn J Radiol 2009;27: 259–63.
- Mjaaland I, Segers RM, Dybvik KI, Bjerkeset O, Kvaløy JT, Heikkilä R. [Recurrence and survival after breast-conserving treatment of breast cancer]. Tidsskr Nor Laegeforen 2010;130:370–4.
- George R, Chung TD, Vedam SS, Ramakrishnan V, Mohan R, Weiss E, . Audio-visual biofeedback for respiratory-gated radiotherapy: Impact of audio instruction and audio-visual biofeedback on respiratory-gated radiotherapy. Int J Radiat Oncol Biol Phys 2006;65:924–33.
- Cervino LI, Gupta S, Rose MA, Yashar C, Jiang SB. Using surface imaging and visual coaching to improve the reproducibility and stability of deep-inspiration breath hold for left-breast-cancer radiotherapy. Phys Med Biol 2009;54: 6853–65.
- Storchi PR, van Battum LJ, Woudstra E. Calculation of a pencil beam kernel from measured photon beam data. Phys Med Biol 1999;44:2917–28.
- Webb S, Fox RA. Verification by Monte Carlo methods of a power law tissue-air ratio algorithm for inhomogeneity corrections in photon beam dose calculations. Phys Med Biol 1980;25:225–40.
- Taylor CW, Povall JM, McGale P, Nisbet A, Dodwell D, Smith JT, . Cardiac dose from tangential breast cancer radiotherapy in the year 2006. Int J Radiat Oncol Biol Phys 2008;72:501–7.
- Das IJ, Cheng EC, Freedman G, Fowble B. Lung and heart dose volume analyses with CT simulator in radiation treatment of breast cancer. Int J Radiat Oncol Biol Phys 1998; 42:11–9.
- Kong FM, Klein EE, Bradley JD, Mansur DB, Taylor ME, Perez CA, . The impact of central lung distance, maximal heart distance, and radiation technique on the volumetric dose of the lung and heart for intact breast radiation. Int J Radiat Oncol Biol Phys 2002;54:963–71.
- Correa CR, Das IJ, Litt HI, Ferrari V, Hwang WT, Solin LJ, . Association between tangential beam treatment parameters and cardiac abnormalities after definitive radiation treatment for left-sided breast cancer. Int J Radiat Oncol Biol Phys 2008;72:508–16.
- Knoos T, Wieslander E, Cozzi L, Brink C, Fogliata A, Albers D, . Comparison of dose calculation algorithms for treatment planning in external photon beam therapy for clinical situations. Phys Med Biol 2006;51:5785–807.
- Fogliata A, Nicolini G, Vanetti E, Clivio A, Winkler P, Cozzi L. The impact of photon dose calculation algorithms on expected dose distributions in lungs under different respiratory phases. Phys Med Biol 2008;53:2375–90.