Abstract
Radiotherapy (RT) always requires a compromise between tumor control and normal tissue side-effects. Technical innovation in radiation therapy (RT), such as three dimensional RT, is now established. Concerning prostate cancer (PC), it is reasonable to assume that RT of PC will increase in the future. The combination of small margins, a movable target (prostate), few fractions and high doses will probably demand dynamically positioning systems and in real time. This is called four dimensional radiotherapy (4DRT). Moreover, biological factors must be included in new treatments such as hypofractionation schedules. This new era is called five dimensional radiotherapy, 5DRT. In this paper we discuss new concepts in RT in respect to PC.
Radiotherapy (RT) always demands a compromise between tumor control and side-effects. Undesirable side-effects are the result of dose delivered to non-tumor tissue or healthy organs, and can be reduced if the irradiated volume is minimized. However, due to different errors in the radiotherapy chain, and tumor movements, dose to healthy tissue/organs cannot be totally avoided. Radiotherapy will therefore always be associated with some degree of side-effects [Citation1].
Radiotherapy is and will be an important modality in the treatment of PC. There are several randomized studies showing a clear survival benefit of RT in the treatment of PC and RT shall be considered alone or adjuvant to surgery and hormone therapy [Citation2–4].
The goal of developments in radiation therapy is twofold: (1) improve tumor control and/ or (2) decrease side-effects. Suit 2002 [Citation5] has listed up 20 major contributions or steps in the development of radiotherapy from the development of the gantry to computer tomography based dose planning and three dimensional radiotherapy (3DRT).
3DRT became available in the beginning of the 1990s. The Nordic collaboration in the CART program (computer aid in radiotherapy) was an important contribution to this development, see [Citation6]. In these systems the anatomy of the target could be more complex and the margin surrounding the tumor viewed in three dimensions. Compensation for dose absorption in surrounding organs/ tissue (such as the pelvis) became better. Possibly, 3DRT is one of the greatest single improvements in radiation therapy.
Figure 1. The Nordic CART (Computer Aid in RadioTherapy) programme (with permission from Hans Dahlin). The drawing summarises 3DRT as we know it today.
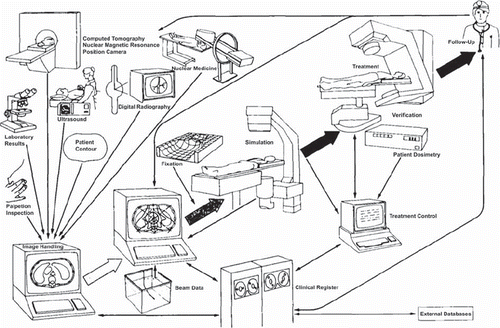
Even if 3DRT can account for a more complex anatomy, it is still difficult in clinical practice to modulate the beam in the axial direction. Wedges can compensate for some inhomogeneous contours, but “banana-shaped” or concave targets remain difficult to treat with appropriate dose distributions.
The introduction of Intensity modulated radiotherapy (IMRT), or later developments VMAT (volumetric modulated arc therapy) or Rapid Arc, made it possible to treat banana-shaped targets (lymph node volumes, prostate with seminal vesicles). Proton beams have used since the 1940s, but the accessibility has been low. In recent years, however, the number of facilities increased, and thus possibilities to treat more patients. Because of limitations of space, and the complexity of these techniques, the interested reader is referred to books such as “The Technical Basis of Radiation Therapy, 4th ed.”. Springer –Verlag, 2006.
It has always been important to be aware of and control movements of the target and patient. In the use of IMRT and protons this is even more important. Displacement errors during IMRT treatments can significantly change the outcome of treatment. Considering that the treatment time for IMRT is two to three times longer then that for conventional 3DRT and the steep dose gradient in protons, the need for improved and continuous patient/ target positioning is obvious.
With better positioning of the target and smaller margins this problem can possibly be reduced. Positioning problems associated with 3DRT and IMRT has led to the development of Image Guided Radiotherapy (IGRT). This development has accelerated during recent years and IGRT has initiated a new era in radiation therapy, namely “four-dimensional radiation therapy” (4DRT).
One major disadvantage of 3DRT is that all treatments are based on the anatomy as defined by the treatment planning CT prior to the actual treatment planning. In an optimal 4DRT system positioning information during irradiation is feedback to the treatment planning system for online corrections. In the clinic this is very complicated and maybe unnecessary to compensate for small movements.
In the case of prostate cancer, and most other tumors, the development of the treatment protocol involves five critical steps [Citation1,Citation7]. The first step involves the diagnostic procedures including determination of the spread of the tumor (e.g., prostate only or lymph node involvement). Next, what is the best treatment for each patient. Third, if radiation therapy is selected, the target(s) must be accurately defined. Fourth, the treatment plan must be optimized (e.g., 3DRT, protons or IMRT) and, finally, the treatment must compensate for inter/intra-treatment variations in position of the patient and the prostate (QA, quality assurance).
In this article we will discuss the last three steps from a 4DRT point of view and discuss necessary developments and improvements of radiation therapy in general and radiation therapy of prostate cancer in particular for the next dimension in radiation therapy – namely 4DRT.
We will also discuss the concepts of biological modeling of the physical dose distribution in 4DRT, and this will be referred to as “five dimensional radiotherapy”, 5DRT. This is the first time the concept of radiobiology will be integrated with the physical dose, and this is possible because of the exceptional control of the dose distribution using 4DRT.
Material and methods
The 4DRT and certainly the 5DRT era is in its formative stage In April 2005 there were approximately 20 references found on on 4D radiotherapy (RT) or four-dimensional RT (depending on search items) in “Pubmed”. On the Internet there were approximately 5 000 references and this reflects the fact that there were multiple 4D projects globally. In 2010 there are approximately 18 500 Internet hits and approximately 400 hits on Pubmed, although some are not fully appreciable.
There are also numerous publications and projects concerning radiotherapy and movement in the 3DRT milieu and aspects from selected articles will be discussed. Several of these articles could be classified as a 4DRT-publication, but the awareness of the technique has been minimal (that is, the keywords on certain articles do not necessarily include 4D, even though the article itself contains 4D information). This makes it possible that we might miss important contributions in our discussion.
Results & discussion
There is no clear definition of 4DRT. 4DRT can be considered as the logical progression forward from 3DRT. 3DRT treatment is often referred to as any treatment using a 3D based dose-planning system and computer tomography (CT). Since 3DRT is a static situation, 4DRT should be considered to be any treatment which dynamically compensates for target or patient movements during radiotherapy. On the other hand 5DRT is a stand alone concept merging physical and technical aspects of RT with radiobiology. It is of course possible to perform 3DRT and include biological information, but it is impossible to have a definition including all combinations. We e suggest that the most advanced technology is used as part of the definition (5DRT>4DRT>3DRT). It is also important to distinguish the six geometric dimensions (X,Y,Z and rotation) from the different levels (dimensions) of radiotherapy. Although they are linked they describe different aspects of RT.
The definition of 4DRT does not include treatments were motion has been taken in account by merely adding a margin around the tumor. With this definition in mind, many of current standard treatments cannot be considered as being true 4DRT although they have the technical possibilities to do online corrections.
The first articles in which the term 4DRT is found is in the late 1990s [Citation8]. There are also authors with a slightly different approach, namely that of including time in a biological model, but today most authors define the motion of the target and its effect on the physical dose distribution as the fourth dimension. This is in concordance with the 4D imaging literature [Citation9]. We suggest that biological considerations of the physical dose distribution should be defined as the fifth dimension if this is applied to the treatment directly (that is not just during follow-up), and we will briefly discuss the concept.
Most radiotherapy publications refer to 4DRT in relation to moving targets due to respiratory movement (lung, liver and breast) and prostate cancer is only briefly discussed [Citation9]. The development follows the evolution of the computer tomography [Citation10,11]. However, the concerns about positioning and moving targets is as old as that of radiotherapy. It is also notable that most of the problems discussed in the literature today, were outlined by the Nordic CART project in the 1970s (). This relates to the fact that the development of modern radiotherapy is closely linked to the development of computers and imaging devices – 4DRT could hardly be done using laser beams and skin marks.
Two publications worth mention are the entire issue of Seminars in Radiation Oncology [Citation11] dealing with moving targets and 4DRT and a recent review by Bucci et al. [Citation12]. These articles present state of the art procedures compensating for moving targets during radiotherapy.
For the clinically oriented reader, literature about moving targets and techniques for dealing with the problem is sometimes confusing for two basic reasons. First, techniques like 3DRT, IMRT, IGRT and 4DRT are used as if they were independent techniques. In clinical practice 3DRT and 4DRT are the level of treatment precision required, and IMRT, IGRT and are the techniques used to achieve that precision. Second, frequently, positioning only refers to the final step in the treatment chain, but in reality, the 4DRT concept includes all steps from dose planning to the last moment of the treatment.
4D computer tomography (4DCT) and treatment planning
Motion of the target during the dose planning CT can change the shape of the target significantly (Seminars), and this is summarized in . Two targets (A and B) are scanned with five CT-slices (1-5). Below are the two resulting 3D images based on the scans. Due to different motion pattern two totally different shapes of the target will be stored in the treatment planning system (A1-5 and B1-5). If we use a high precision RT technique with small margins, this error alone can drastically effect the treatment and it is important to monitor target and patient position throughout the whole radiotherapy chain [Citation13].
Figure 2. A schematic view of the result of movements during the dose planning CT. The target A and B is due to movements displayed as A1-5 and B1-5 respectively due to movements. For details, see text.
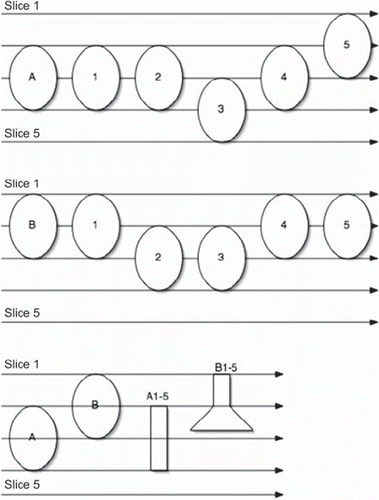
If we assume that the movement is +/- 2cm (a most common margin) and we divide it in steps of 5 mm, we have nine separate locations of the structures. If the treatment plan has three portals the number of combinations in this very simple example is 9 × 9 × 9= 729. It is not possible to produce different dose plans for all these combinations. This emphasizes the fact that in clinical practice it is not and it will not be possible to perform ultimate 4DRT, because the number of combinations is to great and probably are not clinically relevant
Four dimensional imaging devices are today well established and as conventional CTs were a major step forward for RT, 4DCTs will be important for RT in the future. This also stresses the importance of a nomenclature and standards for 4DRT in order to stimulate technical development [Citation10–12].
An interesting new field of avoiding dose to OAR is the introductions of spacers separating the OAR from the target area, thus reducing the dose to the OAR [Citation13]. These must of course be used on the CT and can possible also reduce the motion of the prostate.
Prostate motion
The prostate can move relative to the beam as a part of a patient movement or internally. With laser positioning, displacement errors of several centimeters can be noted [Citation14]. refers to a 20 minute recording of prostate movements, using an electromagnetic positioning implant (Raypilot, Micropos Medicial). The maximum error in this example is approximately 14 mm. Usually, prostate movements are minimal (millimeters), but large errors can occur and this is important with fewer fractions and/or long treatment times.
Electronic portal imaging (EPI), cone computer tomography (cone CT) and IGRT
EPI is probably the most commonly used motion detecting device in clinical practice and is the basis of IGRT. Contrast in an EPI image can be enhanced by using markers [Citation15]. Markers in the target-volume (tumor) will also increase the resolution, since EPI does not visualize soft tissue. EPIs are easy to use and give an x-ray verification of the treatment. It is possible to monitor the motion continuously, but this requires manual inspection (although automatic systems have been developed). The quality of EPI in high voltage therapy is sometimes very poor for all portals. EPIs cannot detect movements axial to the beam, but this is seldom a problem for a treatment field. An additional disadvantage of the EPI is that personnel must leave the room during positioning of the patient.
Cone CT was first discussed in isotope applications in the 1980–1990s. Today it is used for RT and the principle is to use the whole detecting area of the EPI (not just a small pencil beam such as that in a standard CT). One can use the treatment beam, but it is also possible to improve the image quality by using standard x-ray tubes mounted on the treatment gantry (e.g., Electa Synergy). Cone CTs are closely linked to IMRT and the development of VMAT and Rapid Arc but do not solve the problem shown in . It is possible to visualize the body, bone and target, but it is not possible in clinical practice toperform 4DRT. The largest disadvantage with cone CT is that it cannot detect motion during treatment.
Respiration and gating
Most of the work on 4DRT is on motion due to respiration (lung, liver and breast). In this field the problem of is obvious. If one assume that a certain filling of the lung is proportional to the position of the target, it is possible to monitor the respiration and use the flow of air as a surrogate for target position. Once the position is known you can treat in two principle different ways, see . Either you follow the tumor and treat continuously or you can treat only in well defined periods of the respiration cycle. The latter will be more time consuming, but is probably easier to plan and perform. However, it is important to notice that the accelerator must have the possibility to start and stop irradiating with small time intervals.
IMRT, VMAT, Rapid Arc and 4DRT
As mentioned above, IMRT made it possible to treat banana-shaped targets such as lymph node volumes and prostate with seminal vesicles. One problem with IMRT is the larger volume of healthy tissue receiving low dose irradiation. Hall and Wuu (2003) have estimated that this increased volume of normal tissue irradiation, will almost double the incidence of second malignancies [Citation17]. VMAT and Rapid Arc can be considered as IMRT with an infinite number of portals. The low-dose volume using VMAT and Rapid Arc seems to lower and treatment time is shorter. Possible advantages in different tumors are currently being investigated [Citation16].
The major step in dose escalation and side-effect reduction is to reduce the Planning Target Volume (PTV), and IMRT, Rapid Arc and VMAT makes it possible to have a uniformly small margin around any shaped target. On the other hand – small margins stress the importance of continuous positioning monitoring and management of the deviation of the position.
Real time radiotherapy
In order to perform real time corrections in clinical practice, the positioning device must determine the position of the target without human interference. This can be done by using continuous diagnostic x-rays with auto-detection of the target or marker, optical systems (only surface), implanted isotopes, or by using electromagnetic positioning devices (EMP). These techniques have their advantages and disadvantages, but EMP is the only technique which can determine a target position in the body, such as the prostate, without adding more ionizing radiation to the patient.
There are two EMP systems available, Calypso Medical and Micropos Medical [Citation18]. describes one EMP system. A transmitter is implanted near the target and an external antenna (in the treatment table) tracks the signal and thus the position of the target can be determined at sub-millimeter levels. EMP is non-ionizing and the system can therefore be used with personnel in the room.
Figure 4A. The treatment table “listen” to the signal from the non-permanent implant and the prostate is localised with sub-millimetre accuracy. The positioning is done continuously with adding more ionisation to the patient (with permission from Micropos Medical, Gothenburg, Sweden). B An implant in the target volume (to the left).
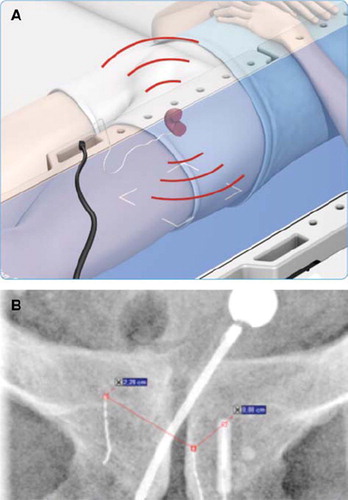
In the treatment of prostate cancer, the combination of small margins, a moving target, few fractions and high doses requires very high precision, and probably demands more than one positioning system. At a minimum, one of these systems should be real time and continuously monitoring.
Biological models and 5DRT
The era of Radiobiology started at Radiumhemmet, Karolinska Hospital, by Magnus Strandquist which combined time and fractionation in the “Stradquist diagram” [Citation19]. Today the most common calculation is the Linear Quadratic (LQ) and BED (Biological Effective Dose) formula introduced by John Fowler [Citation20] using the alfa/beta value. One disadvantage of this particular formula is that it is not suited for heterogeneous dose distributions. Lennernäs et al. have further developed the LQ/BED formula to incorporate heterogeneous dose distributions, making the it suitable for 4DRT. This formula is called the Dose Volume Inhomogenity–BED (DVIC-BED).
Five dimensional radiotherapy (5DRT) is defined as any biological parameter (i.e., sensitivity to RT, drug interactions or résistance to RT) that can alter the outcome of radiotherapy. The 5DRT concept is important to consider in high dose treatments of PC such as high dose rate brachytherapy or hypofractionation. The aim of 5DRT is to, in the clinic, to integrate biological factors and the physical dose distribution over time (fractionation). Five dimensional radiotherapy, is also essential for understanding side-effects of radiotherapy. For PC better understanding of the nature of PC in individual patients will also improve the selection of patients for curative treatments or watchful waiting [Citation21].
For PC, 5DRT is important due to the biological nature of PC and all the possible radiation treatments with different fractionation and doses available. However, if 4DRT has recently been established the era of 5DRT has, possibly, just begun. For example, PC is considered to be a slowly proliferating cancer during treatment [Citation22]. However, recently Thames et al. showed a 6% decrease in biochemical control if the treatment time was extended by one week [Citation23].
During the last decade IMRT in a 3DRT milieu has become very common. One of the goals for QUANTEC (Quantitative Analyses of Normal Tissue Effects in the Clinic) is to summarize 3D dose volume data into constraint parameters to improve IMRT dose planning [Citation24]. However, as pointed out by QUANTEC, data collected from 3DRT is not optimal for the judgment of individual dose plans, since NTCP (Normal Tissue Complication Probability) data extracted from 3DRT are population-based estimates based on a single planning CT with no account for anatomic variation (compared to 4DRT) [Citation25].
The QUANTEC group outlines several other problems which can relate to 5DRT, such as drug interactions, comorbidity and individual radiosensitivity. Methodological problems with side-effect sensoring and determination of the delivered dose to the OAR or target in fractionated RT is also highlighted [Citation24].
The group identified research priorities reflecting the shortcomings of 3DRT data and summarizes that clinical judgment should not be replaced by NTCP or other constraint parameters for the individual patient [Citation27]. It is also notable that the parametres are extracted from 3DRT 1.8–2Gy/fraction and other fractions schedules such as hypofractionation, should be adjusted to conventional fractionation [Citation26]. However, it is not self evident that the Linear Quadratic (LQ) formula which contains no volume parameter is optimal for this conversion for OAR with a non-homogeneous dose distribution.
Conclusion
Radiotherapy is an important modality in the treatment of PC. It can be used either alone with or without dose escalation, or in combination with surgery or hormone therapy. It is reasonable to assume that RT of PC will increase in the future. The development of techniques for 4DRT are important techniques for optimizing RT for PC and to improve constraints for IMRT. Furthermore, the combination of small margins, a moving target, few fractions and high doses will probably require more than one positioning system and at least one of these should be real time. The development and research in 5DRT should be accelerated in order to improve risk assessment for an individual patient. It is important to evaluate new technologies in respect to excising guidelines, but it is equally important it is that new technologies are investigated and also easier used in the clinic if found to be of advantageous for individual patient [Citation28].
Declaration of interest: Bl Lennernäs, Sten Nilsson and Seymour Levitt are founders of Micropos Medical.
References
- Adolfsson J. Screening for prostate cancer – will we ever know? Acta Oncol 2010;49:275–7.
- Widmark A, Klepp O, Solberg A, Damber JE, Angelsen A, Fransson P, . Endocrine treatment, with or without radiotherapy, in locally advanced prostate cancer (SPCG-7/SFUO-3): An open randomised phase III trial. Lancet 2009;373(9660):301–8.
- Bolla M, van Poppel H, Collette L, van Cangh P, Vekemans K, Da Pozzo L, . Postoperative radiotherapy after radical prostatectomy: A randomised controlled trial (EORTC trial 22911). Lancet 2005;366(9485):572–8.
- Gatta G, Zigon G, Buemi A, Coebergh JW, Colonna M, Contiero P, . Prostate cancer treatment in Europe at the end of 1990s. Acta Oncol 2009;48:867–73.
- Suit H. The Gray Lecture 2001: Coming technical advances in radiation oncology. Int J Radiat Oncol Biol Phys 2002; 53:798–809.
- Landberg T. Progress in radiotherapy. Acta Oncol 1995;34: 1023–9.
- Ikushima H. Radiation therapy: State of the art and the future. J Med Invest. 2010;57:1–11.
- Gottlieb KL, Hansen CR, Hansen O, Westberg J, Brink C. Investigation of respiration induced intra- and inter-fractional tumour motion using a standard Cone Beam CT. Acta Oncol 2010;49:1192–8.
- van Dam IE, van Sörnsen de Koste JR, Hanna GG, Muirhead R, Slotman BJ, Senan S. Improving target delineation on 4-dimensional CT scans in stage I NSCLC using a deformable registration tool. Radiother Oncol 2010;96:67–72.
- Levitt SH, Perez CA, Hui S, Purdy JA. Evolution of computerized radiotherapy in radiation oncology: Potential problems and solutions. Int J Radiat Oncol Biol Phys 2008;70: 978–86.
- Keall P.4-dimensional computed tomography imaging and treatment planning. Semin Radiat Oncol 2004;14:81–90.
- Bucci MK, Bevan A, Roach M 3rd. Advances in radiation therapy: Conventional to 3D, to IMRT, to 4D, and beyond. CA Cancer J Clin 2005;55:117–34.
- Isacsson U, Nilsson K, Asplund S, Morhed E, Montelius A, Turesson I. A method to separate the rectum from the prostate during proton beam radiotherapy of prostate cancer patients. Acta Oncol 2010;49:500–5.
- Lennernäs B, Letocha H, Rikner G, Magnusson A, Nilsson S. Field displacement during external radiotherapy in prostatic adenocarcinoma treated with radioactive 198Au implants and external irradiation. Acta Oncol 1995; 34:959–64.
- Poulsen PR, Cho B, Sawant A, Ruan D, Keall PJ. Dynamic MLC tracking of moving targets with a single kV imager for 3D conformal and IMRT treatments. Acta Oncol 2010;49: 1092–100.
- Johansen S, Cozzi L, Olsen DR. A planning comparison of dose patterns in organs at risk and predicted risk for radiation induced malignancy in the contralateral breast following radiation therapy of primary breast using conventional, IMRT and volumetric modulated arc treatment techniques. Acta Oncol 2009;48:495–503.
- Hall EJ, Wuu CS. Radiation-induced second cancers: The impact of 3D-CRT and IMRT. Int J Radiat Oncol Biol Phys 2003;56:83–8.
- Kindblom J, Ekelund-Olvenmark AM, Syren H, Iustin R, Braide K, Frank-Lissbrant I, . High precision transponder localization using a novel electromagnetic positioning system in patients with localized prostate cancer. Radiother Oncol 2009;90:307–11.
- Strandqvist M. Studien iiber die kumulative Wirkung der Rontgenstrahlen bei Fraktionierung. Erfahrungen aus dem Radiumhemmet an 280 Haut- und Lippenkarzinomen. German. Acta Radiol 1944;(Suppl 55):1–300.
- Fowler JF. The radiobiology of prostate cancer including new aspects of fractionated radiotherapy. Acta Oncol 2005;44: 265–76.
- Adami HO. The prostate cancer pseudo-epidemic. Acta Oncol. 2010;49:298–304.
- Gao M, Mayr NA, Huang Z, Zhang H, Wang JZ. When tumor repopulation starts? The onset time of prostate cancer during radiation therapy. Acta Oncol 2010.
- Thames HD, Kuban D, Levy LB, Horwitz EM, Kupelian P, Martinez A, . The role of overall treatment time in the outcome of radiotherapy of prostate cancer: An analysis of biochemical failure in 4839 men treated between 1987 and 1995. Radiother Oncol 2010;96:6–12. Epub 2010 Apr 17.
- Bentzen SM, Constine LS, Deasy JO, Eisbruch A, Jackson A, Marks LB, . Quantitative Analyses of Normal Tissue Effects in the Clinic (QUANTEC): An introduction to the scientific issues. Int J Radiat Oncol Biol Phys 2010;76(3 Suppl):S3–9.
- Marks LB, Yorke ED, Jackson A, Ten Haken RK, Constine LS, Eisbruch A, . Use of normal tissue complication probability models in the clinic. Int J Radiat Oncol Biol Phys 2010;76(3 Suppl):S10–9.
- Michalski JM, Gay H, Jackson A, Tucker SL, Deasy JO. Radiation dose-volume effects in radiation-induced rectal injury. Int J Radiat Oncol Biol Phys 2010;76(3 Suppl):S123–9.
- Liu M, Moiseenko V, Agranovich A, Karvat A, Kwan W, Saleh ZH, . Normal Tissue Complication Probability (NTCP) modeling of late rectal bleeding following external beam radiotherapy for prostate cancer: A Test of the QUANTEC-recommended NTCP model. Acta Oncol 2010;49:1040–4.
- Stensvold A, Dahl AA, Fosså SD, Axcrona K, Lilleby W, Brennhovd B, . Clinicians’ use of guidelines as illustrated by curative treatment of prostate cancer at a comprehensive cancer center. Acta Oncol 2010;50:408–14.