Abstract
Purpose. To report early clinical experience in stereotactic body radiation therapy (SBRT) delivered using volumetric intensity modulated arc therapy with RapidArc (RA) in patients with primary or metastatic tumours at abdominal sites. Material and methods. Thirty-seven consecutive patients were treated using RA. Of these, 16 had primary or metastatic liver tumours, nine had pancreatic cancer and 12 a nodal metastasis in the retro-peritoneum. Dose prescription varied from 45 to 75 Gy to the Clinical Target Volume in 3 to 6 fractions. The median follow-up was 12 months (6–22). Early local control and toxicity were investigated and reported. Results. Planning objectives on target volumes and organs at risk were met in most cases. Delivery time ranged from 2.8 ± 0.3 to 9.2 ± 2.4 minutes and pre-treatment plan verification resulted in a Gamma Agreement Index from 95.3 ± 3.8 to 98.3 ± 1.7%. At the time of analysis, local control (freedom from progression) at six months, was assessable in 24 of 37 patients and was achieved in 19 patients with a crude rate of 79.2%. Seven patients experienced treatment-related toxicity. Three patients experienced a mild and transient G1 enteritis and two showed a transient G1 liver damage. Two had late toxicity: one developed chronic enteritis causing G1 diarrhoea and G1 abdominal pain and one suffered at three months a G3 gastric bleeding. No patients experienced G4 acute toxicity. Conclusions. SBRT for abdominal targets delivered by means of RA resulted to be feasible with good early clinical results in terms of local control rate and acute toxicity profile. RA allowed to achieve required target coverage as well as to keep within normal tissue dose/volume constraints.
Stereotactic body radiation therapy (SBRT) has proved its efficacy in several patient populations with primary and metastatic limited tumours [Citation1]. In particular, SBRT may be appropriate for selected patients with oligo-metastatic disease, defined as less than five lesions [Citation2] or organ-confined limited volume primary tumours. Abdominal SBRT has been reported with reference mainly to primary and secondary liver tumours, as well as pancreatic and renal tumours [Citation1,Citation3–11]. Also, SBRT for abdominal lymph-node metastases has been reported in a few series as a specific topic [Citation11–15].
It is known that in the setting of limited tumour burden, SBRT leads to local control rates higher than 70–80% [Citation1,Citation16], which may improve survival and quality of life.
Although abdominal SBRT was traditionally delivered using multiple arc conformal techniques, the introduction of fast Volumetric Modulated Arc Therapy (VMAT) allowed treatment time to be reduced and thus it may reduce both patient discomfort and the potential for intra-fraction motion [Citation17]. We already investigated the role in this patient population of VMAT delivered using RapidArc (Varian Medical Systems, Palo Alto, CA) [Citation18]. RapidArc (RA) has been investigated previously for some other clinical cases [Citation19–26] showing significant improvements over other advanced techniques in terms of dose distributions and treatment time.
RA is implemented at planning level by means of the Progressive Resolution Optimization (PRO) algorithm in the Eclipse planning system by Varian. The optimisation process is based on an iterative inverse planning process aiming to simultaneously optimise the instantaneous multi leaf collimator (MLC) positions, the dose rate, and the gantry rotation speed to achieve the desired dose distribution. Delivery is performed on Varian Clinacs as single or multiple arcs with dynamic continuous modulation of MLC shapes, gantry speed and dose rate.
As we found advantages in dose distribution also in this setting, since November 2008 SBRT to abdominal nodes and other abdominal targets has been delivered by means of RA.
The aim of this retrospective study was to evaluate our early experience in terms of technical feasibility, local control rate and acute toxicity profile of SBRT with RA for patients with primary or secondary abdominal tumours.
Material and methods
Population for study
Between October 2005 and March 2010 at Istituto Clinico Humanitas (ICH) we treated 95 patients with primary or metastatic tumours in the abdominal region by linac-based hypo-fractionated SBRT. Here we report data regarding the first 37 consecutive cases treated with SBRT delivered using RA between November 2008 and March 2010. This group of patients included liver tumours (two hepato-cellular carcinomas, 14 metastases), nine primary pancreatic tumours and 12 lymph-nodal metastases.
Disease extension was evaluated in all cases by Computed Tomography (CT)+/2 Magnetic Resonance Imaging (MRI). Presence of metabolic active tumour was confirmed by Positron Emission Tomography (FDG-PET) in 23 patients. In 26 patients the target lesion was a metastasis from different primary tumours, either solitary (15 patients) or a dominant lesion as part of oligo-metastatic disease, defined as less than five lesions (11 patients).
All the patients had been considered unfit for surgery or other non-surgical treatments at the time of radiation. Chemotherapy was stopped at least three weeks before SBRT and withheld until disease progression. Median patient age was 66 years. The most relevant patient, tumour and treatment characteristics, stratified by tumour group, are reported in .
Table I. Main characteristics of patients cohort.
Planning strategy
CT scans for planning were acquired for all patients, all patients were positioned supine with their arms above the head; patients were immobilised by means of a vacuum pillow combined with a thermoplastic body mask including a styrofoam block for abdominal compression to minimise internal organ motion (spontaneous or induced by breathing). Contrast free and Contrast-enhanced planning CT scans were acquired in free quiet breathing mode at 3 mm slice thickness with the patient fixed in a stereotactic body frame (Stereotactic body Frame 3D-Line/Elekta).
The gross tumour volume (GTV) included macroscopic disease on CT as well as on PET if available. The clinical target volume (CTV) was defined equal to the GTV. The planning target volume (PTV) was generated by taking into account both the internal margin (IM) and the set-up margin (SM). IM depends on intra- and inter-fraction organ motion which are not expected to be significant in a short course of radiation for retroperitoneal nodes adjacent to spine and large vessels since these structures are relatively fixed, while it may be relevant for pancreatic and liver tumours [Citation27–31]. Since 4D-CT was not used, the margins compensating for organ motion, for pancreas and liver lesions, were chosen based on the expected impact of abdominal compression, applied for simulation and daily treatment in these patients. Abdominal compression was shown to significantly reduce tumour motion in the lateral, superior-inferior, and overall directions. In particular, abdominal compression can significantly reduce and minimise the motion of the diaphragm and pancreas [Citation32]. Since SM was maintained at a minimum by the cone-beam CT (CBCT) systematic verification of set up variations, the overall CTV-PTV margin was prescribed as 6–15 mm in the cranial-caudal axis and 3–8 mm in the anterior–posterior and lateral axes, allowing mainly for residual intra-fraction organ motion, for intrinsic delineation uncertainties as well as for inaccuracies in CBCT image interpretation.
The main organs at risk (OAR) considered were spinal cord, kidneys, stomach, duodenum, small bowel and liver. Stomach, duodenum and small bowel were contoured when appropriate. Organ motion was taken into account for small bowel by contouring the intestinal cavity (the volume containing bowel loops) [Citation33].
Dose prescription to CTV was set to 45 Gy in 6 daily fractions of 7.5 Gy for nodal metastases and pancreatic tumours, while a dose of 50 or 75 Gy in 3 daily fractions was delivered to liver tumours. Dose was prescribed as mean dose to CTV. Planning objectives to target coverage aimed to cover the CTV with 95% of the prescribed dose and the PTV with 80% of the prescribed dose (except for liver metastases treated at 75 Gy where this threshold was lowered to 67%).
For OARs, plans were required to meet the following objectives (following or adapted from [Citation18]) : D0.1 cm3 for spinal cord <18 Gy (dose at a volume of 0.1 cm3 should be lower than 18 Gy); V15Gy <35% for both kidneys (volume receiving 15 Gy should be lower than 35%), V36Gy <1% for duodenum, V36Gy <3% for stomach and small bowel, V15Gy <(total liver volume minus 700 cm3, i.e. at least 700 cm3 of liver should receive less than 15 Gy) for liver. In addition D0.5cm3 <30 Gy for duodenum, stomach and small bowel was considered as a secondary objective.
All plans were designed and optimised for a Varian Clinac 2100CD equipped with a Millennium multileaf collimator (MLC) with a leaf width of 5 mm at the isocentre in the inner 20 cm and 10 mm for the outer 2×10 cm for a 6M photon beam. Further details on RA technique can be found in [Citation19,Citation20]. RA plans were individually designed using full or partial single or multiple arcs according to resulting quality of dose distributions. Number and length of arcs was chosen to obtain the best adherence to planning objectives for each patient.
All dose distributions were computed with the Analytical Anisotropic Algorithm (AAA) implemented in the Eclipse planning system with a calculation grid resolution of at maximum 2.0 mm.
Treatment delivery
Before treatment, each plan was verified to assess dosimetric agreement between computed and delivered dose distributions. This quality assurance process was performed according to the GLAaS method [Citation34,Citation35] based on absorbed dose measurements by means of the electronic portal imager attached to the linac (PortalVision aS1000). Data were analysed with the EPIQA software (EpiDos Srl, Bratislava, Slovakia). Results of measurements were scored in terms of the Gamma Agreement Index (GAI) based on the γ of Low analysis [Citation36] with thresholds: Distance to Agreement = 3 mm, ΔDose = 3%.
Treatment was delivered in three to six consecutive working days, with the patient keeping a three-hour fast to avoid gross displacement of stomach and bowel. Treatment delivery included stereotactic frame localisation and cone beam CT imaging (CBCT) in the first session aiming at a preliminary isocentre positioning while for following fractions, patient set-up was realised by means CBCT image guidance with, eventually, on-line couch adjustment at each fraction. Couch repositioning was operated after automatic matching of CBCT images to reference planning CT, followed by manual refining. Matching was performed on bones and, when visible, on tumours and other soft tissue structures (e.g. main blood vessels).
Analysis, follow-up and statistics
For each group of patients, technical parameters of the delivery were scored in terms of number of arcs, total number of monitor units (MU), monitor units per Gy (MU/Gy), total beam on time (from electronic delivery records). Dosimetric quality of treatments was measured from dose volume histogram (DVH) analysis. For CTV and PTV the following data (whenever appropriate) were reported: target coverage (mean, D1%, D95%, V67%, V80%, V95%, V107%), homogeneity (Standard Deviation [Citation37]) and conformity for PTV (CI95%). CI was defined as the ratio between the volume of patient irradiated at 95% of the prescribed dose and the CTV volume. CI was defined relative to CTV because of the planning objectives described above. For OARs, the mean dose, the maximum dose (Dxcm3) and appropriate values of VxGy (volume receiving at least x Gy) were scored.
Acute radiation induced toxicities were scored according to NCI Common Terminology Criteria for Adverse Events (CTCAE version 3.0 [Citation38]). Patients were followed up in three months interval. Local control was evaluated on CT imaging at three and six months and every six months afterwards. In patients who had a PET before SBRT, also metabolic response was evaluated at six and 12 months. The median follow-up was 12 months (range 6–22). Response was evaluated on CT imaging at first follow-up according to WHO criteria [Citation39]. Local control was evaluated in terms of freedom from local progression (FFLP), defined as no evidence of tumour growth inside the target lesion, calculated from the date of the last radiation treatment.
Results
Technical and dosimetric findings
shows examples of dose distributions for four patients. PTV and organs at risk are outlined as solid lines in the images. report the average dose volume histograms of the four groups of patients for PTV and OARs as well as for healthy tissue. reports the summary of technical delivery parameters as well as the results of the pre-treatment quality assurance measurements (GAI). and summarises the results of the DVH analysis.
Figure 1. Examples of dose distributions for the four groups of patients. Colourwash scale is reported on the figure. Solid lines represents target volumes and organs at risk.
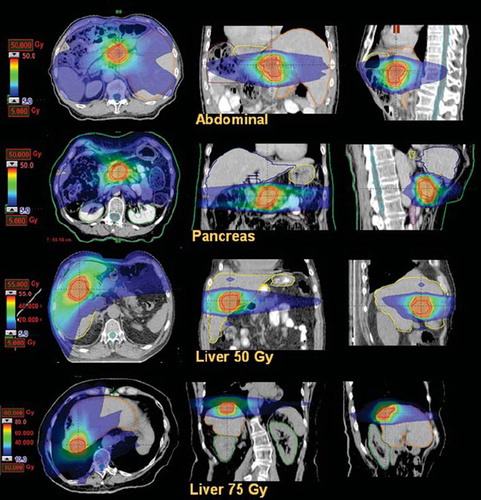
Figure 2. Average dose volume histograms for target volumes and healthy tissue for the abdominal metastases patients. Dashed lines represent the inter-patient variability at one standard deviation.
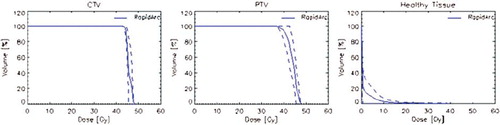
Figure 3. Average dose volume histograms for target volumes and healthy tissue for the pancreas patients.
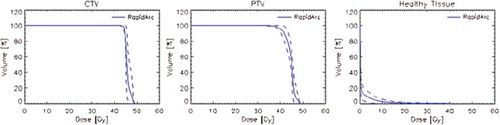
Figure 4. Average dose volume histograms for target volumes and liver for the hepatic patients treated at 45 Gy.
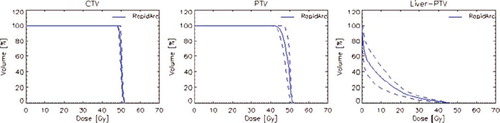
Figure 5. Average dose volume histograms for target volumes and liver for the hepatic patients treated at 75 Gy.
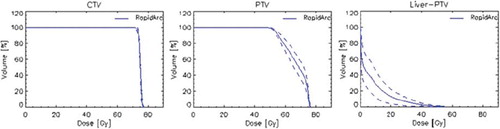
Table II. Main technical features of delivered treatments.
Table III. Summary of dose volume histogram analysis for CTV, PTV and healthy tissue.
Table IV. Summary of dose volume histogram analysis for organs at risk.
Abdominal and pancreas patients were all treated with one or two arcs and the average beam on time did not exceeded three minutes per fraction of 7.5 Gy (moderate reduction of gantry speed was observed in all cases to compensate for the maximum dose rate of 600 MU/minute available at the linac). The liver cases, with a higher dose per fraction (of 16.7 or 25 Gy) required longer beam on time (from six to nine minutes) and a considerable reduction of the rotational gantry speed with respect to its maximum of 4.8°/s was necessary. Liver patients were treated with either two or three arcs. Average MU/Gy was in the order of 200–220. Pre-treatment quality assurance measurements, stratified per patient group, showed satisfactory results with a minimum deviation between groups of less than 3% and no statistically significant differences. In all cases, average GAI exceeded the acceptance threshold of 95%. In total, 10 of a total of 112 arcs had a GAI >90% but <95% (mean 93.6% ± 1.3, range: 91.3–94.8). The results were considered clinically acceptable.
Concerning target volumes, the main objectives on coverage were reached for both CTV and PTV with minor deviations considered clinically negligible. Homogeneity was expressed as standard deviation in Gy and in average was <1.5% of the prescribed dose for CTV and <3.5% for abdominal, pancreas and low dose liver PTVs. Homogeneity was ∼10% for the high dose liver patients because of the lower threshold applied for target coverage (V67%) applied to better protect the rest of the healthy liver tissue.
Planning objectives for organs at risk were reached in most of the cases (e.g. spinal cord or stomach or liver). Depending on anatomical position, dose to duodenum was on average moderately violated in pancreas patients but without evidence of corresponding toxicity (see below). Dose to duodenum, stomach and small bowel was not reported for liver patients because it was below significant levels. The choice to prescribe 50 Gy or 75 Gy, in three fractions, was evaluated in each case in order to respect dose-constraints for OARs previously explained. When OARs objectives were not achievable prescribing 70 Gy, the lower dose of 50 Gy was adopted. High dose liver patients, compared to the low dose group, showed a slightly better sparing of ipsilateral kidneys and of healthy liver itself although not statistically significant.
Tumour response
All patients completed the planned radiotherapy. Tumour response was evaluated on CT imaging at first follow-up, and radiological images after treatment were available in 33 of 37. Among 33 patients evaluable for tumour response at first follow-up visit, a complete response was found in one patient, according to WHO criteria [Citation39]. In particular, the single case of complete response was a metastasis from rectum adenocarcinoma on segment 8 of the liver. The maximum axial diameter of the lesion was 15 mm (CTV = 17mm). Dose prescription on PTV was 50 Gy.
A partial response was achieved within three months after radiation in 14 patients (overall response rate 45.4%). Nine of the 14 partial responses were in the liver, one in the pancreas, four in the lymph-nodes. Eight patients had stable disease (three in irradiated liver lesions, six in pancreas, six in lymph-nodes) and two showed progressive disease at treatment site (pancreas diseases in both cases). Tumour responses were all evaluated with a reduction/no changes/increasing in diameter of the irradiated lesion compared with the initial pre-radiation treatment maximum dimension, according to WHO Criteria, as previously cited.
Local control
At the time of analysis 24 patients had a follow-up longer than six months. Local control at six months, defined as freedom from local progression, was achieved in 19 patients even if in some patients with a concomitant tumour growth at distant sites. The crude rate of local control at six months was 79.2% in the whole group (Crude rate was reported instead of actuarial because of short follow-up. The median follow-up was 12 months (range 6–22)). The local control rate in evaluable cases according to patient groups was: liver: 5/7; pancreatic tumours: 4/6; nodal metastases: 10/11.
Toxicity
Both acute and late toxicities were minimal. A total of three patients experienced a mild and transient G1 acute enteritis and two showed a transient G1 liver damage at blood chemistry two weeks after SBRT. Both toxicities recovered spontaneously within three months after RT. Concerning late toxicities, one patient, treated for a pancreatic cancer adjacent to gastric antrum, suffered at three months a G3 gastric bleeding from an ulcer in the posterior wall of the antrum, which recovered after repeated endoscopic treatments. This patient received a maximum dose of 40.6 Gy to the stomach (mean dose to the entire stomach was 3.4 Gy). This maximum was located proximal to the peripheral area of the PTV. These data were retrospectively assessed on the planning CT, in addition, uncertainties related to stomach motion between fractions might have contributed to increase this estimate of the dose. One patient, treated for a nodal metastasis, complained of late effects: he developed chronic enteritis causing G1 diarrhoea and G1 abdominal pain.
Discussion and conclusions
An increasing experience on the use of SBRT for the treatment of primary and metastatic tumours in several anatomical sites has accumulated in the last decade. SBRT has emerged as a possible non invasive approach for local ablation in some series of selected cancer patients and should generally be combined with systemic therapy [Citation1–16,Citation18]. Surgical resection is the most frequently used approach for the majority of abdominal primary lesions, but also for some metastatic sites, such as in the liver. However, in abdominal sites, SBRT for patients with either organ-confined primary tumours or oligo-metastatic disease may play a major role for improving survival in a clinically significant subset of cancer patients. In this scenario, focal radiotherapy could play a role improving local control of oligo-metastatic focal disease. As smaller foci of metastases are found, high conformal radiation, such as stereotactic radiosurgery, stereotactic body radiotherapy (SBRT) or similar techniques, may well prove more effective than surgery because of decreasing morbidity and the potential of delivering ablative treatment on an outpatient basis [Citation1–16,Citation18]. Although in most cases the radiation sterilisation of a metastatic lesion is not expected to lead to definitive cure, it could be effective in delaying further chemotherapy or at least this may contribute to better quality of life. In a similar way, local treatment by radiation may be beneficial in patients with unresectable pancreatic cancer or hepato-cellular carcinoma. Even if obtaining local control might salvage only few patients, it could be clinically relevant in order to reduce tumour burden or delay further treatments, as well as to preserve quality of life by avoiding symptomatic tumour growth. Although mechanisms of ablative-dose injury remain elusive, in an extensive review, Timmerman pointed out that several prospective trials, using SBRT in multiple organ sites, have now confirmed the efficacy of treatment in a variety of patient populations [Citation1]. As expected on the basis of data from these several datasets [Citation3–18], our preliminary results support the hypothesis that high biologically effective doses may be given safely by markedly hypo-fractionated schedules in abdominal SBRT, provided that dose distribution is carefully tailored according to normal tissues constraints. The potential benefit in terms of quality of life is also supported by the low incidence of acute and late toxicity recorded in the patients analysed in this study. Low toxicity rates have also been reported in other reports dealing with similar populations [Citation3–18]. As pointed out by Hoyer et al. [Citation3], in a mixed population of 64 patients with colorectal-metastases (in the liver, lung, lymph nodes, suprarenal gland), where high doses of 15 Gy x 3 were prescribed, the treatment related toxicity was low for the majority of them. Although the Hoyer's study population is comparable to the one in the present study in terms of heterogeneity of the tumour sites in abdomen, in our case the histo-pathology type of the tumours is various. This aspect is an important bias to consider when we compare data, especially in terms of local control and toxicity. Clearly, considering toxicities, the risk of damaging normal tissue is expected to be related to the site of target and its size. The only G3 toxicity event found in our series was a gastric bleeding in a patient treated for a pancreatic cancer adjacent to gastric antrum, received a maximum dose of 40.6 Gy (mean dose to the organ of 3.4 Gy) to the stomach. As a matter of fact, rates of gastrointestinal toxicity have been reported in other papers dealing with SBRT for pancreatic tumours [Citation8–11]. In a Phase II study of SBRT using a conventional linac, 22 patients were submitted to 45 Gy in 3 fractions and 64% of the patients showed ≥grade 2 toxicity, including a case of non-fatal gastro-intestinal perforation [Citation9]. Another series of 36 patients with non-metastatic, locally advanced, unresectable pancreatic cancer received 3 fractions of 8, 10, or 12 Gy (total dose, 24–36 Gy) of SBRT according to the tumour location in relation to the stomach and duodenum, using fiducial-based respiratory motion tracking on a robotic radiosurgery system [Citation8]. Even if the dose prescription was defined on the relationships between target and organ at risk (proximity for example), nine Grade 2 (25%) and five Grade 3 (14%) toxicities attributable to SBRT occurred. On the whole, the toxicity rates observed in these series were not higher than those observed in similar patients previously treated in our centre with 3D multiple arc techniques. SBRT using RA technique was investigated on lung, spine metastases, and abdominal region [Citation15,Citation18,Citation25,Citation26]. In particular, at our knowledge, in abdominal region, only one paper, including only eight patients treated with RA was published [Citation15].
In the current study, SBRT delivered using RA approach on 37 patients ended with a mild toxicity profile in terms of incidence and severity of the acute and late gastrointestinal symptoms although the limited statistical power prevents drawing absolute conclusions. In our institution, image-guidance by means of CBCT, is daily used for SBRT patients to define and correct setup of each patient. Daily CBCT checking before each session might represent a reason of the low toxicity profile recorded in the current study. The other factor influencing the good tolerability of the treatment can be related to the ability to keep organs at risk dosimetric involvement at very low levels, generally below planning objectives, with the only exception of the duodenum in the case of pancreas patients. Whether patients experience toxicity or not is, in addition, also dependent on size and number of treated targets and their proximity to critical OARs. The limited power of the current study did not allow events to be properly stratified as a function of anatomical localisation of targets. In the present study, the short-term clinical results of abdominal SBRT delivered using of RA were also evaluated. In particular, SBRT was mainly used for patients who were not amendable for surgery or other standard approaches, such as, for example RFA for liver metastasis, and thus could represent a group of patients with a poor prognosis. Nevertheless, data reported in current study showed a good rate of short-term local control, defined as no evidence of tumour growth inside the target lesion, in step with our previous results as well as with several data from literature [Citation3–13,Citation40].
One of the most intuitive criticisms of current study regards the heterogeneity of the population of study composed of a mix of primary and metastatic sites. However, the primary objective of the study was to report the feasibility of SBRT delivered with the RA technique in abdomen tumour sites, where SBRT is not the standard of practice worldwide for this subset of patients. Further studies will analyse more extensive groups of homogeneous patients selected for single primary or metastatic pathology and/or for specific tumour site in abdomen. A drawback of volumetric arc irradiation in the abdomen is that, because of the risk of collisions, non-coplanar arc arrangements are not feasible if full arcs of 360 degrees are applied. Partial arcs, technically achievable with RapidArc, could further improve the dose distribution and this will be part of a next study. In the present study, plans were optimised for each individual patient aiming to achieve the best adherence to dosimetric objectives. This implied usage of one to three full or partial arcs. There is therefore a potential impact in the prolongation of the time needed to deliver a single fraction with consequent discomfort for the patient since, when using multiple arcs, the linear accelerator has some dead time given by its reset cycle. This time can be minimised by using, as done here, opposite gantry rotation directions for consecutive arcs. The total impact of multiple arc usage was anyway minimal and consisted in about 30 extra seconds on average per each additional arc (reset time).
Usage of RapidArc for abdominal targets to be irradiated in few fractions might be exposed to some difficulties due to internal organs motion and possible interplay effects. Ong et al. [Citation41] and Court et al. [Citation42] investigated this issue in the case of lung SBRT and in generic cases. Results for lung SBRT showed that in the case of both excessive multileaf modulation and up to 25 mm of motion, the degree of agreement (GAI) between calculation and dynamic delivery was kept higher than 98%. This was under the assumption that multiple arcs were applied. This fact, strengthens the practice of multiple arc delivery used for this study, although originally motivated by different rational.
Concerning pre-treatment quality assurance, about 10% of the arcs had a GAI inferior to 95% but were considered as acceptable clinically. Reasons for this decision are multiple and mostly related to sensitivity issues. The main aspect is linked to the discrete nature of the gamma analysis and the discrete nature of both the dose calculation grids and the measurements. In fact, recalculating the same gamma with a slightly larger threshold (e.g. DTA = 3.5mm) would have recovered 7/10 of the values to scores higher than 95%. For the remaining three cases, the criteria would have been met with a ΔD threshold of 4%. Anyway, as stated by Courts et al. [Citation42] very complex RA plans also can lead to dosimetric inaccuracies.
In summary, in the current preliminary report, hypo-fractionated stereotactic radiotherapy with RA for abdominal tumour targets were considered to be feasible and able to provide good toxicity profile and clinical results, supporting its role in aggressively treating selected cases with limited tumour burden. Clearly, in the present retrospective analysis, the small number and heterogeneity of the analysed population, and the limited follow-up represent significant limitations in the power of the study. A larger and more homogeneous number of patients and a more prolonged follow-up will be required to confirm our preliminary results, especially in the long-term. Even if most patients eventually show progressive disease, local control achieved by SBRT may have the potential to show significant improvements in quality of life.
Declaration of interest: Dr. L. Cozzi is Head of Research at Oncology Institute of Southern Switzerland and acts as a Scientific Advisor to Varian Medical Systems. Other authors have no conflict of interest.
References
- Timmerman RD, Kavanagh BD, Cho LC, Papiez L, Xing L. Stereotactic body radiation therapy in multiple organ sites. J Clin Oncol 2007;25:947–52.
- Macdermed DM, Weichselbaum RR, Salama JK. A rationale for the targeted treatment of oligometastases with radiotherapy. J Surg Oncol 2008;98:202–6.
- Hoyer M, Roed H, Traberg Hansen A, Ohlhuis L, Petersen J, Nellemann H, . Phase II study on stereotactic body radiotherapy of colorectal metastases. Acta Oncol 2006;45: 823–30.
- Schefter TE, Kavanagh BD, Timmerman RD, Cardenes HR, Baron A, Gaspar LE. A phase I trial of stereotactic body radiation therapy (SBRT) for liver metastases. Int J Radiat Oncol Biol Phys 2205;62:1371–8.
- Mendez Romero A, Wunderink W, Hussain SM, De Pooter JA, Heijmen BJ, Nowak PC, . Stereotactic body radiation therapy for primary and metastatic liver tumors: A single institution phase I-II study. Acta Oncol 2006;45:831–7.
- Katz AW, Carey-Sampson M, Muhs AG, Milano MT, Schell MC, Okunieff P. Hypofractionated stereotactic body radiation therapy (SBRT) for limited hepatic metastases. Int J Radiat Oncol Biol Phys 2007;67:793–8.
- Tse RV, Hawkins M, Lockwood G, Kim JJ, Cummings B, Knox J, . Phase I Study of individualized stereotactic body radiotherapy for hepatocellular carcinoma and intrahepatic cholangiocarcinoma. J Clin Oncol 2008;26:657–64.
- Mahadevan A, Jain S, Goldstein M, Miksad R, Pleskow D, Sawhney M, . Stereotactic body radiotherapy and gemcitabine for locally advanced pancreatic cancer. Int J Radiat Oncol Biol Phys 2010;78:735–42.
- Hoyer M, Roed H, Sengelov L, Traberg A, Ohlhuis L, Pedersen J, . Phase-II study on stereotactic radiotherapy of locally advanced pancreatic carcinoma. Radiother Oncol 2005;76:48–53.
- Schellenberg D, Goodman KA, Lee F, Chang S, Kuo T, Ford JM, . Gemcitabine chemotherapy and single-fraction stereotactic body radiotherapy for locally advanced pancreatic cancer. Int J Radiat Oncol Biol Phys 2008;72: 678–86.
- Koong AC, Le QT, Ho A, Fong B, Fisher G, Cho C, . Phase I study of stereotactic radiosurgery in patients locally advanced pancreatic cancer. Int J Radiat Oncol Biol Phys 2004;58:1017–21.
- Choi CW, Cho CK, Yoo SY, Kim MS, Yang KM, Yoo HJ, . Image-guided stereotactic body radiation therapy in patients with isolated para-aortic lymph node metastases from uterine cervical and corpus cancer. Int J Radiat Oncol Biol Phys 2009;74:147–53.
- Kim MS, Yoo SY, Cho CK, Yoo HJ, Yang KM, Kang JK, . Stereotactic body radiotherapy for isolated para-aortic lymph node recurrence after curative resection in gastric cancer. J Korean Med Sci 2009;24:488–92.
- Kim MS, Cho CK, Yang KM, Lee DH, Moon SM, Shin YJ. Stereotactic body radiotherapy for isolated paraaortic lymph node recurrence from colorectal cancer. World J Gastroenterol 2009;15:6091–5.
- Bignardi M, Navarria P, Mancosu P, Cozzi L, Fogliata A, Tozzi A, . Clinical outcome of hypofractionated stereotactic radiotherapy for abdominal lymph node metastases Int J Radiat Oncol Biol Phys 2010 (in press).
- Milano MT, Katz AW, Schell MC, Philip A, Okunieff P. Descriptive analysis of oligometastatic lesions treated with curative-intent stereotactic body radiotherapy. Int J Radiat Oncol Biol Phys 2008;72:1516–22.
- Matuszak MM, Yan D, Grills I, Martinez A. Clinical applications of volumetric modulated arc therapy. Int J Radiat Oncol Biol Phys 2010;77:608–16.
- Bignardi M, Cozzi L, Fogliata A, Lattuada P, Mancosu P, Navarria P, . Critical appraisal of volumetric modulated arc therapy in stereotactic body radiation therapy for metastases to abdominal lymph nodes. Int J Radiat Oncol Biol Phys 2009;75:1570–7.
- Cozzi L, Dinshaw KA, Shrivastava SK, Mahantshetty U, Engineer R, Deshpande DD, . A treatment planning study comparing volumetric arc modulation with RapidArc and fixed field IMRT for cervix uteri radiotherapy. Radiother Oncol 2008;89:180–91.
- Fogliata A, Clivio A, Nicolini G, Vanetti E, Cozzi L. Intensity modulation with photons for benign intracranial tumours. A planning comparison of volumetric single arc, helical arc and fixed gantry techniques. Radiother Oncol 2008;89:254–62.
- Palma D, Vollans E, James K, Nakano S, Moiseenko V, Shaffer R, . Volumetric modulated arc therapy for delivery of prostate radiotherapy. Comparison with intensity modulated radiotherapy and three-dimensional conformal radiotherapy. Int J Radiat Oncol Biol Phys 2008;72:996–1001.
- Weber DC, Peguret N, Dipasquale G, Cozzi L. Involved-node and involved-field volumetric modulated arc vs. fixed beam intensity-modulated radiotherapy for female patients with early-stage supra-diaphragmatic Hodgkin lymphoma: A comparative planning study. Int J Radiat Oncol Biol Phys 2009;75:1578–86.
- Wu QJ, Yoo S, Kirkpatrick JP, Thongphiew D, Yin FF. Volumetric arc intensity-modulated therapy for spine body radiotherapy: Comparison with static intensity-modulated treatment. Int J Radiat Oncol Biol Phys 2009;75:1596–604.
- Mancosu P, Navarria P, Bignardi M, Cozzi L, Fogliata A, Lattuada P, . Re-irradiation of metastatic spinal cord compression: A feasibility study by volumetric-modulated arc radiotherapy for in-field recurrence creating a dosimetric hole on the central canal. Radiother Oncol 2010;94:67–70.
- Ong CL, Palma D, Verbakel WF, Slotman BJ, Senan S. Treatment of large I-II lung tumors using stereotactic body radiotherapy (SBRT): Planning considerations and early toxicity. Radiother Oncol 2010;97:431–6.
- Kuijper IT, Dahele M, Senan S, Verbakel WF. Volumetric modulated arc therapy versus conventional intensity modulated radiation therapy for stereotactic spine radiotherapy: A planning study and early clinical data. Radiother Oncol 2010;94:224–8.
- Wysocka B, Kassam Z, Lockwood G, Brierley J, Dawson LA, Buckley CA, . Interfraction and respiratory organ motion during conformal radiotherapy in gastric cancer. Int J Radiat Oncol Biol Phys 2010;77:53–9.
- Gwynne S, Wills L, Joseph G, John G, Staffurth J, Hurt C, . Respiratory movement of upper abdominal organs and its effect on radiotherapy planning in pancreatic cancer. Clin Oncol 2009;21:713–9.
- Mori S, Hara R, Yanagi T, Sharp GC, Kumagai M, Asakura H, . Four-dimensional measurement of intrafractional respiratory motion of pancreatic tumors using a 256 multi-slice CT scanner. Radiother Oncol 2009;92:231–7.
- Kubas A, Mornex F, Merle P, d'Hombres A, Lorchel F, Chapet O. Irradiation of hepatocellular carcinoma: Impact of breathing on motions and variations of volume of the tumor, liver and upper abdominal organs. Cancer Radiother 2008;12:768–74.
- Kirilova A, Lockwood G, Choi P, Bana N, Haider MA, Brock KK, . Three-dimensional motion of liver tumors using cine-magnetic resonance imaging. Int J Radiat Oncol Biol Phys 2008;71:1189–95.
- Heinzerling JH, Anderson JF, Papiez L, Boike T, Chien S, Zhang G, . Four-dimensional computed tomography scan analysis of tumor and organ motion at varying levels of abdominal compression during stereotactic treatment of lung and liver. Int J Radiat Oncol Biol Phys 2010;70:1571–5.
- Sanguineti G, Little M, Endres EJ, Sormani MP, Parker BC, . Comparison of three strategies to delineate the bowel for whole pelvis IMRT of prostate cancer. Radiother Oncol 2008;88:95–101.
- Nicolini G, Vanetti E, Clivio A, Fogliata A, Korreman S, Bocanek J, . The GLAaS algorithm for portal dosimetry and quality assurance of RapidArc, an intensity modulated rotational therapy. Radiat Oncol 2008;3:24.
- Nicolini G, Fogliata A, Vanetti E, Clivio A, Cozzi L. GLAaS: An absolute dose calibration algorithm for an amorphous silicon portal imager. Applications to IMRT verifications. Med Phys 2006;33:2839–51.
- Low DA, Harms WB, Mutic S, Purdy JA. A technique for quantitative evaluation of dose distributions. Med Phys 1998; 25:656–61.
- International Commission on Radiation Units and Measurements. ICRU report 62: Prescribing, recording and reporting Intensity Modulated Photon Beam Therapy (IMRT) (ICRU report 83). Washington, DC: International Commission on Radiation Units and Measurements; 2010.
- National Cancer Institute. Cancer Therapy Evaluation Program. Common Terminology Criteria for Adverse Events. Version 3.0. DCTD, NCI, NIH, NHHS. March 31, 2003. Available from: http://cteo.cancer.gov
- Miller AB, Hoogstraten B, Staquet M, Winkler A. Reporting results of cancer treatment. Cancer 1981;47:207–14.
- Jereczek-Fossa BA, Fariselli L, Beltramo G, Catalano G, Serafini F, Garibaldi C, . Linac-based or robotic image-guided stereotactic radiotherapy for isolated lymph node recurrent prostate cancer. Radiother Oncol 2009;93: 14–7.
- Ong C, Verbakel W, Cuijpers J, Slotman B, Senan S. Dosimetric impact of interplay effect on rapidarc lung stereotactic treatment delivery. Int J Radiat Oncol Biol Phys 2010; doi:10.1010/j.ijrobp.2010.02.059.
- Court L. Wagar M, Berbeco R, Reisner A, Winey B, Shofield D, . Evaluation of the interplay effect when using RapidArc to treat targets moving in the craniocaudal or right and left directions. Med Phys 2010;37:4–11.