Abstract
Background. A direct association between radiotherapy dose, side-effects and secondary cancers has been described in patients with seminoma. A treatment planning study was performed in order to compare computed tomography-based traditional radiotherapy (CT-tRT) versus bone marrow-sparing intensity-modulated radiation therapy (BMS-IMRT) in patients with Stage I seminoma. Material and methods. We optimized in 10 patients a CT-tRT and a BMS-IMRT treatment plan to deliver 20 Gy to the para-aortic nodes. CT-tRT and IMRT consisted of anteroposterior-posterioranterior parallel-opposed and seven non-opposed coplanar fields using 16 and 6-MV photon energies, respectively. Dose-Volume Histograms for clinical target volume (CTV), planning target volume (PTV) and organs at risk (OARs) were compared for both techniques using Wilcoxon matched-pair signed rank-test. Results. Dmean to CTV and PTV were similar for both techniques, even if CT-tRT showed a slightly improved target coverage in terms of PTV-D95% (19.7 vs. 19.5 Gy, p = 0.005) and PTV-V95% (100 vs. 99.7%, p = 0.011) compared to BMS-IMRT. BMS-IMRT resulted in a significant reduction (5.2 Gy, p = 0.005) in the Dmean to the active bone marrow (ABM). The V100% and V75% of the OARs were reduced with BMS-IMRT by: ABM-V100% = 51.7% and ABM-V75% = 42.3%; bowel-V100% = 15.7% and bowel-V75% = 16.8%; stomach-V100% = 22% and stomach-V75% = 27.7%; pancreas-V100% = 37.1% and pancreas-V75% = 35.9% (p = 0.005 for all variables). Conclusions. BMS-IMRT reduces markedly the dose to the OARs compared to CT-tRT. This should translate into a reduction in acute and long-term toxicity, as well as into the risk of secondary solid and hematological cancers.
Prophylactic radiotherapy (RT) following orchidectomy together with adjuvant carboplatin or surveillance represents one of the possible approaches used in the management of patients with Stage I testicular seminoma [Citation1]. Traditional adjuvant RT using para-aortic field at a dose of 20 Gy in 10 fractions over two weeks confers a relapse-free survival rate of around 97% and a disease-specific survival of almost 100% [Citation2,Citation3].
Although highly effective, this treatment is associated with some acute gastrointestinal side-effects including nausea, vomiting, and decreased performance status during and after treatment [Citation4]. Moreover, it puts all patients at risk of long-term treatment related morbidity. Late morbidity after infradiaphragmatic irradiation is most commonly related to the gastrointestinal tract, including an increased risk to develop peptic ulcer and small bowel obstruction, and at a lesser rate, to other organs such as liver or kidneys [Citation5–7]. There is also evidence of an increased risk of new treatment-induced primary non-germ cell cancers including solid [Citation8–11] and hematological malignancies [Citation12].
Every effort has to be undertaken to optimize the RT technique to reduce acute and long-term complications in these young patients with an excellent prognosis. The best treatment should offer the most effective cure rates while minimizing the risk of side-effects. New technologies such as intensity-modulated RT (IMRT) may currently allow to “sculpt” the dose around the target volume thus reducing the dose to the surrounding healthy tissues.
A direct association between the delivered RT dose, the irradiated volumes and the risk to develop late side-effects and secondary cancer has been described in testicular cancer patients [Citation12–14]. Seminoma affects largely a younger population and secondary cancers represent a leading cause of death in survivors of seminoma [Citation15,Citation16]. A reduction of the dose delivered to active bone marrow (ABM) and to other organs at risk (OARs) achieved with IMRT over traditional RT techniques might potentially translate in a reduction in the risk of acute and late RT-induced side-effects, as well as of secondary malignancies.
The aim of the present study was to assess the potential dosimetric and clinical advantages of adjuvant para-aortic bone-marrow sparing IMRT (BMS-IMRT) over computed tomography-based traditional RT (CT-tRT) in the treatment of patients with testicular Stage I seminoma.
Material and methods
The CT datasets from 10 patients all with Stage I testicular seminoma were included in the study. Before adjuvant para-aortic RT, all patients underwent radical orchidectomy for four right-sided and six left-sided testicular tumors. The American Joint Committee on Cancer (AJCC) pathological staging was pT1 and pT2 in eight and two patients, respectively. All but one patients presented with one (five patients) or two (four patients) of the following pathological risk factors for relapse in patients managed with surveillance only: tumor size > 4 cm, rete testis involvement and/or lymphovascular space invasion [Citation17].
Patients were scanned in treatment position (i.e. lying supine, with both arms above the head) from the lower chest to the obturator foramina using a 3-mm slice thickness without intravenous contrast injection using a Philips Brilliance Big Bore CT (Amsterdam, the Netherlands) multi-slice CT scanner. Treatment plans were implemented on an Eclipse® treatment-planning system (TPS version 8.6.10; Varian Medical System, Palo Alto, CA, USA). The clinical target volume (CTV) encompasses the inter-aortocaval, preaortic and para-aortic lymph node (PALN) regions. It was based on vascular anatomy, including the aorta, the inferior vena cava and the ipsilateral renal vein [Citation3,Citation18,Citation19]. Upper and inferior limits for CTV delineation were seven CT slices (i.e. 2.1 cm) below and above the superior aspect of T11 and the bottom of L5, respectively. To account for treatment set-up uncertainties and organ motion, the planning target volume (PTV) was defined as the CTV with a 3D anisotropic margin of 10 mm in all directions except posteriorly, where a margin of 5 mm was used. The OARs included the entire liver, combined kidneys, stomach, pancreas, bowel, spinal cord and ABM. The bowel was defined as the entire bowel loops within the peritoneal cavity, including individual loops and excluding the rectum. ABM in the PALN region was defined contouring all vertebral bodies within seven CT slices below and above the PTV. The ribs and pelvic bones were also included. In order to exclude the cortex, about 1 mm from the bone wall was subtracted from the vertebral body [Citation20,Citation21]. The percentage of total-body bone marrow activity estimated in adult cancer patients by molecular imaging is 16.6% and 25.3% for the lumbar spine and the pelvis, respectively [Citation22]. The spinal cord was contoured from seven CT slices above the PTV until the inferior border of the vertebral body L2. No planning organ-at-risk volume was added at OARs.
For non target-tissue, the integral dose (DoseInt) was defined as the integral of the absorbed dose extended to over all voxels excluding those within the target volume (DoseInt dimensions are Gy*cm3*105).
The prescribed dose to the PTV was 20 Gy in 10 fractions of 2 Gy [Citation3]. As recommended by the International Commission on Radiation Units and Measurements (ICRU 62) [Citation23], PTV coverage was specified to fall between a minimum (Dmin) and a maximum dose (Dmax) equal to 95% and 107% of prescribed doses, respectively. The goal was to cover 100% of the PTV with ≥ 95% of the prescribed dose (i.e. 19 Gy).
CT-tRT treatment planning
CT-tRT consisted of two anteroposterior-posteroanterior (AP-PA) parallel-opposed fields encompassing the entire PTV using 16-MV photons. The weights of the individual fields were optimized to achieve dose uniformity in the PTV. To obtain the desired PTV coverage, a 1- and 0.7-cm margin was normally used around the PTV in the supero-inferior and lateral directions, respectively. The traditional para-aortic treatment field extended from the T10-11 disk space to the L5-S1 disk space using the criteria from the Medical Research Council (MRC) phase III study [Citation2].
BMS-IMRT treatment planning
BMS-IMRT field arrangement consisted of seven non-opposed coplanar fields arranged at 0, 45, 85, 145, 215, 275, 315 degrees, respectively. The collimator angle was set at 0°. A dynamic sliding-windows IMRT technique using 6-MV photons was used. For BMS-IMRT inverse planning priority was generally given to the CTV-PTV coverage as well as to the dose delivered to the ABM over the other OARs. shows the OARs dose constraints used for the BMS-IMRT plans. For ABM, the optimization input parameter was to achieve a mean dose (Dmean) inferior to 7.5 Gy. A dose of 7.5 Gy or more to ABM was associated with a threefold increased risk of leukemia in a case-control study of leukemia risk in seminoma patients [Citation12]. For both kidneys, a Dmean of less than 6.9 Gy was used as the dose constraint for inverse planning. This was the Dmean value calculated by Martin et al. for both kidneys using a CT-tRT planning in patients with Stage I seminoma [Citation19].
Table I. Target volume and organs at risk dose constraints used in bone marrow-sparing intensity-modulated radiotherapy treatment planning.
Tools for DVH analysis
Quantitative evaluation of plans was performed with cumulative Dose-Volume Histograms (DVH). Minimum (Dmin), Dmean and maximum (Dmax) dose were analyzed for the CTV and PTV. PTV D50% and D95% were also calculated, where Dn% is the minimal dose delivered to the percentage of volume of the target volume. PTV V100% (volume receiving at least 100% of the prescribed dose, i.e. 20 Gy), V95% (i.e. 19 Gy), and V93% (i.e. 18.6 Gy) were compared between both techniques. Additionally, the number of monitor units (MU) required to deliver 2 Gy fraction and the DoseInt to healthy tissues was calculated for each plan. Doses to OARs obtained with BMS-IMRT and CT-tRT were compared for each case.
Average cumulative DVH for PTV and OARs were built from the individual DVHs to visualize the difference between both treatment techniques. These DVHs were obtained by averaging the corresponding volumes over the whole patient's cohort for each dose bin of 0.05 Gy.
Statistical analysis
Comparison between BMS-IMRT and CT-tRT was done using the Wilcoxon matched-pair signed rank-test with a threshold for statistical significance of p ≤ 0.05. All statistical tests were two-sided and were performed using SPSS 17.0 statistic software package (SPSS Inc. Chicago, IL, USA).
Results
Target volume coverage
CTV and PTV dose coverage were comparable between both techniques. No difference in the mean delivered dose to CTV and PTV were observed between CT-tRT and BMS-IMRT. CT-tRT showed a slightly improved target coverage in terms of PTV D95% (PTV D95% difference [Δ PTV D95%] = 0.2 Gy, p = 0.005) and PTV V95% (Δ PTV V95% = 0.3%, p = 0.011) as compared with BMS-IMRT. The mean number of MU per fraction was significantly reduced by a factor of five for CT-tRT when compared to BMS-IMRT planning (236 vs. 1180 MU, respectively). reports a comparison of the dosimetric results of the CTV and PTV between BMS-IMRT and CT-tRT. Dose distributions are shown from one representative case in for axial and sagittal views. shows the average DVH plots for the PTV and OARs.
Figure 1. Patient with Stage I testicular seminoma: color wash bone-marrow-sparing intensity-modulated radiotherapy (BMS-IMRT) (a, c) and computed tomography-based traditional radiotherapy (CT-tRT) (b, d) isodose distributions (95% isodose line) on axial (a, b) and sagittal (c, d) views.
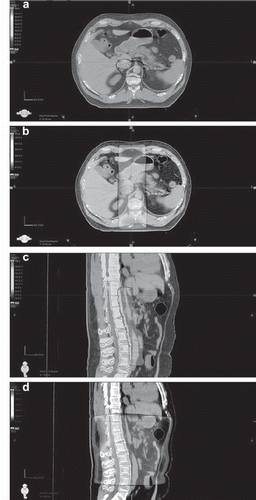
Figure 2. Mean dose-volume histograms for planning target volume (PTV) and organs at risk (OARs) for bone-marrow-sparing intensity-modulated radiotherapy (BMS-IMRT) and computed tomography-based traditional radiotherapy (CT-tRT).
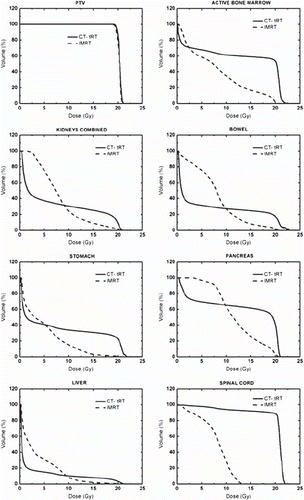
Table II. Comparison of dosimetric parameters for clinical target volume (CTV) and planning target volume (PTV). Results in mean ± SD (n = 10).
Dose distribution to organs at risk and non-target tissues
Mean differences in dose to the OARs and non- target tissues between the two treatment's modalities are presented in .
Table III. Comparison of dosimetric parameters for organs at risk and non-target tissues. Results in mean ± SD (n = 10).
Active bone marrow. Planning dose constraint used for inverse planning (i.e. 7.5 Gy) was only partially respected by BMS-IMRT. The mean dose for all patients was 7.9 ± 0.05 Gy. However, compared with CT-tRT the use of BMS-IMRT resulted in a significant reduction in the mean delivered dose to the ABM (Δ Dmean = 5.2 Gy, p = 0.005). A significant decrease into the dose delivered to ABM was also observed with BMS-IMRT in the mid and high-dose level (10–20 Gy). This reduction in favor of BMS-IMRT was estimated by a factor of 31, 3.5 and 2 for the V100%, V75% and V50%, respectively.
Kidneys. All but one planning dose constraint (Dmean) with BMS-IMRT were generally respected in a majority of patients. With BMS-IMRT, Dmean was higher than the planning goal in all patients (Dmean = 8.0 ± 0.8 Gy), whereas V100% exceeded the dose constraint only in four cases (range, 1.2–1.5%). Dmean as well as the dose delivered to 50% of the volume (D50%) were lower using a CT-tRT technique (Δ Dmean = 1.2 Gy, p = 0.007 and Δ D50% = 5.2 Gy, p = 0.005). On the other hand, volumes irradiated to medium and to high doses (V50% – V100%) were significantly reduced when BMS-IMRT was used (Δ V100% = 7.7%, p = 0.005; ΔV75% = 16.3%, p = 0.005 and Δ V50% = 6.5%, p = 0.038).
Gastrointestinal organs. Planning dose constraints for bowel, stomach, pancreas and liver were met by IMRT-BMS in all patients. For all these organs, the percentage of volume irradiated to high-dose (V100% and V75%) was significantly reduced using BMS-IMRT as compared to a CT-tRT technique (p = 0.005–0.018). Dmean for liver and bowel were lower with CT-tRT, but this difference was only 1 Gy and 1.9 Gy, respectively. A 2.6 Gy difference in the Dmean delivered to the stomach was observed using BMS-IMRT (5 vs. 7.6 Gy, p = 0.011). No difference between the two techniques was observed in the Dmean of pancreas, but BMS-IMRT was able to reduce the dose delivered to 50% of this organ when compared to CT-tRT by 6.1 Gy (10.2 Gy vs. 16.3 Gy, p = 0.017).
Spinal cord. As expected, when using CT-tRT maximal point doses delivered to the spinal cord were approximately higher by a factor 1.6. The mean estimated Dmax of the spinal cord with CT-tRT was 107.4 ± 1.7%, which was higher than the Dmax computed on the PTV (105.8% ± 0.9) with the same technique. On the other hand, BMS-IMRT allowed a significant reduction in the Dmean and the D50% of 11.8 Gy and 12.5 Gy, respectively.
Non-target tissue. No planning dose constraints were implemented. The DoseInt to healthy tissues was significantly decreased with BMS-IMRT when compared to CT-tRT (p = 0.028).
Discussion
The acute and long-term toxicity of non-conformal infradiaphragmatic RT for Stage I seminoma are directly related to the radiation dose [Citation3,Citation24]. In the MRC randomized trial [Citation3], acute morbidity was significantly reduced by delivering 20 Gy instead of 30 Gy. However, 18.4% of the patients in the RT low-dose arm experienced Grade 3–4 nausea and vomiting despite the use of 5-hydroxtryptamnie-3 serotonin antagonist, and 16.7% of the patients reported dyspepsia during the RT course. Moreover, treatment-related lethargy and inability to carry out normal activities was present in 5% and 28% of the patients treated with 20 Gy. It should be noted that randomized trials were based on a two-dimensional bony landmark-based RT [Citation2,Citation3]. Today, CT-based RT planning is considered superior in treating Stage I seminoma. Indeed, as demonstrated by Martin et al., traditional non-conformal para-aortic fields based on bony landmarks may deliver suboptimal dose to an anatomically defined PTV, increasing the risk of underdosage and geographical miss as compared to CT-based treatment plans [Citation19]. On the other hand, a CT-tRT requires wider fields than the traditional plans, translating to a potentially greater rate of acute and late side-effects [Citation19]. In this present study, we analyzed the potential dosimetric advantages of BMS-IMRT, the next technical evolution in the RT delivery over conformal RT. We found a significant reduction using BMS-IMRT in the volume of stomach and bowel irradiated to high-dose (bowel Δ V100% = 15.5%, p = 0.005, stomach Δ V100% = 22%, p = 0.005). We expect therefore a reduction in the rate of acute and late toxicity when compared to CT-tRT. However, the translation of this dosimetric advantage into a clinical benefit requires further clinical investigation.
One of the main arguments against the use of RT in the adjuvant setting of early stage seminoma is the increased risk of secondary malignancies associated with this treatment. Travis et al. investigated the occurrence of second cancers among over 40 000 men treated for testicular cancer between 1943 and 2001 [Citation25]. Patients treated with adjuvant RT presented a statistically increased risk of solid cancer when compared to the general population, with an estimated relative risk (RR) of 2. The RR was even higher for infradiaphragmatic organs in or close to the RT fields: cancer of the stomach (RR = 4.1), pancreas (RR = 3.8) and bladder (RR = 2.7). Even if the use of para-aortic RT could completely spare the bladder and therefore reduce the risk of radiation-induced malignancies at this level, large volumes of stomach and pancreas are inevitably irradiated at full-dose when an AP-PA technique is used. Given the important dose-response relationship for secondary cancers [Citation26–28], we believe that a reduction in the dose delivered to these structures using BMS-IMRT may potentially translate into a decreased risk of new malignancies. This dose-related relationship in the induction of secondary cancers was confirmed by the organ equivalent dose models developed in patients treated with para-aortic fields for Stage I testicular seminoma by Zwahlen et al. [Citation13]. This was true, independently from the dose-response model used, especially for a delivered dose to OARs of less than 15 Gy. Below this dose, the relationship between the delivered dose and the secondary cancer risk is stronger.
A case control study of the leukemia risk in a cohort of 18 567 patients treated for testicular cancer between 1970 and 1993 showed that RT (Dmean to ABM of 12.6 Gy) was associated with a threefold elevated risk of leukemia [Citation12]. This risk increased significantly with an increased dose to the ABM, with an estimated RR of leukemia for RT doses ≥ 15 Gy of 7.8 (95% CI, 1.1–79). With the high conformality in the dose distribution in our study achieved with BMS-IMRT, the mean dose to ABM was reduced by 5.2 Gy (26% of the total delivered dose, ABM Dmean = 7.9 Gy). Moreover, it is noteworthy that Grade 1 and 2 leukopenia occurred in 12.3% and 1.8% of the patients treated at 20 Gy to the para-aortic field in the MRC randomized trial [Citation3]. These results show that BMS-IMRT is expected to reduce the risk of leukemia as well as hematological toxicity [Citation29,Citation30].
The potential advantage of BMS-IMRT to decrease the toxicity for OARs within the treatment field may be counter-balanced by the use of five times more MU compared to CT-tRT. Scatter dose, number of MU and volume of normal tissues receiving low-dose radiation have been linked to an increased risk of secondary malignancies after RT [Citation31]. Consequently, the mean dose delivered may not be a useful criterion for judging the risk of secondary malignancies. This risk may increase primarily with MU needed to deliver the prescribed dose. Nevertheless, it remains to be shown whether an increase in MU will translate into a higher incidence of secondary malignancies. No studies with long follow-up are available to reliably estimate the radiation induced risk of secondary cancers with IMRT. Moreover, new RT treatment techniques, such as volumetric single arc RT, permit a significant reduction (by a factor of about 2 into MU/Gy) in scatter and linear accelerator head leakage dose when compared to conventional IMRT [Citation32,Citation33].
An other important issue is the dose delivered to the contralateral testis. Gonadal shielding may reduce the severity and duration of oligospermia, as well as minimize dose-related genetic abnormalities in patients undergoing RT limited to the para-aortic region [Citation34]. In our study, we were unable to calculate retrospectively the dose to the testes for BMS-IMRT. Moreover, our ability to estimate the scatter dose and the volume of normal tissues receiving low-dose radiation was limited because the planning CT images extended only little over the treatment fields. This restricted our ability to predict the risk of radio-induced malignancies to organs outside the para-aortic region. It should be noted that the increased number of MU needed for BMS-IMRT may be counter-balanced by a higher scatter dose to normal tissues observed with the higher photon energies needed for CT-tRT [Citation35]. The DoseInt to healthy tissues was also significantly reduced using BMS-IMRT compared to CT-tRT. We believe that standard cerrobend blocks with 1.5 cm thickness are sufficient in BMS-IMRT to reduce by more than 50% the scattered dose to the contralateral testis [Citation34,Citation36].
In summary, BMS-IMRT represents a promising treatment approach for Stage I seminoma patients. We believe that the marked OARs dose-sparing effect will reduce acute and long-term toxicity, as well as the risk of secondary solid and hematological cancers compared to conventional techniques. However, additional investigations are warranted to assess the short- and long-term clinical benefit of this technique.
Acknowledgments
Dr Thomas Zilli was supported in part by a Fellowship grant from Sanofi-Aventis. The authors report no conflicts of interest. The authors alone are responsible for the content and writing of the paper.
References
- de Wit R, Fizazi K. Controversies in the management of clinical stage I testis cancer. J Clin Oncol 2006;24: 5482–92.
- Fossa SD, Horwich A, Russell JM, Roberts JT, Cullen MH, Hodson NJ, . Optimal planning target volume for stage I testicular seminoma: A Medical Research Council randomized trial. Medical Research Council Testicular Tumor Working Group. J Clin Oncol 1999;17:1146.
- Jones WG, Fossa SD, Mead GM, Roberts JT, Sokal M, Horwich A, . Randomized trial of 30 versus 20 Gy in the adjuvant treatment of stage I Testicular Seminoma: A report on Medical Research Council Trial TE18, European Organisation for the Research and Treatment of Cancer Trial 30942 (ISRCTN18525328). J Clin Oncol 2005;23:1200–8.
- Aass N, Fossa SD, Host H. Acute and subacute side effects due to infra-diaphragmatic radiotherapy for testicular cancer: A prospective study. Int J Radiat Oncol Biol Phys 1992;22:1057–64.
- Coia LR, Hanks GE. Complications from large field intermediate dose infradiaphragmatic radiation: An analysis of the patterns of care outcome studies for Hodgkin's disease and seminoma. Int J Radiat Oncol Biol Phys 1988;15:29–35.
- Fossa SD, Aass N, Kaalhus O. Radiotherapy for testicular seminoma stage I: Treatment results and long-term post-irradiation morbidity in 365 patients. Int J Radiat Oncol Biol Phys 1989;16:383–8.
- Hamilton CR, Horwich A, Bliss JM, Peckham MJ. Gastrointestinal morbidity of adjuvant radiotherapy in stage I malignant teratoma of the testis. Radiother Oncol 1987;10: 85–90.
- Bokemeyer C, Schmoll HJ. Secondary neoplasms following treatment of malignant germ cell tumors. J Clin Oncol 1993;11:1703–9.
- Fossa SD, Langmark F, Aass N, Andersen A, Lothe R, Borresen AL. Second non-germ cell malignancies after radiotherapy of testicular cancer with or without chemotherapy. Br J Cancer 1990;61:639–43.
- Travis LB, Curtis RE, Storm H, Hall P, Holowaty E, Van Leeuwen FE, . Risk of second malignant neoplasms among long-term survivors of testicular cancer. J Natl Cancer Inst 1997;89:1429–39.
- van Leeuwen FE, Stiggelbout AM, van den Belt-Dusebout AW, Noyon R, Eliel MR, van Kerkhoff EH, . Second cancer risk following testicular cancer: A follow-up study of 1,909 patients. J Clin Oncol 1993;11:415–24.
- Travis LB, Andersson M, Gospodarowicz M, Van Leeuwen FE, Bergfeldt K, Lynch CF, . Treatment-associated leukemia following testicular cancer. J Natl Cancer Inst 2000;92:1165–71.
- Zwahlen DR, Martin JM, Millar JL, Schneider U. Effect of radiotherapy volume and dose on secondary cancer risk in stage I testicular seminoma. Int J Radiat Oncol Biol Phys 2008;70:853–8.
- Majewski W, Majewski S, Maciejewski A, Kolosza Z, Tarnawski R. Adverse effects after radiotherapy for early stage (I,IIa,IIb) seminoma. Radiother Oncol 2005;76:257–63.
- Schairer C, Hisada M, Chen BE, Brown LM, Howard R, Fossa SD, . Comparative mortality for 621 second cancers in 29356 testicular cancer survivors and 12420 matched first cancers. J Natl Cancer Inst 2007;99:1248–56.
- Zagars GK, Ballo MT, Lee AK, Strom SS. Mortality after cure of testicular seminoma. J Clin Oncol 2004;22:640–7.
- Warde P, Specht L, Horwich A, Oliver T, Panzarella T, Gospodarowicz M, . Prognostic factors for relapse in stage I seminoma managed by surveillance: A pooled analysis. J Clin Oncol 2002;20:4448–52.
- Martinez-Monge R, Fernandes PS, Gupta N, Gahbauer R. Cross-sectional nodal atlas: A tool for the definition of clinical target volumes in three-dimensional radiation therapy planning. Radiology 1999;211:815–28.
- Martin JM, Joon DL, Ng N, Grace M, Gelderen DV, Lawlor M, . Towards individualised radiotherapy for Stage I seminoma. Radiother Oncol 2005;76:251–6.
- Ahmed RS, Kim RY, Duan J, Meleth S, De Los Santos JF, Fiveash JB. IMRT dose escalation for positive para-aortic lymph nodes in patients with locally advanced cervical cancer while reducing dose to bone marrow and other organs at risk. Int J Radiat Oncol Biol Phys 2004;60:505–12.
- Argiris A, Maris T, Vlahos L. T2 relaxation times of irradiated vertebral bone marrow in patients with seminoma. Magn Reson Imaging 1997;15:335–40.
- Hayman JA, Callahan JW, Herschtal A, Everitt S, Binns DS, Hicks RJ, . Distribution of proliferating bone marrow in adult cancer patients determined using FLT-PET imaging. Int J Radiat Oncol Biol Phys 2010.
- Chavaudra J, Bridier A. [Definition of volumes in external radiotherapy: ICRU reports 50 and 62]. Cancer Radiother 2001;5:472–8.
- Hamilton C, Horwich A, Easton D, Peckham MJ. Radiotherapy for stage I seminoma testis: Results of treatment and complications. Radiother Oncol 1986;6:115–20.
- Travis LB, Fossa SD, Schonfeld SJ, McMaster ML, Lynch CF, Storm H, . Second cancers among 40,576 testicular cancer patients: Focus on long-term survivors. J Natl Cancer Inst 2005;97:1354–65.
- Boice JD, Jr., Engholm G, Kleinerman RA, Blettner M, Stovall M, Lisco H, . Radiation dose and second cancer risk in patients treated for cancer of the cervix. Radiat Res 1988;116:3–55.
- Inskip PD, Stovall M, Flannery JT. Lung cancer risk and radiation dose among women treated for breast cancer. J Natl Cancer Inst 1994;86:983–8.
- Travis LB, Hill D, Dores GM, Gospodarowicz M, van Leeuwen FE, Holowaty E, . Cumulative absolute breast cancer risk for young women treated for Hodgkin lymphoma. J Natl Cancer Inst 2005;97:1428–37.
- Albuquerque K, Giangreco D, Morrison C, Siddiqui M, Sinacore J, Potkul R, . Radiation-related predictors of hematologic toxicity after concurrent chemoradiation for cervical cancer and implications for bone marrow-sparing pelvic IMRT. Int J Radiat Oncol Biol Phys 2010.
- Mell LK, Tiryaki H, Ahn KH, Mundt AJ, Roeske JC, Aydogan B. Dosimetric comparison of bone marrow-sparing intensity-modulated radiotherapy versus conventional techniques for treatment of cervical cancer. Int J Radiat Oncol Biol Phys 2008;71:1504–10.
- Hall EJ, Wuu CS. Radiation-induced second cancers: The impact of 3D-CRT and IMRT. Int J Radiat Oncol Biol Phys 2003;56:83–8.
- Cozzi L, Dinshaw KA, Shrivastava SK, Mahantshetty U, Engineer R, Deshpande DD, . A treatment planning study comparing volumetric arc modulation with RapidArc and fixed field IMRT for cervix uteri radiotherapy. Radiother Oncol 2008;89:180–91.
- Shaffer R, Morris WJ, Moiseenko V, Welsh M, Crumley C, Nakano S, . Volumetric modulated Arc therapy and conventional intensity-modulated radiotherapy for simultaneous maximal intraprostatic boost: A planning comparison study. Clin Oncol (R Coll Radiol) 2009; 21:401–7.
- Bieri S, Rouzaud M, Miralbell R. Seminoma of the testis: Is scrotal shielding necessary when radiotherapy is limited to the para-aortic nodes? Radiother Oncol 1999;50:349–53.
- Howell RM, Hertel NE, Wang Z, Hutchinson J, Fullerton GD. Calculation of effective dose from measurements of secondary neutron spectra and scattered photon dose from dynamic MLC IMRT for 6 MV, 15 MV, and 18 MV beam energies. Med Phys 2006;33:360–8.
- Joos H, Sedlmayer F, Gomahr A, Rahim HB, Frick J, Kogelnik HD, . Endocrine profiles after radiotherapy in stage I seminoma: Impact of two different radiation treatment modalities. Radiother Oncol 1997;43:159–62.