Abstract
Background. The on-going development of both intensity-modulated radiotherapy (IMRT), including the more recent intensity-modulated arc therapy, as well as particle beam therapy, has created a clear need for accurate verification of dose distributions in three dimensions (3D). Presage™ is a new 3D dosimetry material that exhibits a radiochromic response when exposed to ionizing radiation. In this study we have 1) developed an improved optical set-up for measurements of changes in OD of Presage™ point dosimeters, 2) investigated the dose response of Presage™ for photons and carbon ions in the therapy range, 3) investigated the dose response of Presage™ for photons in the kGy range and 4) investigated the fading (i.e. bleaching) of Presage™ postirradiation. Materials and methods. Presage™ was examined in 1 × 1 × 4.5 cm3 optical cuvettes; a cuvette holder assured accurate repositioning, and the optical setup included a reference detector to take into account laser intensity fluctuations. The cuvettes were measured pre- and postirradiation for a two week period. Results. A linear response was observed between dose and optical response between 0 Gy and 100 Gy for γ-radiation from Co-60 and for carbon ions (both plateau and SOBP) from 0 to 20 Gy. The dosimeter was found to have a saturation dose of approximately 100 Gy for photons. A linear energy transfer (LET) effect was not observed in the dose response of different LET radiation. The postirradiation change in optical fading was found to be 0.5% ΔOD/day. Conclusions. Our study shows that Presage™ remains a dosimeter of interest for radiation therapy with other particles as well as photons in the therapy dose range.
The development of intensity-modulated radiotherapy (IMRT) and its successor intensity-modulated arc therapy have demonstrated a need for a dosimeter that accurately and conveniently can measure dose distributions in three dimensions (3D) to ensure treatment quality [Citation1–3]. The same requirements will apply in the on-going introduction of spot-scanned particle beam therapy where also variations in particle range contribute to the uncertainties in the dose distributions.
Gel dosimetry has shown promising properties for performing 3D dose measurements. In 3D gel dosimetry a macroscopic volume of radiation-sensitive gel is read-out by either MRI, x-ray CT or optical CT [Citation4–11]. Fricke and polymer gels are the two main types of gels reported in the literature [Citation12,Citation13]. The ferrous sulphate (Fricke) solution has been used as the basis for dosimetry for almost 50 years [Citation14]. When Gore in 1984 combined the system with magnetic resonance imaging 3D radiation dosimetry was made possible [Citation15]. Fricke gels have high reproducibility but suffer from diffusion problems [Citation16]. Polymer gels were introduced by Maryanski et al. [Citation17] a decade later. Polymer gels have a high sensitivity to radiation but suffer from light scattering artefacts. The preparation is more difficult as the dosimeter has to be manufactured in an oxygen-free environment due to the nature of their free radical chemistry [Citation18]. Still, polymer gels that can be manufactured in the presence of normal levels of oxygen (normoxic) have been suggested [Citation19].
Presage™ is a recently introduced 3D dosimetry material consisting of an optically clear solid-state polyurethane matrix, containing a leucomalachite dye that exhibits a radiochromic response when exposed to ionizing radiation. Presage™ is insensitive to atmospheric oxygen and unlike Fricke and polymer gels, it does not need an external container. These attractive features make it a dosimeter of significant interest [Citation20,Citation21]. The performance of Presage™ has been studied for photons in the therapy dose range, from simple coplanar fields [Citation22,Citation23], to complex IMRT treatments [Citation24]. For particle beams, little is at present known about the dose response of Presage™. Amorphous track models for particle dosimetry describe how high track core doses of several kGy can cause detector under-response due to local saturation of the dosimeter [Citation25–28], in line with a recently published preliminary analysis using protons [Citation29].
In this study we have investigated the dose response relations of the Presage™ dosimeter for both photons and carbon ions. The photon experiments included both dose levels in the therapy range as well in the kGy range, the latter also assumed relevant for the response of the dosimeter for particle beams. To explore the LET-dependency of Presage™, we also performed carbon ion irradiations both in the plateau and at the Bragg Peak.
Material and methods
Photon experiments
For the photon experiments in the therapy range Presage™ detectors in the shape of cuvettes were placed in a water phantom in the plane of the isocenter at a depth of 1.5 cm (). A Co-60 source (a Gammatron) with a field size of 30 × 30 cm2 was used for these experiments. The water level was adjusted to a source-to-surface distance (SSD) of 78.5 cm to achieve charged-particle equilibrium in the Presage™ detectors. The beam entered vertically from the top. The deposited dose was measured in the isocenter with a waterproof farmer-type ionization chamber with its axis perpendicular to the beam ().
Figure 1. Co-60 irradiation of Presage™ detectors. The samples of Presage™ mounted for irradiation with γ-photons from a Co-60 source with a farmer-type ionization chamber.
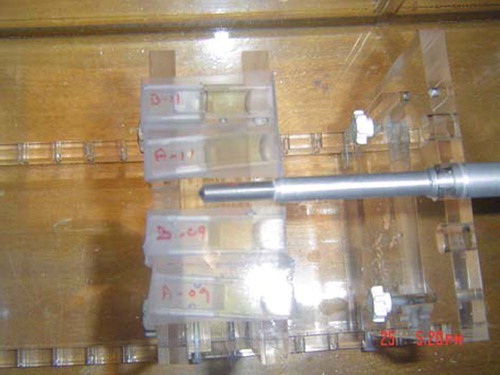
The dose response of Presage™ in the kGy dose range was investigated through collaboration with Risoe National Laboratory for Sustainable Energy. Cuvettes were irradiated to dose levels from 0 to 10 kGy with a dose accuracy of 3% (, ). Two different gamma cells were used, with dose rates of 10.7 Gy/min and 92.6 Gy/min. To investigate dose-rate effects, two sets of cuvettes were irradiated with 100 Gy and 200 Gy at both dose rates. The traceability to the national standards at National Physical Laboratory, UK, of the Cobalt-60 irradiation was ensured through the accreditation of the Risoe High Dose Reference Laboratory.
Table I. Overview of irradiated Presage™ samples. An overview of the number of cuvettes irradiated with different particles and doses. The table also includes the number of “blanks” that were used to estimate the background throughout each experiment. *Eight cuvettes were irradiated in the dose range from 50 to 10 kGy. The actual doses can be seen in . **From the series of irradiations performed at HIT.
Carbon ion experiments
The carbon ion irradiations performed in this study consisted of two separate series of experiments. The first series compared mono-energetic carbon ions to γ-radiation from the Gammatron. The photon irradiation was performed at the German Cancer Research Center (DKFZ), Heidelberg, Germany. Twenty two cuvettes were irradiated with carbon ions and γ-photons to doses in the range 1–10 Gy (). The samples irradiated with mono-energetic 400 MeV/u carbon ions in the plateau were placed in a PTW motorized water phantom. The beam entered the phantom horizontally from the side. Further details of these experiments performed at Gesellschaft für Schwerionenforschung (GSI), Darmstadt, Germany are outlined in .
The second series compared carbon ions in the plateau to the Spread Out Bragg Peak (SOBP) and was performed at the Heidelberg Ion Therapy (HIT) centre, Heidelberg, Germany. This series of experiments was performed by constructing a slab phantom around the detector. A water equivalent material was used and the beam entered the room horizontally. An average energy of 400 MeV/u was setup for the irradiation in the plateau and the cuvettes were irradiated with 10 Gy and 20 Gy (). The SOBP was established by continuously changing the energy of the beam from 213 to 270 MeV/u. As the Presage™ samples irradiated from 5 to 20 Gy were positioned in the beginning of the SOBP, the LET dependent effects were expected to be smaller. The traceability to the national standards at PTB in Braunschweig, Germany, of the carbon ion irradiations was ensured through calibration protocols using dosimeters from PTW Freiburg. The Presage™ dosimeters were pre-scanned before irradiation and postscanned after irradiation as mentioned below. In both experiments the dosimeters were placed at a depth sufficient for charged-particle equilibrium.
Fading experiments
The postirradiation fading of the Presage™ dosimeters was studied over a period of two weeks. Approximately every other day the transmission of the irradiated samples was measured. During this period the samples were kept in complete darkness at 5°C. The detectors were irradiated at 22°C.
Optical setup and transmission analysis
The optical setup for transmission measurements was initially constructed as a simple one-dimensional laser setup with a laser (Aerotech He-Ne laser, 632 nm), a cuvette and a detector. It was found that this arrangement did not take into account the fluctuations in intensity of the He-Ne laser. Therefore, a setup with a reference detector was constructed (). In this setup an etalon split the beam. The beam going into detector 2 (Thorlabs Det 36 A) had an intensity of approximately 4% of the initial power due to the reflection on the surface of the etalon. The other beamline continued through a polarizer through the cuvette and into detector 1 (Thorlabs detector 110). The detectors were large enough to allow scattering to be neglected. The polarizer served the purpose of adjusting the intensity of light going into the detector. The cuvette was mounted in a cuvette holder in order to secure accurate repositioning from day to day using a two-axis translation stage. The output signals were analyzed using a LeCroy Wavesurfer Oscilloscope. The optical reproducibility of the setup was found to be better than 0.1%.
Figure 3. Optical setup. The laser setup used for determination of the optical density of the irradiated samples.
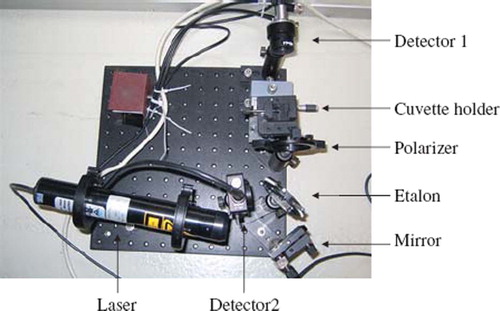
The visible spectrum of the Presage™ samples was obtained using a HELIOS spectrophotometer. The optical accuracy of this device was similar to that of the laser setup (better than 0.1% optical reproducibility).
As the cuvettes were not free from scratches and other perturbations of the surface every cuvette was measured four times in a 2 mm × mm matrix. The mean value of these four measurements was used in the calculation of the optical densities. The traditional definition of optical density OD = −log (I/I0) leads to the slope of the radiochromic response using the following expression:
where I is the intensity after the sample, I0 is the initial intensity, P1 is signal from detector 1 and P2 is the signal from detector 2. Pre and post refer to before and after irradiation. The change in optical density was calculated by normalizing the optical density (P1/P2) before and after irradiation. From the matrix measurements on each cuvette an average of the OD was calculated. The error on the average value obtained for each cuvette was 3.1% for the cuvettes used in this study.
Results
The optical spectra recorded after irradiation with γ-photons from 0 to 10 kGy is shown in . As expected, the maximum absorption was found at approximately 633 nm. In the change in OD at 633 nm is plotted with error bars for the standard deviation for multiple readings. The dose response was found to be linear in the dose range from 0 to 100 Gy. The dosimeter was found to have a saturation dose of approximately 100 Gy. At higher doses the response saturated with a lower response for additional doses. At 100 Gy a dose-rate induced reduction in response of 3% was measured (average value of ΔOD is plotted). In general the laser setup gave almost identical results to the spectrophotometer in the dose range studied. Therefore, measured data from the spectrophotometer is presented in the following.
Figure 4. Optical spectrum of Presage™ detectors postirradiation. The optical spectrum of Presage™ following irradiation with γ-photons from a Co-60 source in the dose range from 0 to 10 kGy.
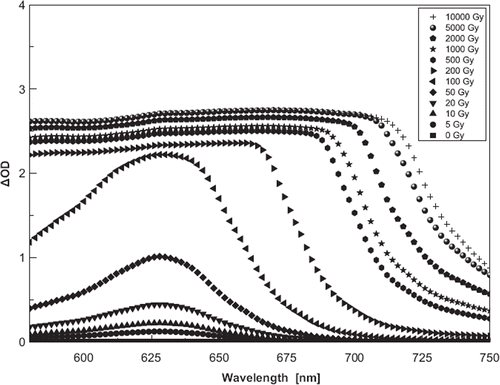
Figure 5. Presage™ detector dose response. The optical density change at 633 nm is plotted for Co-60 irradiated Presage™ samples. The dose response is found to be linear up to approximately 100 Gy.
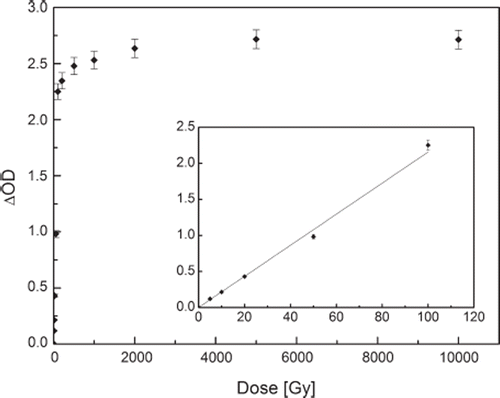
In the slopes of the dose response curves are presented for γ-photons compared to monoenergetic carbon ions. The average slopes of the carbon ion series were slightly lower than the average slopes of the two Co-60 series, within the experimental error.
Table II. Dose response of Presage™ detectors. The dose response of Presage™ following irradiation with carbon ions (plateau, 400 MeV/u) and γ-photons from Co-60. Carbon I and Carbon II are two different data sets and are therefore presented separately. The same applies for Co-60 I and Co-60 II. A lower slope is expected for high LET irradiation. Rˆ2 – linear correlation coefficient squared.
compares the optical response curves for Presage™ carbon ions in the plateau and the SOBP. The slope of the SOBP curve was higher than the one for the plateau measurements. In these data are plotted. The linear fit to the plateau data was not as good as the value of R-squared indicates.
Figure 6. Presage™ detector carbon ion dose response. The dose response of Presage™ dosimeters after irradiation with carbon ions in the Plateau and the SOBP at HIT. The response is found to be linear despite the difference in LET.
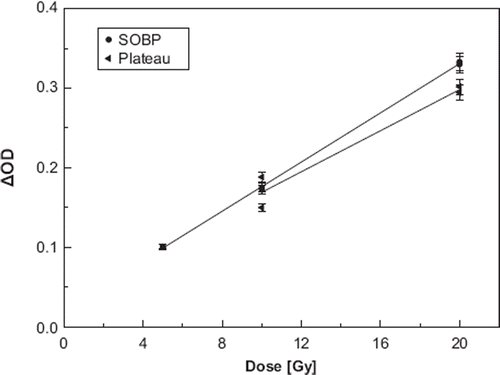
Table III. Detector response following SOBP and Plateau carbon ion irradiation. The slope of the dose response for Presage™ following irradiation with carbon ions both in the plateau and the SOBP. *The high energy deposition in and around the SOBP creates carbon ions with a variety of different energies and other particles all with different LET.
The fading experiments showed a change in ΔOD of 0.5 percentage point / day for both carbon and Co-60 over the two week period.
Discussion
A linear response of Presage™ between dose and optical response was found between 0 Gy and 100 Gy using photons. Furthermore, a linear relationship was found for carbon ions (both at the plateau and at the SOBP) from 0 to 20 Gy. We did not find any LET effect in our experiments and similar values for the slope of the dose response curves are reported.
The linear dose relationship for Presage™ was measured from 0 to 100 Gy with γ-photons. Above 100 Gy the spectrum of the dose response () was found to be non-linear at 633 nm. This observation is in agreement with Al-Nowais et al. [Citation30] that report a linear dose relationship in the dose range 0–120 Gy.
The dose-rate reduction in response of 3% at 100 Gy is supported by published data by Guo et al. [Citation21]. In this publication a change in dose-rate (of 2%) at dose rates of 2 Gy/min and 10 Gy/min was reported. Since the dose-rates of the gamma cells were 10.7 Gy/min and 92.8 Gy/min a more pronounced effect was expected.
The slopes of the optical density curves (presented in and ) were found to have similar dose responses despite very different LET radiation. The values reported are found to be within the range of optical density slopes reported by Mostaar et al. [Citation31]. In this study the concentrations of the chemical components were varied in order to determine the dynamic range of the Presage™ formula.
Since carbon ions have high LET (10.9 keV/μm), local saturation of the dosimeter is expected and thus a lower value of the slope. summarizes the slopes of optical density curves for carbon ions in the plateau compared to the SOBP. The slopes measured for the SOBP response is slightly higher than for the plateau. This result is in contradiction to our initial assumptions and is expected to be caused by the limited number of data points available at the plateau (). Other studies have confirmed that the Bragg Peak is normally underestimated in gel dosimetry compared to the entrance dose [Citation19]. However, in a study by Al-Nowais et al. [Citation29] the dose response of carbon ions in Presage™ was also found to be linear using energy modulated proton beams.
The postirradiation changes in optical fading was measured in this study and found to be 0.5% ΔOD/day. Guo et al. have reported significant fading 5-days postirradiation with values higher than in this study. The difference in fading times can be explained by variations in the chemical formulation of Presage™. Dosimeters with a less sensitive formulation are more stable and fading is diminished [Citation21].
This study has been focusing on point dosimeter measurements. However, the real potential for Presage™ lies in the development of accurate 3D dosimetry. We have recently installed a 3D optical tomography scanner (Octopus, MGS research) and are currently exploring possibilities for 3D dosimetry using Presage™.
In conclusion, Presage™ remains a dosimeter of interest for radiation therapy with other particles as well as photons and electrons in the therapy dose range.
Acknowledgements
We would like to thank John Adamovics from the Department of Chemistry and Biology, Rider University, Lawrenceville, New Jersey for providing the samples of Presage™. The irradiations in the kGy dose range were performed in collaboration with Jakob Helt-Hansen at Risoe National Laboratory for Sustainable Energy. This work is supported by CIRRO – The Lundbeck Foundation Center for Interventional Research in Radiation Oncology and The Danish Council for Strategic Research.
Declaration of interest: The authors report no conflicts of interest. The authors alone are responsible for the content and writing of the paper.
References
- Webb S. Intensity-modulated radiation therapy. Bristol: Institute of Physics; 2001.
- Letourneau D, Gulam M, Yan D, Oldham M, Wong JW. Evaluation of a 2D diode array for IMRT quality assurance. Radiother Oncol 2004;70:199–206.
- Kapulsky A, Gejerman G, Hanley J. A clinical application of an automated phantom-film QA procedure for radiation of IMRT treatment planning and delivery. Med Dosim 2004; 29:279–84.
- Baldock C, De Deene Y, Doran S, Ibbott G, Jirasek A, Lepage M, . Polymer gel dosimetry. Phys Med Biol 2010;55:R1–63.
- Hilts M, Audet C, Duzenli C, Jirasek A. Polymer gel dosimetry using x-ray computed tomography: A feasibility study. Phys Med Biol 2000;45:2559–71.
- Kelly BG, Jordan KJ, Battista JJ. Optical CT reconstruction of 3D dose distributions using the ferrous-benzoic-xylenol (FBX) gel dosimeter. Med Phys 1998;25:1741–50.
- Maryanski MJ, Zastavker YZ, Gore JC. Radiation dose distributions in three dimensions from tomographic optical density scanning of polymer gels. II. Optical properties of the BANG polymer gel. Phys Med Biol 1996;41: 2705–17.
- Oldham M, Siewerdsen J, Shetty A, Jaffray D. High resolution gel-dosimetry by optical-CT and MR scanning. Med Phys 2001;28:1436–42.
- Oldham M, Siewerdsen J, Kumar S, Wong J, Jaffray D. Optical-CT gel-dosimetry I: Basic investigations. Med Phys 2003;30:623–34.
- Oldham M, Kim L. Optical-CT gel-dosimetry II: Optical artifacts and geometrical distortion. Med Phys 2004;31: 1093–104.
- Björeland A, Lindvall P, Karlsson A, Gustavsson H, Bäck SÅ, Karlsson M, . Liquid ionization chamber calibrated gel dosimetry in conformal stereotactic radiotherapy of brain lesions. Acta Oncol 2008;47:1099–109.
- Macdougall ND, Pitchford WG, Smith MA. A systematic review of dose measurements in photon radiotherapy using polymer and Fricke gel dosimetry. Phys Med Biol 2002;47: R107–21.
- Doran SJ. The history and principles of chemical dosimetry for 3-D radiation fields: Gels, polymers and plastics. Appl Radiat Isot 2009;67:393–8.
- Schreiner LJ. Review of Fricke gels. J Phys: Conf Ser 2004;3: 9–21.
- Gore JC, Kang YS, Schulz RJ. Measurement of radiation dose distributions by nuclear magnetic resonance (NMR) imaging. Phys Med Biol 1984;29:1189–97.
- Schulz RJ, deGuzman AF, Nguyen DB, Gore JC. Dose-response curves for Fricke-infused agarose gels as obtained by nuclear magnetic resonance. Phys Med Biol 1990;35:1611–22.
- Maryanski MJ, Gore JC, Kennan RP, Schulz RJ. NMR relaxation enhancement in gels polymerized by ionizing radiation: A new approach to 3D dosimetry by MRI. Magn Reson Imaging 1993;11:253–8.
- Baldock C. Historical overview of the development of gel dosimetry: Another personal perspective. J Phys: Conf Ser 2009;164:012002.
- Gustavsson H, Bäck SÅJ, Medin J, Grusell E, Olsson LE. Linear energy transfer dependence of a normoxic polymer gel dosimeter investigated using proton beam absorbed dose measurements. Phys Med Biol 2004;49:3847–55.
- Adamovics J, Maryanski MJ. Characterisation of PRESAGE™: A new 3-D radiochromic solid polymer dosimeter for ionising radiation. Radiat Prot Dosimetry 2006;120:107–12.
- Guo P, Adamovics J, Oldham M. Characterization of a new radiochromic three-dimensional dosimeter. Med Phys 2006; 33:1338–45.
- Guo P, Adamovics J, Oldham MA. A practical three-dimensional dosimetry system for radiation therapy. Med Phys 2006;33:3962–72.
- Brady S, Brown W, Clift C, Yoo S, Oldham M. Investigation into the feasibility of using PRESAGE™/optical-CT dosimetry for the verification of gating treatments. Phys Med Biol 2010;55:2187–202.
- Oldham M, Sakhalkar H, Guo P, Adamovics J. An investigation of the accuracy of an IMRT dose distribution using two- and three- dimensional dosimetry techniques. Med Phys 2008;35:2072–80.
- Butts JJ, Katz R. Theory of RBE for heavy ion bombardment of dry enzymes and viruses. Radiat Res 1967;30:855–71.
- Hansen JW, Olsen KJ. Theoretical and experimental radiation effectiveness of the free radical dosimeter alanine to irradiation with heavy charged particles. Radiat Res 1985;104:15–27.
- Paganetti H, Goitein M. Biophysical modelling of proton radiation effects based on amorphous track models. Int J Radiat Biol 2001;77:911–28.
- Greilich S, Grzanka L, Bassler N, Andersen CE, Jäkel O. Amorphous track models: A numerical comparison study. Radiat Meas 2010;45:1406–9.
- Al-Nowais S, Doran S, Kacperek A, Krstajic N, Adamovics J, Bradley D. A preliminary analysis of LET effects in the dosimetry of proton beams using Presage and Optical CT. Appl Rad Isot 2009;67:415–8.
- Al-Nowais S, Nisbet A, Adamovics J, Doran S. An attempt to determine the saturation dose for Presage. J Phys: Conf Ser 2009;164:012043.
- Mostaar A, Hashemi B, Zahmatkesh MH, Aghamiri SMR, Mahdavi SR. A basic dosimetric study of Presage: The effect of different amounts of fabricating components on the sensitivity and stability of the dosimeter. Phys Med Bio 2010;55:903–12.