To the Editor,
While dose escalation has shown promising results in radiotherapy for lung cancer [Citation1], increased pulmonary toxicity has limited its applicability [Citation2,Citation3]. At respiratory-gated radiation therapy (RT), the lung dose could be reduced by selecting beam-on-phase in the respiratory cycle (i.e. the gating window) [Citation4–6]; however, the time required for irradiation was increased [Citation7]. Prolongation of the treatment time may lead to set-up errors due to patient motion and increase the load on available instruments. Residual tumor motion within the gating window can be reduced when a narrow gating window is used [Citation8]. However, extremely narrow gating windows will markedly shorten the available duration per respiratory cycle and will prolong the treatment time [Citation9].
In this simulation study we evaluated the influence of gating window width on lung dose and treatment time. Between January 2007 and April 2009 we subjected 42 consecutive lung cancer patients to four-dimensional computed tomography scanning (4D-CT scanning). After excluding ten patients whose images were unacceptable due to extremely irregular respiration and 17 patients whose tumors exhibited less than 5 mm 3D motion on 4D-CT images [Citation10], the CT data sets of the 15 patients with 15 tumors were used to simulate different treatment scenarios. Of these, nine were located in the right lung (one each in the right upper- and right middle lobe, seven in the right lower lobe), two were in the left upper- and four in the left lower lobe.
The CT scanner used was a GE LightSpeed RT (GE Medical Systems, Waukesha, WI, USA). We described the 4D-CT procedure elsewhere [Citation11]. Briefly, during scanning, the patients were supine with their arms above the head; respiration was quiet and uncoached. A commercially available external respiratory monitor system (Real-Time Position Management system, Varian Medical Systems, Palo Alto, CA, USA) recorded respiratory motion in temporal correlation with CT scan acquisition. Each respiratory cycle was divided into ten specific respiratory phases and each reconstructed CT image was assigned to one of these ten phases. Ten 3D-CT image sets were obtained from one 4D-CT data set. For a typical patient the approximate scan time was 150 s and the approximate computed tomography dose index (CTDIvol) [Citation12] was 30 mGy.
For target delineation and treatment planning we used a commercially available treatment planning system (XIO version 4.33.02, Computerized Medical Systems, St. Louis, MO, USA). The same radiation oncologist contoured the clinical target volume (CTV) and defined ten CTVs at different respiratory phases on ten corresponding 3D-CT image sets with a standardized window setting (level = 2500, width = 1500). A composite CTV was constructed by combining the CTVs and considered the internal target volume (ITV). A 5-mm margin was added to the ITV to generate planning target volumes (PTVs). The field edge was 5 mm beyond the PTV. We used 5–8 static 6-MV x-ray beams with a prescription dose of 60 Gy at the isocenter. Inhomogeneity corrections based on the superposition method were employed.
Using phase-based gating around end-expiration we developed four treatment plans for each patient (). They were gated treatment protocols using a narrow- (Protocol Gating Narrow, PgN), medium-(Protocol Gating Medium, PgM), and wide (Protocol Gating Wide, PgW) gating window, and a non-gated irradiation protocol (Protocol Non-Gating, Png). Under PgN, PgM and PgW, the beam is on during 10%, 30%, and 50% of the respiratory cycle, respectively. Under the gated protocols we used 1-, 3-, and 5 CTVs around end-expiration to form a composite CTV. In Png, all ten CTVs were used to form a combined CTV to simulate non-gated irradiation. For each of the four protocols in the same patient, we applied identical treatment parameters, including the isocenter location and beam directions and -weighting. To evaluate the protocols we used two dosimetric parameters, i.e. the mean lung dose (MLD) and V20 (% of total lung receiving ≥20 Gy). We used a 3D-CT data set that corresponded to end-expiration for dose calculation. Using a dose rate of 600 monitor units (MUs)/min we calculated the time required for the delivery of 100 MUs based on the width of the gating window.
Figure 1. Protocols under the four treatment scenarios. Example of the isodose distribution under PgN, PgM, PgW and Png is shown.
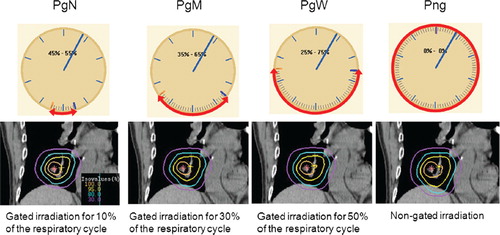
Pairwise comparisons of the mean lung dosimetric parameters (mean lung dose and V20) were made with paired t-test with Bonferroni's correction. The assumption of normality was tested using Shapiro–Wilk test. The statistical tests were performed with PASW statistics 17.0 for Windows (IBM Japan, Ltd., Tokyo, Japan). A two-sided p-value <0.05 was considered statistically significant.
The average (±S.D.) CTV was 46.3 cm3 (±59.2 cm3). The average (±S.D.) 3D CTV centroid motion was 8.3 mm (±3.8 mm). In all 15 patients, the composite CTV increased as the number of combined CTVs increased. Comparison of the average CTV under Png (74.8 cm3) and under PgN, PgM and PgW (46.3, 54.3, and 61.5 cm3, respectively) showed that a reduction of 40–20% could be achieved by respiratory gating.
The mean lung dose and V20 delivered under the four protocols are shown in . The average (±S.D.) mean lung dose was 5.26 Gy (±2.73), 5.45 Gy (±2.83), 5.60 Gy (±2.95), and 5.91 Gy (±2.92) under PgN, PgM, PgW, and Png, respectively; the average (±S.D.) V20 was 10.4% (±6.2), 10.8% (±6.3), 11.1% (±6.6), and 11.8% (±6.4), respectively. The standard deviations for MLD and V20 were relatively large. Pairwise comparisons of the mean lung dose and V20 using paired t-test with Bonferroni's correction indicated significant differences among all treatment protocols (). There was a clear trend toward an increasing lung dose with increasing width of gating window.
Figure 2. Mean lung dose and percentage of total lung receiving ≥ 0 Gy (V20) of the protocols in the 15 patients. Data are presented as mean and 95% confidence interval.
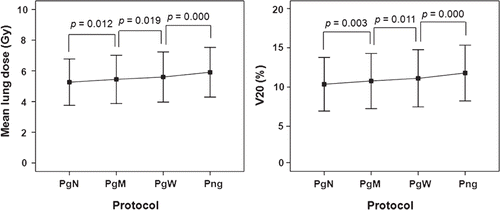
Under Png the treatment time required for the delivery of 100 MUs was 10 s at a dose rate of 600 MUs/min. The treatment time under the gated protocols was calculated according to the width of the gating window we set. It became longer with a narrower gating window; the treatment time required for 100 MUs was 100-, 33.3-, and 20 s for PgN, PgM and PgW, respectively.
is a diagram of the patient-averaged reduction in the mean lung dose plotted against the time (s) required for the delivery of 100 MUs. Compared to the non-gated protocol (Png), gated delivery for 50% of the respiratory cycle (PgW) would reduce mean lung dose at a reasonable cost of treatment time prolongation. As gating window gets narrower (PgM, PgN), more and more prolongation of treatment time would be required to reduce mean lung dose.
Discussion
Our results demonstrate the trade-off between lower lung dosimetric parameters and increased treatment time. As shown in , narrowing of the gating window from a 30% to a 10% of the respiratory cycle (i.e. from PgM to PgN) produced a small lung dose reduction relative to the magnitude of the corresponding treatment time prolongation. Therefore, we suggest that a gating window width of a 30% of the respiratory cycle was appropriate under our study conditions.
Vedam et al. [Citation9] who analyzed the diaphragm position of two patients selected a 40% duty cycle (40% of the respiratory cycle) by balancing the desired reduction in the margins with a corresponding increase in the treatment time. Their results are similar to that of the current study, although their analysis was based on only one patient. To our knowledge, the present study is the first comparison of lung dosimetric parameters and the irradiation time among protocols featuring different gating-window widths. Considering the paucity of data, we cannot conclude whether our findings would apply to other patient populations. In this study, we used phase-based gating around end-expiration and a gating window of a 10%, 30% and 50% of the respiratory cycle, and the recommended window width can only be used under these particular circumstances. In addition, the time required for irradiation is part of the total treatment time. As the time required for patient set-up or image guidance differs among facilities, significance of the irradiation time for patient throughput is not consistent. Further work in assessing the relationship between lung dosimetric parameters and treatment time in gated radiotherapy is indicated.
It is unclear whether the reduction of 0.31–0.65 Gy in mean lung dose by respiratory gating (Png vs. PgN, PgM, PgW) is clinically relevant, especially when conventional fractionation of 1.8–2.0 Gy per day is used [Citation2,Citation3]. Our study was intended for future dose escalation, and we assumed the use of medium to large fraction sizes in 3D conformal RT for lung cancer. At present, little is known about the relationship between the radiation dose and lung toxicity at RT with large fraction size [Citation13]. Assuming the use of larger fractions, the clinical significance of the reduction in the lung dosimetric parameters should not be underestimated.
We included the patients with tumors of various volumes. The relatively heterogeneous patient group could be a limitation of this study. However, recently we showed that the target volume was not significantly correlated with the lung dose reduction by respiratory gating [Citation10]. In the present study, there was also no clear difference in the estimated lung dose reduction between large and small tumors. Including heterogeneous tumors in the analysis might lead to a relatively wide applicability of the results of our present study.
In conclusion, by narrowing the gating window the lung dosimetric parameters could be lowered at the cost of treatment time prolongation. As gating window gets narrower, more and more prolongation of treatment time would be required to reduce lung dose. A gating window width of a 30% of the respiratory cycle was appropriate under our study conditions.
Acknowledgements
This study was partially supported by a Grant-in-Aid for Young Scientists (B), #20790900, from the Ministry of Education, Culture, Sports, Science and Technology of Japan, 2008–2009. The authors report no conflicts of interest.
References
- Baumann P, Nyman J, Lax I, Friesland S, Hoyer M, Ericsson SR, . Factors important for efficacy of stereotactic body radiotherapy of medically inoperable stage I lung cancer. A retrospective analysis of patients treated in the Nordic countries. Acta Oncol 2006;45:787–95.
- Graham MV, Purdy JA, Emami B, Harms W, Bosch W, Lockett MA, . Clinical dose-volume histogram analysis for pneumonitis after 3D treatment for non-small cell lung cancer (NSCLC). Int J Radiat Oncol Biol Phys 1999;45: 323–9.
- Tsujino K, Hirota S, Endo M, Obayashi K, Kotani Y, Satouchi M, . Predictive value of dose-volume histogram parameters for predicting radiation pneumonitis after concurrent chemoradiation for lung cancer. Int J Radiat Oncol Biol Phys 2003;55:110–5.
- Korreman S, Mostafavi H, Le QT, Boyer A. Comparison of respiratory surrogates for gated lung radiotherapy without internal fiducials. Acta Oncol 2006;45:935–42.
- Nottrup TJ, Korreman SS, Pedersen AN, Persson GF, Aarup LR, Nystrom H, . Interfractional changes in tumour volume and position during entire radiotherapy courses for lung cancer with respiratory gating and image guidance. Acta Oncol 2008;47:1406–13.
- Slotman BJ, Lagerwaard FJ, Senan S. 4D imaging for target definition in stereotactic radiotherapy for lung cancer. Acta Oncol 2006;45:966–72.
- Fox T, Simon EL, Elder E, Riffenburgh RH, Johnstone PAS. Free breathing gated delivery (FBGD) of lung radiation therapy: Analysis of factors affecting clinical patient throughput. Lung Cancer 2007;56:69–75.
- Berbeco RI, Nishioka S, Shirato H, Chen GTY, Jiang SB. Residual motion of lung tumours in gated radiotherapy with external respiratory surrogates. Phys Med Biol 2005;50: 3655–67.
- Vedam SS, Keall PJ, Kini VR Mohan R. Determining parameters for respiratory-gated radiotherapy. Med Phys 2001; 28:2139–46.
- Saito T, Sakamoto T, Oya N. Predictive factors for lung dose reduction by respiratory gating at radiotherapy for lung cancer. J Radiat Res 2010;51:691–8.
- Saito T, Sakamoto T, Oya N. Comparison of gating around end-expiration and end-inspiration in radiotherapy for lung cancer. Radiother Oncol 2009;93:430–5.
- Turner AC, Zankl M, DeMarco JJ, Cagnon CH, Zhang D, Angel E, . The feasibility of a scanner-independent technique to estimate organ dose from MDCT scans: Using CTDIvol to account for differences between scanners. Med Phys 2010;37:1816–25.
- Paludan M, Hansen AT, Petersen J, Grau C, Hoyer M. Aggravation of dyspnea in stage I non-small cell lung cancer patients following stereotactic body radiotherapy: Is there a dose-volume dependency? Acta Oncol 2006;45:818–22.