Abstract
Background. Patients with non-small cell lung cancer (NSCLC) have poor prognosis partly because of high local failure rates. Escalating the dose to the tumour may decrease the local failure rates and thereby, improve overall survival, but the risk of complications will limit the possibility to dose-escalate a broad range of patients. Escalating only PET-active areas of the tumour may increase the potential for reaching high doses for a variety of tumour sizes and locations. Material and methods. Ten patients were randomly chosen for a dose escalation planning study. A planning target volume (PTV) was defined on the mid-ventilation scan of a four-dimensional computed tomography (4D-CT) scan and a boost planning target volume (PTV-boost) was defined based on a positron emission tomography computed tomography (PET-CT) scan. Treatment plans were created aiming to reach the highest achievable of 74 Gy, 78 Gy or 82 Gy in 2 Gy per fraction prescribed to the PTV-boost without compromising normal tissue constraints and with the PTV prescribed in all cases a biological equivalent dose in 2 Gy fractions of 66 Gy. Results. Nine of ten patients could be escalated to the highest dose level (82 Gy), while one patient was limited by the oesophagus dose constraint and could only reach 74 Gy. Four patients could be dose-escalated above 82 Gy without compromising normal tissue constraints. Conclusion. Dose-escalating only the PET-active areas of lung tumours to doses of 82 Gy while respecting normal tissue constraints is feasible, also in a series of unselected patients including cases with relatively large tumours.
Non-small cell lung cancer (NSCLC) patients have in general very low survival rates, for example in Denmark the five years survival is as low as 10% [Citation1]. For patients treated with radiotherapy (RT) to standard dose levels of 60–66 Gy in 30–33 fractions, the low survival rates are partly a consequence of local failures. Clinical studies have shown that 40–55% of patients have recurrent disease in their original tumour site [Citation2,Citation3], and that achieving local control requires dose levels of 80–100 Gy in 2 Gy fractions [Citation4,Citation5]. Dose escalation towards these levels should therefore be pursued. Unfortunately, it can in many cases be difficult to escalate the dose to the lung targets without causing a high risk of complications. Radiation-induced pneumonitis, in particular, may be a problem when irradiating a large portion of the lung [Citation6,Citation7].
In previous studies, comparison between the positron emission tomography (PET)-active volumes before and after RT [Citation8] has shown a large overlap. Since the latter volume is associated with local relapse [Citation9], a generally accepted hypothesis is that areas that are PET-active before RT are more radio-resistant and therefore, require higher dose in order to obtain local control.
Several clinical series, as well as planning studies, have looked at the possibility to escalate the dose to either the whole lung tumour [Citation4,Citation10,Citation11] or to the central part of the tumour [Citation7,Citation12]. With the increasing availability of PET-computed tomography (CT), functional image-based tumour definition of NSCLC tumours has become feasible. Escalation of the dose only to the PET-active areas could potentially make dose escalation available to a broader range of patients.
In this study, we have therefore investigated to what extent we can escalate the dose to the PET-active areas of stage II-III inoperable NSCLC tumours. The study was performed in a series of unselected patients representing our clinical variation in terms of tumour size and location, as part of the basis for designing a Danish clinical dose escalation protocol for these patients.
Material and methods
Patient selection
We randomly selected ten patients from 55 NSCLC patients treated with a curative intent between February 2010 and February 2011. The ten patients included had a pathologically proven inoperable NSCLC, stage IIB-IIIB (American Joint committee on Cancer Staging [Citation13]). All patients received two to three cycles of induction chemotherapy (IC) before the planning CT-scan and PET/CT. The IC included carboplatin combined with vinorelbine delivered in 21 day cycles. One patient continued with another series of carboplatin and vinorelbine during RT, while five patients were treated with concomitant vinorelbine three times a week during RT in a Danish phase II protocol. Further patient and tumour characteristics can be found in .
Volume definition
All patients underwent a 3D-CT with contrast, a 4D-CT without contrast, and a PET-CT scan with the 18F-deoxyglucose (FDG) tracer. The PET-CT was acquired between one and 21 days before treatment start (median 10 days). The gross target volume (GTV) for the primary tumour (GTVt), and pathological lymph nodes (GTVn), were delineated on the mid-ventilation scan of the 4D-CT [Citation14] by two clinical oncologists with the help of a specialist radiologist, using both lung window and mediastinal window in the Eclipse TPS (Varian Medical Systems, CA, USA), guided by the visual interpretation of the PET-CT scan and the 3D-CT with contrast. The volume of the GTVs ranged from 9 to 359 cm3 (median 72.6 cm3). A margin of 0.5 cm was added to the GTVt and GTVn to create respective CTVs, which was modified for overlap with bones and major blood vessels in the mediastinum. The CTVs were expanded to the Internal Target Volume (ITV) by first adding another 0.5 cm in all directions to each CTV, and then merging the two resulting sub-volumes into one. The planning target volume (PTV) was created by adding another 0.5 cm lateral and 0.8 cm cranio-caudal to the ITV. All margins used followed the recommendations from the Danish Oncological Lung cancer Group (DOLG).
Within the PTV, the PET-active areas of the tumour were defined as the PTV-boost. The PET-active lymph nodes in the mediastinum were excluded as proximity to oesophagus and trachea, in many cases, will make doses above 66 Gy in 2 Gy fractions unachievable. The PET-active areas were auto-segmented using the registration module in the Eclipse treatment planning system (TPS) (Varian Medical systems, Palo Alto, CA, USA). The tumour volume was defined by the 40% of the maximum standard uptake value (SUV) in the tumour. A 5 mm isotropic margin was subsequently added to the auto-segmented volume to obtain the PTV-boost. The PTV-boost was transferred from the PET-CT to the mid-ventilation scan of the 4D-CT using a rigid registration. The deformation errors between the two scans, due to different fixation, as well as day-to-day anatomic changes, were estimated to be within 2–3 mm. This error is likely to have been smaller if the PET-CT had been acquired in the fixation used for RT, which was not the case for the patients included in this study.
Dose levels and constraints
The PET active area, PTV-boost, was planned to receive the highest possible of three dose levels; 74 Gy in 37 fractions, 78 Gy in 39 fractions or 82 Gy in 41 fractions. The PTV was prescribed a biologically equivalent dose in 2 Gy fractions (BED2Gy) of 66 Gy. Based on calculations with the linear quadratic model with an α/β = 10 Gy [Citation6], PTV was prescribed a physical dose of 67 Gy in 37 fractions, 67.5 Gy in 39 fractions, or 68 Gy in 41 fractions. The dose to the PTV-boost was planned as a simultaneously integrated boost. During the planning optimisation, we required 99% of PTV-boost to be covered by the 95% isodose curve of the boost dose level and 95% of the PTV to be covered by the 95% isodose curve of the lower dose level [Citation15].
shows a list of the constraints to organs at risks (ORs) that were respected to minimise the risk of complications. The constraints on the spinal canal, heart, and the volume of the lung receiving at least 20 Gy, followed the recommendations from DOLG. For the oesophagus, DOLG recommends a maximum dose of 66 Gy, but in this study, we allowed a small volume to receive a slightly higher dose. In addition, to these we use constraints on plexus brachialis [Citation16], trachea, and the mean lung dose [Citation17]. The most demanding constraints were lung dose for all tumour locations, heart dose for tumours in the lower part of the lung and the dose to plexus brachialis for tumours in the upper part of the lung.
Table I. Constraints on dose to ORs. Dmax is the maximal allowed dose for the organ, Dmean the maximal mean dose allowed for the tissue and VD the maximal volume of the organ receiving at least the dose D defined as a percentage of the entire volume or a fixed number. For the oesophagus V(BED2Gy = 66Gy) is the volume receiving at least a biologically equivalent dose in 2 Gy fractions of 66 Gy calculated with an α/β = 10.
IMRT planning
For all patients, intensity modulated radiotherapy (IMRT) plans were optimised with a pencil beam algorithm and then, finally calculated with a convolution algorithm accounting for lateral scatter, AAA in the Eclipse TPS (Varian Medical Systems, Ca, US) using only 6 MV photons. The plans were optimised such that all constraints in were respected, the dose to the lung minimised, and at the same time the number of beams were kept low, and preferable only coplanar beams were used. All patients ended up with between five and seven beams. In four patients, it was possible to use only coplanar beams, while two to three non-coplanar were required in six patients, in order to minimise the dose to either lung or heart.
Results
The volumes of the PTV ranged from 191 to 1088 cm3 with a median of 531 cm3 (). The volume of PTV-boost ranged from 6 to 147 cm3 with a median of 66 cm3. There was a large variation in the tumour location (). a and b shows the delineation of PTV and PTV-boost for one patient, on a blend between the 4D-CT mid-ventilation scan and the PET scan.
Figure 1. Delineation, beam configuration and isodose curves for case 8, with a. showing a transversal and b. a sagittal view of the tumour on an image blended between the mid-ventilation scan of the 4D-CT and the PET scan. The blue curve is the PTV. The orange curve is 40% of the maximal tumour SUV and adding a 5 mm isotropic margin to this yields PTV-boost represented by the cyan curve. The heart (magenta), the oesophagus (light green) and the spinal canal (green) are close to the target. Part c. shows the transversal and d. the sagittal view with beam configuration and isodose levels. The isocenters of the fields are represented by a yellow line. The plan consisted of four coplanar beams (field number 1–4) and three non-coplanar beams (field number 5–7). The orange isodose curve is 95% of the 82 Gy prescribed to PTV-boost, while the magenta is 95% of 68 Gy (BED2Gy = 66) prescribed to PTV. The red 30 Gy isodose curve shows the conformity of the plan.
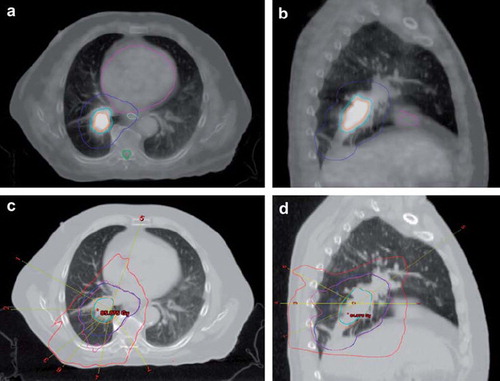
Table II. Tumour characteristics, dose escalation level obtained and lung dose. For the description of the tumour location the following abbreviations are used. Side: L/R is left/right, lobe: L/M/U is lower/middle/upper and position: C/P is central/peripheral. The lung volume used is the lung excluding the GTV.
Nine of ten patients could be escalated to the highest attempted dose level of 82 Gy. Five of these received a mean lung dose close to 20 Gy, and it would therefore, not be possible to escalate their dose further, while the four remaining cases could be escalated further without compromising our constraints on organs at risk (OR). One patient could only reach 74 Gy due to proximity of the PTV-boost to the oesophagus. Across all patients, 95% of each prescribed dose covered 99.7% of PTV-boost and 98.0% of the PTV (median values). With respect to ORs, all constraints listed in were complied with. The mean lung dose, as well as the volume of lung receiving at least 20 Gy, are listed in .
An example of the beam configuration and isodose curves for one of the patients receiving 82 Gy is shown in . This plan used four coplanar and three non-coplanar beams, of which one beam was used to minimise the dose to the heart, while two beams were chosen to reduce the lung dose. The dose volume histograms for this case are shown in for the relevant ORs.
Discussion
Dose escalation in RT of lung cancer has been the topic of several dose escalation trials, involving a range of strategies in terms of patient selection, tumour definition, normal tissue constraints, dose levels, and fractionation schemes [Citation4,Citation10,Citation11,Citation18]. The long-term outcomes of these will be instrumental in the further selection of safe dose escalation strategies to improve patient survival. In contrary to the majority of trials, the present planning study has investigated dose escalation restricted to only PET-active parts of the tumour, with the result that nine of ten patients could reach our highest dose level (82 Gy). Although this is a limited patient material, we find these unselected patients to be representative of our clinical reality in terms of variation in tumour location and size.
In this study, the boost target was treated with 2 Gy per fraction, while the large PTV received a slightly hyper-fractionated dose. Another approach would be to prescribe the dose to the large PTV in 2 Gy per fraction, and then hypo-fractionate the dose to the boost target. The advantage of the hypo-fractionated regime is a shorter overall treatment time, diminishing the effect of tumour repopulation (For NSCLC the dose recovered per day is 0.45 Gy [Citation19]), while it requires more care with respect to the normal tissues and their constraints. One clinical trial [Citation18], has shown that hypo-fractionated dose escalation in NSCLC can be achieved without high risk of complication, while another [Citation11] argues that hyper-fractionating the dose to the whole tumour with two fractions of 1.8 Gy daily will make dose escalation more feasible for tumours close to critical organs. Another important issue in the design of dose escalation trials is the method used to determine the dose escalation level for each patient. If the trial is not randomised according to dose level, the dose can, for example, be prescribed according to the effective lung volume irradiated [Citation4,Citation10,Citation18], or as the highest achievable dose level not compromising normal tissue constraints [Citation11].
Large tumour volumes reduce the achievable levels of dose in escalation trials, because they lead to irradiation of a larger volume of the lung and hence, constraints on dose to the lung will limit dose escalation. Our study represented the tumour volumes of our daily clinical practice and included also patients with rather large PTVs. Firstly, because no patient selection based on tumour volumes or planning feasibility was performed, yielding large GTVs (median 72.6 cm3). Secondly, relatively large margins compared to other dose escalation trials [Citation11,Citation18], were used for the generation of target volumes following the recommendations from the Danish Oncological Lung cancer Group. Using smaller margins will make dose escalation to higher dose levels available to a broader range of patients, but increases the risk of local recurrences on the periphery of the planning target volume. Our clinic already uses 4D-CT for target delineation, as well as daily cone-beam CT for patient setup, and decreasing our margins may be feasible, while keeping a low risk of geographical miss [Citation20].
The optimal solution to the delineation of the PET-active volume is still much debated [Citation21]. Besides, as the anatomical representation may differ between the acquisition of the PET-CT and the 4D-CT, clinical access to 4D PET-CT would be advantageous. Using pre-treatment PET to define the boost volumes in the way used in this study, generates smaller boost volumes compared with studies where the boost is generated using the GTV with a small margin added [Citation12], because only part of the GTV is PET-active. Assuming that the PET-active part of the tumour is also the part requiring the largest dose is still a hypothesis based on a moderate number of studies of the correlation between the PET signal before RT and the pattern of local failures [Citation8,Citation9]. Another issue is the stability of the pre-treatment PET signal, as well as the PET signal throughout the course of RT. Aerts et al. found a large overlap between PET-active areas of the tumour before and during RT, while noting that the volumes of these areas could change considerably [Citation22], similarly to the volume changes of a GTV delineated on CT [Citation20]. These volume changes have to be taken into account when choosing margins, as well as PET threshold values for the delineation of PTV-boost, and similar studies are required to obtain knowledge about the stability of the pre-treatment PET signal.
The decision to exclude lymph nodes in the mediastinum from the boost PTV is debatable, and unfortunately the majority of pattern of failure studies do not distinguish between a failure in GTV of the primary tumour, or the GTV of the lymph nodes. Sura et al. looked at 26 NSCLC patients with local failure and found that all patients had recurrence in the primary tumour, while only eight had recurrences in the irradiated lymph nodes, five of these being marginal or geographic misses [Citation23].
As the knowledge about the PET signal, as well as the confidence in its interpretation increases, the advantages of more sophisticated dose painting, compared to just escalation of one area, will become an issue that receives great attraction [Citation24]. Also with respect to the involved normal tissues, improved imaging techniques will reveal important information on the functional properties of different organs. Acquiring a single photon emission tomography (SPECT) scan before radiotherapy, may for instance be used to identify well-perfused areas of the lung and preferentially avoid these in treatment planning [Citation25]. In our institution, we are currently developing a 4D-CT based method for lung ventilation imaging that has considerable potential for biologically adaptive RT according to normal tissue functional imaging [Citation26].
The number of beams (in particular non-coplanar beams), was kept as low as possible in this study. This objective was applied to minimise the treatment delivery time and thereby, decreasing the risk of patient movement during treatment, maintaining a relatively comfortable treatment time as well as well as limiting the use of accelerator capacity.
In conclusion, this study has shown that for a wide range of tumour sizes and locations, the PET-active areas can be dose-escalated to 82 Gy without compromising normal tissue constraints. In only one of ten cases, dose escalation above 74 Gy could not be achieved due to the proximity to the oesophagus.
Acknowledgements
Ditte Sloth Møller acknowledges support by the Nordic Association for Clinical Physics and Varian Medical Systems (Palo Alto, CA, USA). This work was supported by CIRRO - The Lundbeck Foundation Center for Interventional Research in Radiation Oncology.
Declaration of interest: The authors report no conflicts of interest. The authors alone are responsible for the content and writing of the paper.
References
- Dansk Lunge Cancer Gruppe & Dansk Lunge Cancer Register, Årsrapport 2007.
- Martel MK, Ten Haken RK, Hazuka MB, Kessler ML, Strawderman M, Turrisi AT, . Estimation of tumor control probability model parameters from 3-D dose distributions of non-small cell lung cancer patients. Lung Cancer 1999;24:31–7.
- Mehta M, Manon R. Are more aggressive therapies able to improve treatment of locally advanced non-small cell lung cancer: Combined modality treatment? Semin Oncol 2005;32:S25–34.
- Kong FM, Haken RKT, Schipper MJ, Sullivan MA, Chen M, Lopez C, . High-dose radiation improved local tumor control and overall survival in patients with inoperable/unresectable non-small-cell lung cancer: Long-term results of a radiation dose escalation study. Int J Radiat Oncol Biol Phys 2005;63:324–33.
- Mehta M, Scrimger R, Mackie R, Paliwal B, Chappell R, Fowler J. A new approach to dose escalation in non-small-cell lung cancer. Int J Radiat Oncol Biol Phys 2001;49:23–33.
- Fenwick JD, Nahum AE, Malik ZI, Eswar CV, Hatton MQ, Laurence VM, . Escalation and intensification of radiotherapy for stage III non-small cell lung cancer: Opportunities for treatment improvement. Clin Oncol 2009;21: 343–60.
- Holloway CL, Robinson D, Murray B, Amanie J, Butts C, Smylie M, . Results of a phase I study to dose escalate using intensity modulated radiotherapy guided by combined PET/CT imaging with induction chemotherapy for patients with non-small cell lung cancer. Radiother Oncol 2004;73: 285–7.
- Aerts HJ, van Baardwijk AA, Petit SF, Offermann C, Loon JV, Houben R, . Identification of residual metabolic-active areas within individual NSCLC tumours using a pre-radiotherapy 18Fluorodeoxyglucose-PET-CT scan. Radiother Oncol 2009;91:386–92.
- Manus MPM, Hicks RJ, Matthews JP, Wirth A, Rischin D, Ball DL. Metabolic (FDG-PET) response after radical radiotherapy/chemoradiotherapy for non-small cell lung cancer correlates with patterns of failure. Lung Cancer 2005;49: 95–108.
- Bradley J, Graham MV, Winter K, Purdy JA, Komaki R, Roa WH, . Toxicity and outcome results of RTOG 9311: A phase I-II dose-escalation study using three-dimensional conformal radiotherapy in patients with inoperable non-small-cell lung carcinoma. Int J Radiat Oncol Biol Phys 2005;61:318–28.
- Van Baardwijk A, Wanders S, Boersma L, Borger J, Ollers M, Dingemans A-MC, . Mature results of an individualized radiation dose prescription study based on normal tissue constraints in stages I to III non-small-cell lung cancer. J Clin Oncol 2010;28:1380–6.
- St-Hilaire J, Sévigny C, Beaulieu F, Germain F, Lavoie C, Dagnault A, . Dose escalation in the radiotherapy of non-small-cell lung cancer with aperture-based intensity modulation and photon beam energy optimization for non-preselected patients. Radiother Oncol 2009;91:342–8.
- Tanoue LT, Detterbeck FC. New TNM classification for non-small-cell lung cancer. Expert Rev Anticancer Ther 2009;9:413–23.
- Wolthaus JWH, Schneider C, Sonke J-J, van Herk M, Belderbos JSA, Rossi MMG, . Mid-ventilation CT scan construction from four-dimensional respiration-correlated CT scans for radiotherapy planning of lung cancer patients. Int J Radiat Oncol Biol Phys 2006;65:1560–71.
- Prescribing, recording, and reporting intensity-modulated photon-beam therapy. J ICRU 2010 Report No. 83;10.
- Emami B, Lyman J, Brown A, Coia L, Goitein M, Munzenrider JE, . Tolerance of normal tissue to therapeutic irradiation. Int J Radiat Oncol Biol Phys 1991;21:109–22.
- Marks LB, Bentzen SM, Deasy JO, Kong FM, Bradley JD, . Radiation dose-volume effects in the lung. Int J Radiat Oncol Biol Phys 2010;76:S70–6.
- Adkison JB, Khuntia D, Bentzen SM, Cannon GM, Tome WA, Jaradat H, . Dose escalated, hypofractionated radiotherapy using helical tomotherapy for inoperable non-small cell lung cancer: Preliminary results of a risk-stratified phase I dose escalation study. Technol Cancer Res Treat 2008;7:441–7.
- Koukourakis M, Hlouverakis G, Kosma L, Skarlatos J, Damilakis J, Giatromanolaki A, . The impact of overall treatment time on the results of radiotherapy for non small cell lung carcinoma. Int J Radiat Oncol Biol Phys 1996;34:315–22.
- Knap MM, Hoffmann L, Nordsmark M, Vestergaard A. Daily cone-beam computed tomography used to determine tumour shrinkage and localisation in lung cancer patients. Acta Oncol 2010;49:1077–84.
- MacManus M, Nestle U, Rosenzweig KE, Carrio I, Messa C, Belohlavek O, . Use of PET and PET/CT for radiation therapy planning: IAEA expert report 2006–2007. Radiother Oncol 2009;91:85–94.
- Aerts HJWL, Bosmans G, van Baardwijk AAW, Dekker ALAJ, Oellers MC, Lambin P, . Stability of 18F-deoxyglucose uptake locations within tumor during radiotherapy for NSCLC: A prospective study: Int J Radiat Oncol Biol Phys 2008;71:1402–7.
- Sura S, Greco C, Gelblum D, Yorke ED, Jackson A, Rosenzweig KE. (18)F-fluorodeoxyglucose positron emission tomography-based assessment of local failure patterns in non-small-cell lung cancer treated with definitive radiotherapy. Int J Radiat Oncol Biol Phys 2008;70:1397–402.
- Aerts HJWL, Lambin P, Ruysscher DD. FDG for dose painting: A rational choice. Radiother Oncol 2010;97:163–4.
- Partridge M, Yamamoto T, Grau C, Høyer M, Muren LP. Imaging of normal lung, liver and parotid gland function for radiotherapy. Acta Oncol 2010;49:997–1011.
- Nyeng TB, Kallehauge JF, Høyer M, Petersen JBB, Poulsen PR, Muren LP. Clinical validation of a 4D-CT based method for lung ventilation measurement in phantoms and patients. Acta Oncol (in press).