Abstract
Background and purpose. Uncorrelated motion of targets and large deformations of organs at risk represent challenges for image-guidance in simultaneous integrated boost (SIB) radiotherapy (RT) of pelvic tumour sites. This study aims to evaluate the robustness towards geometrical uncertainties in prostate cancer using two image-guided RT (IGRT) set-up strategies for two SIB delivery methods. Secondly, we evaluate the ability of geometrical parameters to predict when the applied margins are insufficient, resulting in target underdosage (TUD). Material and methods. The study included nine patients with eight to nine repeat computed tomography (CT)-scans evenly distributed throughout their treatment course. The prostate target (CTV-p) and the lymph node target including seminal vesicles (CTV-ln/sv) were delineated in all scans. SIB treatment plans for intensity-modulated RT and volumetric modulated arc therapy were generated on the planning CT and transferred to the repeat CTs for dose re-calculation using registration based on either anatomy or intra-prostatic fiducial markers. Receiving operator characteristic analysis was used to deduce the ability of the parameters to predict TUD. Results. The dosimetric differences between the two positioning strategies were small for all parameters evaluated and significant only for the dose to rectum. Anatomy based registration resulted in inferior target coverage with a larger number of TUDs, mostly seen in the seminal vesicles. For both targets the highest sensitivity and specificity of predicting TUD was seen for the relative volume and the lowest was found for the displacement vector. Conclusions. Positioning based on fiducials gave the best trade-off between coverage of the targets although resulting in the highest dose to rectum. Target underdosage occurred mostly in the seminal vesicles. For both targets, the best parameter to predict TUD was the relative volume.
Modern radiotherapy (RT) with intensity modulated RT (IMRT) and volumetric modulated arc therapy (VMAT) enables highly conformal doses to targets and a greater sparing of the surrounding healthy tissue [Citation1,Citation2]. For localised prostate cancer, there is evidence of a dose-response relationship where higher doses lead to an increase in biochemical disease control [3-7]. Treating the pelvic lymph nodes in patients with locally advanced prostate cancer could in theory improve even further the outcome for these patients since the spread of disease usually involves the lymph nodes before more distant metastasis occur [Citation8,Citation12]. However, clinical studies investigating whole pelvic RT have shown ambiguous results [8-11]. A concern has been that irradiation of the pelvic lymph nodes leads to an increase in early and late normal tissue complications [9-12]. Here, the use of IMRT has reduced both the dose and the normal tissue toxicity as compared to three dimensional (3D)-conformal RT [Citation13,Citation14]. As the delivery of high doses is limited by the irradiation of normal tissue this calls for methods for accurate on-line localisation of the target before delivery of treatment; the latter being the main purpose of image-guided radiotherapy (IGRT) [Citation1,Citation15]. Schulze et al. investigated the impact of various IGRT strategies, ranging from rigid translations to re-optimisation, on IMRT and 3D-conformal RT [Citation15]. Besides finding IMRT to be more sensitive to organ motion, they also revealed that IGRT based on either fiducials or bony anatomy resulted in target coverage problems for the seminal vesicles further enhancing the problem with two independently moving structures [Citation15]. The effect of positioning based on fiducials for the lymph node target in repeat CT-scans has been investigated by Rossi et al., finding large differences between planned and delivered dose in 20% of the patients [Citation16]. Hence, they suggested that an adaptation of the lymph node planning target volume (PTV) could be made after acquiring data of prostate movement during delivery of the boost to prostate PTV [Citation16]. Another strategy is to deliver a simultaneously integrated boost (SIB) treatment to prostate and pelvic lymph nodes, which have shown to enable sparing of critical structures [Citation14]. However this will complicate the IGRT process further due to the independent movement of the involved targets [Citation17].
The main aim of this study was to evaluate the impact of target motion on IGRT set-up strategies for SIB treatments delivered with either IMRT or VMAT. Image-guidance was based on either intra-prostatic fiducials or on bony anatomy. Due to the uncorrelated motion and deformations of the two targets, image-guided approaches might be insufficient and treatment plan adaptations might be required. The second aim of this study was therefore to evaluate the ability of geometrical parameters to predict target underdosage (TUD), indicating the need of treatment plan adaptation.
Materials and methods
Nine patients previously treated with pelvic IMRT at Haukeland University Hospital (Bergen, Norway) during May to October in 2007 were included in the study. All patients had undergone a planning CT and eight to nine additional CT-scans evenly distributed throughout their treatment course, resulting in a total of 86 CT scans. Every scan was acquired with a slice thickness of 2–3 mm, covering L4 to the anus and with the patient in supine position using similar fixation devices as during treatment delivery. All patients had three fiducial gold markers implanted in the prostate.
Definition of target volumes
A radiation oncologist delineated the clinical target volume of the prostate (CTV-p), the pelvic lymph nodes and seminal vesicles CTV (CTV-ln/sv), the rectum and the bladder, in all CT-scans. The seminal vesicles and the pelvic lymph nodes were included in the same target since they received the same prescription dose. Further details of the definition of the targets and organs at risk (OR) have been described previously [Citation18]. Additionally, for the bowel we used the bowel cavity approach, with delineation from about 1 cm above PTV-ln/sv to the sigmoid. The CTV-p was expanded to form the PTV of prostate (PTV-p) by 7 mm in all directions except for in the superior-inferior (SI) direction where a 9 mm expansion was used. The CTV-ln/sv was expanded by 5 mm/8 mm (SI) to form PTV-ln/sv. The dose prescribed to PTV-p and PTV-ln/sv was 74 Gy and 55 Gy, respectively, given in 37 fractions using a SIB approach. All treatment plans were normalised to the mean dose of PTV-p.
Treatment planning
For each patient both an IMRT and a VMAT (RapidArc) plan were retrospectively constructed in Eclipse (ARIA ver.8.6, Varian Medical Systems, Palo Alto, CA, USA) using locally developed clinical templates. All treatment plans used photon beams with 15 MV beam quality shaped with 120-leaf millennium multileaf collimators (MLC). The IMRT and VMAT plans for each patient were made with the same isocentre. For the IMRT plans beams with gantry angles of 0°, 40°, 103°, 165°, 195°, 257° and 320° were used. RapidArc plans were generated with two arcs, one rotating from 179° counter clockwise to 181° and the other clockwise from 181° to 179°. Identical plan criteria including order of prioritisation () according to the Propel A + B trials were applied (see www.cirro.dk, CIRRO-IP080209 for further details). Similar dose-volume constraints were utilized for both SIB delivery techniques in order to satisfy these plan criteria.
Table I. The applied constraints including priorities used in the optimization of the treatment plans.
Repositioning and dose re-calculation
To simulate the different positioning strategies all CT-scans were rigidly registered based on intensities using the treatment planning system and manually inspected to match the bony anatomy. This strategy will hereafter be denoted as positioning based on anatomy. Positioning based on the intra-prostatic fiducials was achieved by segmenting the gold markers and finding their common centre-of-mass (COM). For the fiducial registration the translations from the COM to the isocentre was kept constant in each repeat CT-scan as compared to the treatment plan. The acquired MLC movements and monitor units (MU) from the treatment plan were transferred based on the two co-registration strategies to the repeat scans. Subsequently, dose calculations were performed for each scan keeping the MLC movements and MU constant.
Evaluation
Target underdosage was defined to occur when more than 1% of the CTV received less than 95% of the prescription dose, i.e. D99% < 95% of the prescription dose. In addition, for both targets and ORs the generalised equivalent uniform dose (gEUD) was calculated according to [Citation19,Citation20]:
The volume, V, is the total volume of the organ of interest and D is the dose. The index variable, i, summarise all dose-bins in the differential dose volume histogram (DVH). The dose-volume parameter a was set to 12 for rectum [Citation21], to 8 for the bladder [Citation1] and to -20 for both targets [Citation22]. For the targets, an a value of -20 gives a higher weighting for low doses [Citation22]. The positioning strategies and delivery techniques were compared with a Wilcoxon signed-rank test applying a significance level of 0.05. For both targets in each treatment plan the conformity index (CI) defined below was calculated [Citation23].
where VPTV is the volume of the PTV and PV is the volume enclosed by 95% of the prescribed dose. A value close to one corresponds to greater conformity [Citation23]. The resulting mean ± standard deviation (std) for the CI for PTV-p calculated for the primary/planning CT-scan was similar for IMRT (1.12 ± 0.10) and RapidArc (1.14 ± 0.11). For PTV-ln/sv the CI was slightly worse for the primary IMRT plans with a mean of 1.48 ± 0.05 as opposed to 1.43 ± 0.04 for RapidArc. For the nine patients the average MU was more than doubled for the IMRT plans (980 ± 23 MU) as compared to the RapidArc plans (461 ± 33 MU).
As for the ability to predict TUD three parameters were explored: 1) The relative volumetric change of the target volumes in the repeat scans as compared to those in the planning CT; 2) The displacement vector defined as the difference in distance derived from the two positioning techniques (Equation 3); 3) The Dice similarity coefficient (DSC) [Citation24] (Equation 4), a volumetric parameter including information about spatial overlap.
Displacement vector,
In Equation 4, Vplan indicates the CTV as delineated in the planning CT and Vregistration is the CTV transferred to the planning CT after registration based on either fiducials or anatomy. Receiving operating characteristic (ROC) analysis was used to compare the parameters, determining the area under the curve (AUC) [Citation25]. The ROC curves were constructed by varying thresholds based on data of the three parameters and by calculating the sensitivity and specificity in identifying TUD for each threshold. No fitting of the ROC curves was used.
Results
The two positioning strategies, fiducials versus anatomy-based, had the largest deviations in the anterior-posterior (AP) direction (, left). The displacements (mean ± std) was 0.0 ± 0.1 cm in the left-right (LR) direction, 0.0 ± 0.2 cm in the SI direction and 0.0 ± 0.3 cm in the AP direction (, right). The mean ± std for the displacement vector was 0.3 ± 0.2 cm.
Figure 1. Displacements comparing fiducial to anatomy-based shifts displayed as the displacement vector with the circle marking the isocentre (left) and for each anatomical direction along with the observed frequency (right).
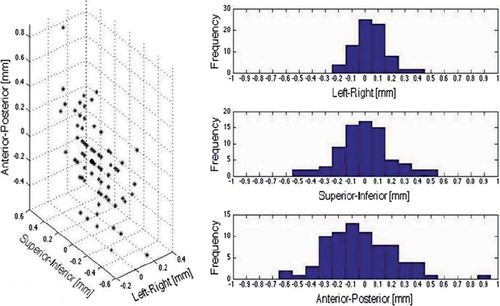
Target coverage
In the majority of patients the treatment-average D99% and gEUD for CTV-p differed less than 1 Gy and 0.5 Gy, respectively, to the plan (, left). Hence, no significant differences were seen for these two dose parameters when comparing the two positioning strategies, irrespective of delivery technique. As a consequence, all patients had a treatment-average D99% >95% of 74 Gy and TUDs occurred in only two patients ( and ). For one patient this underdosage was in the posterior part of CTV-p but for the other patient it was located cranially and in the anterior part of CTV-p.
Figure 2. D99 as a function of gEUD for both targets CTV-p (left column) and CTV-ln/sv (right column) and SIB technique with IMRT (top row) and VMAT (bottom row) Crosses denote fiducial based registration and circles denote anatomy based registration.
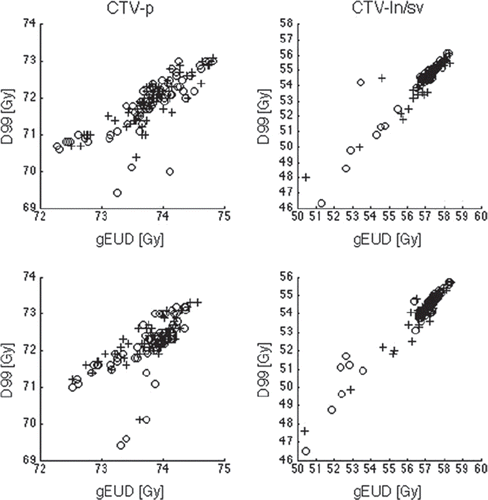
Table II. Mean of patient-average target D99% along with range of patient average in brackets, as well as the fraction of the scans where TUD was observed.
Table III. Mean of patient-average gEUD along with range of patient average in brackets for both targets and ORs.
For the CTV-ln/sv target, the dosimetric deviations were greater than seen for CTV-p, although not significantly different when comparing the two positioning strategies. For this target, the greatest decrease in delivered vs. planned D99 and gEUD were obtained when positioning was based on anatomy as compared to fiducials (). This was also reflected in a larger number of TUDs with anatomy based registration for both delivery techniques (). The TUDs were seen for the same patient and located in the seminal vesicles. This patient had the largest decrease in patient treatment-average gEUD versus planned for CTV-ln/sv; 5.9 Gy with VMAT and 3.8 Gy with IMRT, both obtained using anatomy based registration. Corresponding values for D99 was 4.8 Gy with VMAT and 4.3 Gy with IMRT.
The impact of delivery technique for the same set-up strategy revealed larger differences both in mean patient-average D99 and gEUD as compared to changing the set-up strategy using the same delivery technique ( and ). However, the differences were modest and only statistically significant when evaluating D99. For CTV-p a higher D99 were obtained with VMAT as compared to IMRT (p < 0.001) as oppose to CTV-ln/sv where a higher D99 was obtained using VMAT (p < 0.001). This was observed independent of positioning strategy.
Predictive ability of the parameters
The ROC analysis showed high sensitivity and specificity of the parameters for CTV-p. When positioning was based on anatomy, the AUC was 0.97 for relative volume, 0.93 for DSC and 0.72 for the displacement vector (, left). As only one TUD was seen for CTV-p when positioning was based on fiducials this was not included in the ROC analysis. Lower AUC-values were observed for CTV-ln/sv; 0.80 for the relative volume, 0.66 for DSC and 0.60 for the displacement vector with positioning based on fiducials (, right). The AUC for CTV-ln/sv with anatomy-based registration were similar to those obtained for fidicuals; 0.77 for relative volume, 0.74 for DSC and 0.63 for the displacement vector. The ROC analysis was considered for VMAT only since this technique resulted in a greater number of TUDs as compared to IMRT.
Dose to rectum and bladder
For both rectum and bladder, the planned gEUD were similar to the treatment-average gEUD with the mean patient-average deviation being less than 1 Gy. Rectum was the only organ where a significant difference from the choice of positioning strategy was observed. For this OR the highest treatment gEUD was seen when positioning was based on fiducials and significantly different from anatomy-based for both IMRT (p = 0.040) and VMAT (p = 0.046) (). In addition, positioning based on fiducials gave a higher patient-average treatment gEUD than planned for the rectum (). For the bladder, a wider range in patient-average gEUD was seen as compared to rectum. Hence, this OR displayed the greatest difference between planned and treatment-average gEUD across all patients; a reduction of 8.5 Gy with IMRT and 8.0 Gy with VMAT, both seen for fiducial-based registration.
The comparison between delivery techniques while utilising the same positioning strategy showed significantly higher treatment rectum gEUD with IMRT both for ficucial- (p < 0.001) and anatomy-based (p < 0.001) positioning. For the bladder a significant difference was only found for ficucial-based registration (p = 0.02) with higher treatment gEUD seen for IMRT ().
Discussion
This study compared two different IGRT positioning strategies for patients having independently moving targets, i.e. prostate and lymph nodes. In general, the greatest difference in planned versus delivered target dose was observed for the lymph node target, and the difference was larger when positioning was based on anatomy as compared to fiducials. Positioning based on fiducials was expected to give fewer TUDs for the prostate target and this was confirmed by the study. However, with the applied margins for the prostate target even positioning based on anatomy resulted in very few TUDs, independently of delivery technique. These TUDs occurred in two patients and was accompanied by a reduced dose to rectum in one patient and to a reduced dose in the bladder in the other. In contrary to the TUDs for CTV-p resulting in a reduction of dose to the ORs, the TUDs for CTV-ln/sv corresponded to the highest treatment-average gEUD to the bladder amongst the patients.
The consequences of positioning based on bony anatomy versus other IGRT strategies have previously been addressed for RT including both the prostate and seminal vesicles [Citation15,Citation26]. Both studies investigated IMRT plans, one using a repeat CT data set and a uniform margin of 1 cm around the CTV-p whereas the other used CBCT-scans and a margin around CTV-p of 1.0 cm / 0.7 cm (posterior) [Citation15,Citation26]. Their findings of inter-patient variations and difficulties in obtaining proper coverage of the seminal vesicles are consistent with the findings of our study. Partly attributable to a strict definition of TUD, Thongphiew et al. found 43% of the sessions to be unsuccessful [Citation26]. This is a larger fraction than the 4% obtained in our study for CTV-p or 12% if adding the TUDs seen in the seminal vesicles. Additionally, as in our study some TUDs resulted in a reduced dose to the rectum [Citation26].
The consequences of the dose to the pelvic lymph node target using fiducial-based positioning have previously been investigated for IMRT treatments [Citation16]. With margins of 0.5–1.5 cm around CTV-ln/sv these investigators found large deviations in the minimum dose to PTV-ln/sv for two of 10 patients [Citation16]. This finding was explained by the large displacements of the prostate relative to bony anatomy of > 1 cm in the SI direction seen in this patient material [Citation16]. These displacements can be contrasted to our maximum deviation of 0.5 cm in this direction which thereby would be covered by our margins. The displacements found in our study are in good agreement with other studies [Citation27,Citation28]. Like our study Zelefsky et al. also obtained larger displacements in the AP-direction but found the prostate COM to be shifted more posteriorly [Citation28]. These authors also quantified the seminal vesicle displacement and found it to be larger than for the prostate, particularly in the SI-direction [Citation28]. Our margins of 5 mm/8 mm (SI) enclosing CTV-ln/sv did not cover the seminal vesicles part of this target for one patient with either set-up strategy. The reason being a distended rectum, pushing the seminal vesicles towards the bladder in the planning CT unlike in the repeat scans. Consequently, this resulted in TUDs and a reduction of treatment-average gEUD for the CTV-ln/sv as well as an elevated dose to the bladder as compared to the plan. Using bony anatomy for registration, margins up to 1 cm have been shown to be inadequate for the seminal vesicles, in particular for patients having a large rectum and bladder in the planning CT [Citation15,Citation26,Citation28]. Even including seminal vesicles in the more mobile CTV-p and registration based on fiducials have revealed difficulties with dose coverage [Citation29,Citation30]. Suggested margins around seminal vesicles have varied from a minimum of 0.45 cm to larger than 0.8 cm [Citation29,Citation30]. Consequently, due to the larger margins around CTV-p, the number of TUDs for each co-registration seen in our results would probably been altered by including the seminal vesicles within the CTV-p. However, as the TUDs were seen in proximity to the rectum an increased coverage of the seminal vesicles might have resulted in an increased dose to the rectum, which barely met the planning criteria for the patient of interest.
Averaged over an entire course of treatment, TUD in a low fraction of the sessions might not necessarily influence the accumulated dose to the target. However, this study can be seen to illustrate a hypo-fractionated setting where the positioning and adaptations become increasingly important. In such a situation the ability to predict TUD is of great value as guidance for when to re-optimise the treatment plan. The ROC analyses were used with a D99 based endpoint but a biologically relevant endpoint either for the target or for the ORs could also be applied in such an analysis. However, we chose the most sensitive endpoint, which was found to be linearly associated to gEUD (). In the ROC analyses for CTV-p, DSC closely followed the curve for the relative volume but for CTV-ln/sv the DSC curve was closer to the displacement vector. This could be a consequence of the TUDs being found in the seminal vesicles; as this structure occupies only a relatively small volume of the entire CTV the corresponding differences in DSC values are small. Similarly, this can also explain the poorer AUC values for the relative volume obtained for this target. Other, more complex parameters describing the distance from the surface of the target after registrations might be more powerful in predicting TUD.
The results of this study reflect the influence of different positioning strategies for the clinical templates used for planning in our clinic. Other constraints and templates would obviously alter the results. However, all treatment plans were made by the same planner to avoid unnecessary variations. Both delivery techniques had similar CI and were significantly different only in planned gEUD for CTV-p (p = 0.004) and rectum (p = 0.008). Another effect that we have neglected in our analysis is intra-fractional motion, which would further blur the accumulated dose and reduce the efforts of the IGRT positioning strategy [Citation31]. Here VMAT should have an advantage since the true value of this technique is its reduced beam delivery time in comparison to IMRT, thereby reducing the influence of intra-fractional motion [Citation2,Citation23,Citation32,Citation33]. Additionally, the two positioning strategies, anatomy and fiducials, can be considered as two extremes for each target. Another type of rigid registration and matching of the true CTV-p instead of substituted by the fiducials might have altered the results for the displacements [Citation34]. Moseley et al. compared the shift derived from soft-tissue matching of prostate CTV to fiducial-based matching [Citation35]. They obtained displacements within 0.2 cm from our values [Citation35]. However, as already stated the displacements obtained in our study are similar to other studies so the alterations of the results using our margins are probably negligible. On the other hand, another study showed that a CT to CBCT soft-tissue match acquired from deformable image registration (DIR) yielded less TUDs, from 43% using bony anatomy to 27% with DIR [Citation26]. Hence, this registration thereby made it possible to better account for the often complex changes in patient anatomy. However, we have previously investigated the performance of a DIR application and found it inadequate in terms of contour propagation for rectum and bladder [Citation18]. Instead, for this study we chose to manually delineate both target volumes and ORs in all CT-scans. Although all contours were delineated systematically and by the same radiation oncologist in both the planning and the repeat CT-scans, it will introduce an uncertainty to our results as variations in contouring for pelvic RT have been documented [Citation36]. This uncertainty will probably influence the ROC analysis the most.
In conclusion, this study has shown that with the applied margins the selection of positioning strategy has only slight influence on the target coverage. However, positioning based on fiducials, although resulting in slightly higher doses to rectum, gave the best trade-off between adequate target coverage for CTV-p and CTV-ln/sv. This finding was consistent for both IMRT- and VMAT-plans. Target underdosage occurred mostly in the seminal vesicles and the best parameter to predict any TUD was the relative volume.
Acknowledgements
This work has been supported by research grants from CIRRO – The Lundbeck Foundation Center for Interventional Research in Radiation Oncology.
Declaration of interest: The authors report no conflicts of interest. The authors alone are responsible for the content and writing of the paper.
References
- Ghilezan M, Yan D, Liang J, Jaffray D, Wong J, Martinez A. Online image-guided intensity-modulated radiotherapy for prostate cancer: How much improvement can we expect? A theoretical assessment of clinical benefit and potential of dose escalation by improving precision and accuracy of radiation therapy. Int J Radiat Oncol Biol Phys 2004;60: 1602–10.
- Otto K. Volumetric modulated arc therapy: IMRT in a single gantry arc. Med Phys 2008;35:310–7.
- Kuban DA, Tucker SL, Dong L, Starkschall G, Huang EH, Cheung MR, . Long-term results of the M. D. Anderson randomized dose escalation trial for prostate cancer. Int J Radiat Oncol Biol Phys 2008;70:67–74.
- Zelefsky MJ, Yamada Y, Fuks Z, Zhang Z, Hunt M, Cahlon O, . Long-term results of conformal radiotherapy for prostate cancer: Impact of dose escalation on biochemical tumor control and distant metastases-free survival outcomes. Int J Radiat Oncol Biol Phys 2008;71:1028–33.
- Dearnaley DP, Sydes MR, Graham JD, Aird EG, Bottomley D, Cowan RA, . Escalated-dose versus standard-dose conformal radiotherapy in prostate cancer: First results from the MRC RT01 randomised controlled trial. Lancet Oncol 2007;8:475–87.
- Ganswindt U, Paulsen F, Anastasiadis AG, Stenzl A, Bamberg M, Belka C. 70 Gy of more: Which dose for which prostate cancer? J Cancer Res Clin Oncol 2005; 131:407–19.
- Karlsdottir A, Muren LP, Wentzel-Larsen T, Johannessen D, Haukaas SA, Halvorsen OJ, . Outcome in intermediate or high-risk prostate cancer patients receiving radiation dose and hormone therapy. Acta Oncol 2009;48:874–81.
- Wang D, Lawton C. Pelvic lymph node irradiation for prostate cancer: Who, why, and when. Semin Radiat Oncol 2008;18:35–40.
- Pommier P, Chabaud S, Lagrange JL, Richaud P, Lesaunier F, Le Prise E, . Is there a role for pelvic irradiation in localized prostate adenocarcinoma? Preliminary results of GETUG-01. J Clin Oncol 2007;25:5366–73.
- Lawton CA, DeSilvio M, Roach M, . An update of the phase III trail comparing whole pelvic to prostate only radiotherapy and neoadjuvant to adjuvant total andraogen suppression: Updated analysis of RTOG 94-13, with emphasis on unexpected hormone/radiation interactions. Int J Radiat Oncol Biol Phys 2007;69:646–55.
- Roach M, DeSilvio M, Valicenti R, Uhl V, Machtay M, Seider MJ, . Whole-pelvis, “Mini-pelvis”, or prostate-only external beam radiotherapy after neoadjuvant and concurrent hormonal therapy in patients treated in the radiation therapy oncology group 9413 trail. Int J Radiat Oncol Biol Phys 2006;66:647–53.
- Bayley A, Rosewall T, Craig T, Bristow R, Chung P, Gospodaro-wicz M, . Clinical application of high-dose, image-guided intensity-modulated radiotherapy in high-risk prostate cancer, Int J Oncol Biol Phys 2010;77:477–83.
- Ashman J, Zelefsky M, Hunt M, Leibel S, Fuks Z. Whole pelvic radiotherapy for prostate cancer using 3D conformal and intensity-modulated radiotherapy. Int J Radiat Oncol Biol Phys 2005;63:765–71.
- Li AX, Wang JZ, Jursinic PA, Lawton CA, Wang D. Dosimetric advantage of IMRT simultaneous integrated boost for high-risk prostate cancer. Int J Radiat Oncol Biol Phys 2005;61:1251–7.
- Schulze D, Liang J, Yan D, Zhang T. Comparison of various IGRT strategies: The benefit of online treatment plan re-optimization. Radiother Oncol 2009;90:367–76.
- Rossi P, Schreibmann E, Jani A, Master V, Johnstone P. Boost first, eliminate systematic error, and individualize CTV to PTV margin when treating lymph nodes in high-risk prostate cancer. Radiother Oncol 2009;90:353–8.
- Xia P, Hwang A, Kinsey E, Pouliot J, Roach M. Comparison of three strategies in management of independent movement of the prostate and pelvic lymph nodes. Med Phys 2010;37: 5006–13.
- Thörnqvist S, Petersen JB, Høyer M, Bentzen L, Muren LP. Propagation of target and organ at risk contours in radiotherapy of prostate cancer using deformable image registration. Acta Oncol 2010;49:1023–32.
- Niemierko A. Reporting and analyzing dose distributions: A concept of equivalent uniform dose. Med Phys 1997;24: 103–10.
- Niemierko A. A generalized concept of equivalent uniform dose. Med Phys 1999;26:1000. Abstract.
- Söhn M, Yan D, Liang J, Meldolesi E, Vargas C, Alber M. Incidence of late rectal bleeding in high-dose conformal radiotherapy of prostate cancer using equivalent uniform dose-based and dose-volume based normal tissue complication probability models. Int J Radiat Oncol Biol Phys 2007; 67:1066–73.
- Søvik Å, Ovrum J, Olsen DR, Malinen E. On the parameter describing the generalised equivalent uniform dose (gEUD) for tumours. Phys Med 2002;23:100–6.
- Yoo S, Wu JQ, Lee RE, Fang-Fang Y. Radiotherapy treatment plans with RapidArc for prostate cancer involving seminal vesicles and lymph nodes. Int J Oncol Biol Phys 2010;76: 935–42.
- Dice LR. Measures of the amount of ecologic association between species. Ecology 1945;26:297–302.
- Eng J. Receiver operating characteristic analysis: A primer. Acad Radiol 2005;12:909–16.
- Thongpheiw D, Wu J, Lee RW, Chankong V, Yoo S, McMahon R, . Comparison of online IGRT techniques for prostate IMRT treatment: Adaptive vs repositioning correction. Med Phys 2009;36:1651–62.
- Pawlowski JM, Yang ES, Malcolm AW, Coffey CW, Ding GX. Reduction of dose delivered to organs at risk in prostate cancer patients via image-guided radiation therapy. Int J Radiat Oncol Biol Phys 2010;76:924–34.
- Zelefsky M, Crean D, Margeras GS, . Quantification and predictors of prostate position variability in 50 patients evaluated with multiple CT scans during conformal radiotherapy. Radiother Oncol 1999;50:225–34.
- Liang J, Wu Q, Yan D. The role of seminal vesicle motion in target margin assessment for online image-guided radiotherapy for prostate cancer. Int J Radiat Oncol Biol Phys 2009;73:935–43.
- Mutanga T, de Boer H, van der Wielen G, Hoogeman M, Incrocci L, Heijmen B. Margin evaluation in the presence of deformation, rotation, and translation in prostate and entire seminal vesicle irradiation with daily marker-based setup corrections. Int J Radiat Oncol Biol Phys 2010 (in press).
- Budiharto T, Slagmolen P, Haustermans K, Maes F, Junius S, Verstraete J, . Intrafractional prostate motion during online image guided intensity-modulated radiotherapy for prostate cancer. Radiother Oncol 2011;98:181–6.
- Kristoffersen F, . Rotational radiotherapy for prostate cancer in clinical practice. Radiother Oncol 2010;97:480–4.
- Kjær-Kristoffersen F, Ohlheus L, Medin J, Korreman S. RapidArc volumetric modulated therapy for prostate cancer patients. Acta Oncol 2009;48:227–32.
- Waldenström A-C, Alsadius D, Pettersson N, Johansson K-A, Holmberg E, Steineck G. Variation of position and volume of organs at risk in the small pelvis. Acta Oncol 2010;49: 491–9.
- Moseley DJ, White EA, Wiltshire KL, Rosewall T, Sharpe MB, Siewerdsen JN, . Comparison of localization performance with implanted fiducial markers and cone-beam computed tomography for on-line image-guided radiotherapy of the prostate. Int J Radiat Oncol Biol Phys 2007;67: 942–53.
- Fiorino C, Reni M, Bolognesi A, Cattaneo GM, Clandrino R. Intra- and inter-observer variability in contouring prostate and seminal vesicles: Implications for conformal treatment planning. Radiother Oncol 1998;47:285–92.