Abstract
Background. Stereotactic body radiotherapy (SBRT) is often the preferred treatment for the advanced liver tumours which owing to tumour distribution, size and multi-focality are out of range of surgical resection or radiofrequency ablation. However, only a minority of patients with liver tumours may be candidates for conventional SBRT because of the limited radiation tolerance of normal liver, intestine and other normal tissues. Due to the favourable depth-dose characteristics of protons, intensity-modulated proton therapy (IMPT) may be a superior alternative to photon-based SBRT. The purpose of this treatment planning study was therefore to investigate the potential sparing of normal liver by IMPT compared to photon-based intensity-modulated radiotherapy (IMRT) for solitary liver tumours. Material and methods. Ten patients with solitary liver metastasis treated at our institution with multi-field SBRT were retrospectively re-planned with IMRT and proton pencil beam scanning techniques. For the proton plans, two to three coplanar fields were used in contrast to five to six coplanar and non-coplanar photon fields. The same planning objectives were used for both techniques. A risk adapted dose prescription to the PTV surface of 12.5–16.75 Gy × 3 was used. Results. The spared liver volume for IMPT was higher compared to IMRT in all 10 patients. At the highest prescription dose level, the median liver volume receiving less than 15 Gy was 1411 cm3 for IMPT and 955 cm3 for IMRT (p < 0.005); also the mean liver dose was lower with IMPT compared to IMRT (median 9.1 Gy vs. 20.0 Gy; p < 0.005). All IMPT and IMRT plans met the VD < 15 Gy > 700 cm3 constraint. For the Dmean ≤ 15 Gy constraint, nine of 10 cases could be treated at the highest dose level using IMPT whereas with IMRT, only two cases met this constraint at the highest dose level and six at the lowest dose level. Conclusion. A considerable sparing of normal liver tissue can be obtained using proton-based SBRT for solitary liver tumours.
Surgical resection is the preferred therapy for primary liver tumours and oligo-liver metastases [Citation1]. However, only a relatively low proportion of patients is amendable for resection due to technical and medical conditions. Stereotactic body radiation therapy (SBRT) is useful for ablation of liver tumours and may be an alternative for patients who are not suitable for surgery [Citation2]. SBRT is a highly conformal and precise treatment where a large radiation dose is delivered to the liver lesions over few fractions [Citation3]. This method is increasingly used in treatment of both primary and secondary liver cancer. A feared complication following liver SBRT is the development of radiation-induced liver disease (RILD) [Citation2,Citation4–6], and selection of patients and dose prescription are often relatively conservative due to the risk of RILD [Citation7].
Intensity-modulated proton therapy (IMPT) may have an advantage in the management of liver tumours due to the favourable depth-dose characteristics of protons as well as the possibilities of intensity-modulated planning and delivery, potentially opening for normal liver tissue sparing. We have therefore performed a treatment planning study comparing IMPT and photon-based intensity-modulated radiotherapy (IMRT) based on SBRT principles with high-precision hypo-fractionated radiotherapy to patients with fairly large solitary liver metastases. The purpose of the study was to investigate the advantage of protons over photons focusing specifically on the liver as the primary organ at risk (OAR).
Materials and methods
Patients who had received SBRT for solitary liver metastases were eligible for inclusion in the present study. They were all immobilised in a SBRT frame (SBF; Elekta, Stockholm, Sweden). An abdominal compression device mounted on the SBF was used to limit internal respiration-related motion-amplitude of the tumour (or diaphragm as a surrogate) to less than 10 mm. Treatment planning and delivery were overall performed as described in a previous report [Citation2]. Planning was based on a contrast-enhanced CT-scan acquired after bolus injection of 150 ml Visipaque 275 with a delay of 70 s. The CT-scan was reconstructed with a 3 mm inter-slice distance. The clinical target volume (CTV) was defined as the hypo-dense tumour, including also any uncertain tumour volume on the contrast-enhanced CT-scan. A standard margin of 5 mm in the transversal plane and 10 mm in the cranio-caudal direction was added to the CTV to form the planning target volume (PTV). OARs such as the liver, duodenum, stomach, intestine, kidneys, lungs, heart and spinal cord were contoured.
Coplanar as well as non-coplanar beam arrangements were used in treatment planning. For IMRT planning, five to six beams (all 6 MV beam quality) from optimal directions were used. For IMPT planning, two to three coplanar beams were chosen based on the same geometrical considerations. IMPT planning was performed using the Eclipse planning system (v10.0, Varian Medical Systems, Palo Alto, CA, USA) using a default machine and proton beam data. A pencil beam scanning technique with multi-field optimisation was used. The constraints used for IMRT and IMPT optimisation to CTV, PTV and OARs are listed in . We used a risk adapted strategy for dose prescription aiming at the highest prescription dose (levels A–D) where the constraints to OARs could still be met. Using a three-fraction schedule, prescribed doses per fraction were: A: 12.50 Gy, B: 13.75 Gy, C: 15.00 Gy and D: 16.75 Gy. Doses were prescribed at the PTV surface with an inhomogeneous dose distribution aiming at a central dose of 150%. Fourteen patients with liver metastases who all had received SBRT with prescription doses ranging from 30–37.5 Gy (prescribed to the PTV surface) were considered for inclusion in the present study. In four patients one or more of the constraints could not be met, most often this was due to exposure of the duodenum or stomach in close vicinity to the target. After exclusion of these cases, 10 patients were left for this analysis. For these patients, it was possible to make IMPT and IMRT plans in all cases at the highest prescription dose level (D: 3 × 16.75 Gy) without compromising the dose constraints to the OARs.
Table I. Constraints to targets and organs at risk.
Endpoints for this analysis were volume of normal liver (i.e. excluding the CTV) spared to a dose of 15 Gy (VD < 15 Gy) as well as the mean dose to the normal liver (Dmean). The non-parametric Wilcoxon rank test for paired samples was used for analysis of differences between IMPT and IMRT plans.
Results
The 10 included patients had fairly large solitary metastases with a median CTV of 70 cm3 (range 25–232 cm3). In all cases at least 99% of the PTV received the prescription dose and 99% of the CTV received 142.5% of the prescription dose. The dose distributions for the IMRT and IMPT plans for a typical patient with a central liver metastasis are shown in .
Figure 1. Patient with solitary liver metastasis (CTV = 84 cm3) planned with IMRT (left) and IMPT (right). Threshold for dose colour wash is 15 Gy. Red and purple lines indicate CTV and PTV, respectively.
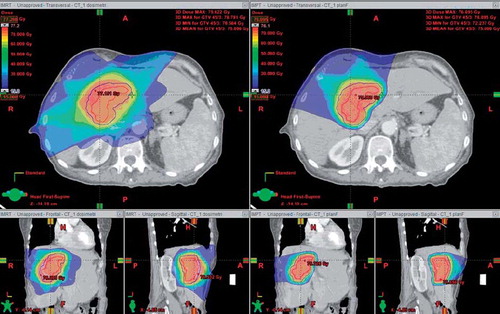
IMPT was superior to IMRT when evaluating the integral dose to the liver and the sparing effect of protons was most pronounced at the lower doses (). For all cases, the spared liver volume (VD < 15 Gy) for IMPT plans were higher compared to IMRT plans, even at the highest dose level D (): the median VD < 15 Gy was 1411 cm3 for IMPT and 955 cm3 for IMRT (p < 0.005). However, all patients met the primary constraint to the liver, VD < 15 Gy of minimum 700 cm3. As seen in , the liver Dmean at the highest dose level D was considerably lower in IMPT plans than in IMRT plans with median values of 9.1 Gy and 20.0 Gy, respectively (p < 0.005). At the highest dose level D, the Dmean ≤ 15 Gy was met in nine of the 10 cases with IMPT while for dose levels A–C the criteria was met for all patients. For IMRT plans, the Dmean ≤ 15 Gy criteria was met in two cases for dose level D, two cases for level C, four cases for level B and 10 cases for level A.
Figure 2. Mean DVHs for normal liver (excluding CTV) for 10 patients with solitary liver metastasis planned with IMRT and IMPT. The prescribed dose for both plans was 16.75 Gy × 3 (level D).
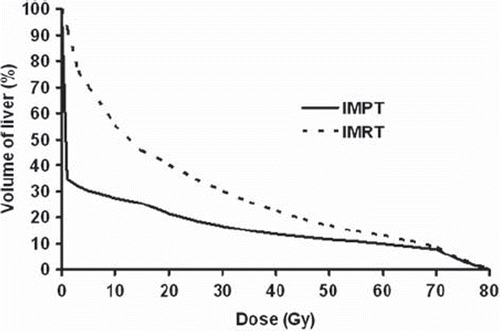
Discussion
During the last decade there has been an increasing use of radiotherapy, most often by means of SBRT, for focal therapy of liver tumours [Citation8]. Phase I–II studies on SBRT of liver tumours have shown that the treatment is highly efficient and results in local control rates [Citation2,Citation6,Citation9,Citation10] which are comparable to those achieved by radiofrequency ablation [Citation11]. Furthermore, SBRT can be delivered with moderate toxicity. There are, however, reports of cases with severe toxicity and even fatal events [Citation2,Citation6]. These are primarily related to RILD causing liver failure.
A recent analysis of large retrospective cohorts of patients receiving SBRT for a variety of lung and liver tumours demonstrated a clear dose-response relationship for local control [Citation12]. The study found that lesions treated to a nominal dose of 54 Gy or greater to the periphery of the PTV in a three-fraction schedule had a three-year actuarial local control rate of 89% compared with 59% for those treated to 36–53.9 Gy and 8% for those receiving less than 36 Gy. It is therefore strongly advisable to aim at doses of 54 Gy and not to go below 36 Gy, whenever possible, in a three-fraction SBRT schedule for lung and liver tumours. However, with three or more lesions and lesion size up to 6 cm in diameter these doses may not always be achievable.
The observed dose-response effect in SBRT calls for methodologies which at the same time allow increased the radiation dose to the target and low doses to the normal tissues. Due to the favourable depth-dose characteristics, protons may be ideal in treatment of liver tumours. The results of the present study strongly indicate that protons are superior to photons in SBRT for large solitary liver metastases. In a risk adapted dose prescription approach the target dose could be escalated successfully by IMPT to the second highest dose level in all 10 cases and in nine of 10 cases to the highest dose level, without exceeding a Dmean of 15 Gy. By IMRT planning this was only possible in two of 10 cases, and even at the lowest dose level, IMRT failed in four cases. The constraint on spared volume of liver (VD < 15 Gy > 700 cm3; CTV excluded) was met in all cases at the highest dose level, both for IMPT and IMRT plans.
The favourable depth-dose characteristics of proton beams often make proton plans less robust to anatomical/geometrical changes [Citation13]. The liver is a mobile and deformable organ and the shape, size and gas content of the stomach, intestine, and colon may vary from day to day [Citation14]. It should therefore be pointed out that the results obtained in this study are based on an ideal, ‘static geometry’ scenario. There are no established concepts for margins in proton therapy and it was therefore decided to use similar margins for the IMRT and IMPT plans. Methods to increase robustness, initially by simple margin expansion approaches, should be explored. Yet it seems likely that the considerable advantages with protons observed in this study would remain also if motion/geometrical uncertainties (and wider margins) had been considered.
Dose-volume constraints to the liver and the intestine often define the limitations for dose- escalation in SBRT for liver metastases. A modelling study by Dawson et al. based on the Lyman-Kutcher-Burman normal tissue complication probability (NTCP) model showed that the liver has a pronounced volume effect (n close to 1.00) [Citation15]. The study also estimated a TD50 of 46 Gy for normal liver and 40 Gy for liver in patients with an underlying liver disease such as hepatitis B-carriers (HBV) as well as in patients with liver cirrhosis who seem to have lower tolerance to radiation doses than patients with healthy liver [Citation15]. It is generally accepted that only Child Pugh (CP) A and B patients can be offered SBRT, and that CP B patients should be treated with extreme caution [Citation6]. However, primary liver cancer occurs frequently in patients with hepatitis or cirrhosis. Due to these co-morbidities these patients are often inoperable for medical reasons, they seem to increase in numbers and they pose a major therapeutic challenge.
The recent Quantitive Analyses of Normal Tissue Effects in the Clinic (QUANTEC) initiative was established with the aims to describe dose-response and dose-volume relationships for radiation toxicities in a number of normal tissues and to give guidelines for constraints in RT planning [Citation7,Citation16]. The dedicated QUANTEC liver paper concluded that the liver has a highly pronounced volume effect [Citation7]. Primarily based on experience from surgical resection of liver tumours, the liver QUANTEC study recommended that at least 700 cm3 of normal liver (CTV excluded) is limited to a dose of 15 Gy (VD < 15 Gy ≥ 700 cm3) in a three-fraction schedule. Furthermore, it is also recommended to keep the Dmean below 15 Gy in normal liver (CTV excluded) and below 12 Gy in HBV carriers and patients with cirrhosis. With these constraints the risk for RILD is expected to be less than 5%. Endpoints defined by VD < 15 Gy ≥ 700 cm3 and Dmean 15 Gy were used in the present study.
In a planning study by Wang et al. comparing treatment plans of IMRT and proton-based RT using standard fractionation with doses of 45–68.4 Gy in nine patients with liver tumours, proton therapy resulted in improved conformality and reduced V30 Gy and mean liver dose compared to IMRT; besides, also the doses to the stomach and duodenum could be reduced [Citation17]. The Dmean to the liver by IMRT was 25.1 Gy and this was reduced by approximately 25% to 17.5 Gy by use of protons. As indicated in this reduction was even more pronounced when comparing IMPT and IMRT in the present study on SBRT of relatively large solitary liver metastases.
A growing number of phase II studies and retrospective cohort studies on moderately hypo-fractionated proton therapy for hepatocellular carcinoma (HCC) are being reported. A phase II study on 76 patients treated for HCC with 63 Gy in 15 fractions at the Loma Linda University Medical Center has recently been reported [Citation18]. In this study the median progression-free survival was 36 months and the three-year progression-free survival rate was 60%. Eighteen patients subsequently underwent liver transplantation; in six explants there was pathological complete response and in seven there was only microscopic residual [Citation18]. Similar results was found in a phase II study from the Chiba Oncology Center where two-year local control and survival rates were 96% and 66%, respectively [Citation19]. In an analysis of a large cohort of 318 patients treated with a median dose of 72.6 Gy over a median number of 22 fractions at the Tsukuba University, a survival rate of 65% at three years was reported, with even more favourable outcome in CP A patients [Citation20]. The same group reported very promising outcome in patients with very large HCC (larger than 10 cm) with two-year overall and progression-free survival rates of 36% and 24%, respectively [Citation21] and favourable outcome even in patients with portal vein thrombosis which is considered to be associated with poor prognosis [Citation22]. In all studies, the treatment was well tolerated and no severe complications and cases of RILD were reported. A few patients experienced gastro-intestinal toxicity, and all were conservatively managed.
In conclusion, the present treatment planning study comparing IMPT and IMRT for SBRT of fairly large solitary liver metastasis showed that the most favourable treatment plans could be obtained by IMPT when considering the liver to be the most important critical organ. The study strongly indicates that IMPT may allow dose-escalation and result in improved outcome in SBRT for liver tumours. Ablation of primary liver cancer and liver metastases may therefore be important future indications for proton therapy.
Acknowledgements
Supported by CIRRO – The Lundbeck Foundation Center for Interventional Research in Radiation Oncology, The Danish Council for Strategic Research, and Varian Medical Systems (Palo Alto, CA, USA). Morten Høyer is supported by a grant from the A.P. Møller og hustru Chastine Mc-Kinney Møllers Fond til almene Formaal.
Declaration of interest: The authors report no conflicts of interest. The authors alone are responsible for the content and writing of the paper.
References
- Simmonds PC, Primrose JN, Colquitt JL, Garden OJ, Poston GJ, Rees M. Surgical resection of hepatic metastases from colorectal cancer: A systematic review of published studies. Br J Cancer 2006;94:982–99.
- Hoyer M, Roed H, Traberg HA, Ohlhuis L, Petersen J, Nellemann H, . Phase II study on stereotactic body radiotherapy of colorectal metastases. Acta Oncol 2006;45: 823–30.
- Lax I, Blomgren H, Naslund I, Svanstrom R. Stereotactic radiotherapy of malignancies in the abdomen. Methodological aspects. Acta Oncol 1994;33:677–83.
- Dawson LA, Ten Haken RK. Partial volume tolerance of the liver to radiation 1. Semin Radiat Oncol 2005;15:279–83.
- Dawson LA, Eccles C, Craig T. Individualized image guided iso-NTCP based liver cancer SBRT. Acta Oncol 2006;45: 856–64.
- Mendez RA, Wunderink W, Hussain SM, de Pooter JA, Heijmen BJ, Nowak PC, . Stereotactic body radiation therapy for primary and metastatic liver tumors: A single institution phase I-II study. Acta Oncol 2006;45:831–7.
- Pan CC, Kavanagh BD, Dawson LA, Li XA, Das SK, Miften M, . Radiation-associated liver injury. Int J Radiat Oncol Biol Phys 2010;76(3 Suppl):S94–100.
- Lo SS, Fakiris AJ, Teh BS, Cardenes HR, Henderson MA, Forquer JA, . Stereotactic body radiation therapy for oligometastases. Expert Rev Anticancer Ther 2009;9:621–35.
- Rusthoven KE, Kavanagh BD, Cardenes H, Stieber VW, Burri SH, Feigenberg SJ, . Multi-institutional phase I/II trial of stereotactic body radiation therapy for liver metastases. J Clin Oncol 2009;27:1572–8.
- Tse RV, Hawkins M, Lockwood G, Kim JJ, Cummings B, Knox J, . Phase I study of individualized stereotactic body radiotherapy for hepatocellular carcinoma and intrahepatic cholangiocarcinoma. J Clin Oncol 2008;26:657–64.
- Wong SL, Mangu PB, Choti MA, Crocenzi TS, Dodd GD, III, Dorfman GS, . American Society of Clinical Oncology 2009 clinical evidence review on radiofrequency ablation of hepatic metastases from colorectal cancer. J Clin Oncol 2010;28:493–508.
- McCammon R, Schefter TE, Gaspar LE, Zaemisch R, Gravdahl D, Kavanagh B. Observation of a dose-control relationship for lung and liver tumors after stereotactic body radiation therapy. Int J Radiat Oncol Biol Phys 2009;73: 112–8.
- Lassen-Ramshad Y, Vestergaard A, Muren A, Petersen J. Plan robustness in proton beam therapy of a childhood brain tumour. Acta Oncol 2011 (in press).
- Worm ES, Hansen AT, Petersen JB, Muren LP, Praestegaard LH, Hoyer M. Inter- and intrafractional localisation errors in cone-beam CT guided stereotactic radiation therapy of tumours in the liver and lung. Acta Oncol 2010;49:1177–83.
- Dawson LA, Normolle D, Balter JM, McGinn CJ, Lawrence TS, Ten Haken RK. Analysis of radiation-induced liver disease using the Lyman NTCP model. Int J Radiat Oncol Biol Phys 2002;53:810–21.
- Bentzen SM, Constine LS, Deasy JO, Eisbruch A, Jackson A, Marks LB, . Quantitative Analyses of Normal Tissue Effects in the Clinic (QUANTEC): An introduction to the scientific issues. Int J Radiat Oncol Biol Phys 2010; 76(3 Suppl):S3–9.
- Wang X, Krishnan S, Zhang X, Dong L, Briere T, Crane CH, . Proton radiotherapy for liver tumors: Dosimetric advantages over photon plans. Med Dosim 2008;33:259–67.
- Bush DA, Kayali Z, Grove R, Slater JD. The safety and efficacy of high-dose proton beam radiotherapy for hepatocellular carcinoma: A phase 2 prospective trial. Cancer Epub 2011 Jan 24.
- Kawashima M, Furuse J, Nishio T, Konishi M, Ishii H, Kinoshita T, . Phase II study of radiotherapy employing proton beam for hepatocellular carcinoma. J Clin Oncol 2005;23:1839–46.
- Nakayama H, Sugahara S, Tokita M, Fukuda K, Mizumoto M, Abei M, . Proton beam therapy for hepatocellular carcinoma: The University of Tsukuba experience. Cancer 2009;115:5499–506.
- Sugahara S, Oshiro Y, Nakayama H, Fukuda K, Mizumoto M, Abei M, . Proton beam therapy for large hepatocellular carcinoma. Int J Radiat Oncol Biol Phys 2010;76: 460–6.
- Sugahara S, Nakayama H, Fukuda K, Mizumoto M, Tokita M, Abei M, . Proton-beam therapy for hepatocellular carcinoma associated with portal vein tumor thrombosis. Strahlenther Onkol 2009;185:782–8.