Abstract
Background. Bone-modifying therapy is a primary research interest in breast cancer. Several features contribute to the importance of the bone environment in the management of breast cancer. Firstly, bone metastases represent the most common site of breast cancer metastases and secondly, the emergence of cancer treatment-induced bone loss (CTIBL) among breast cancer survivors and patients is of increasing concern. Furthermore, concordant with the “seed and soil” theory, agents that alter the bone microenvironment may even prevent tumor cell seeding in bone and limit cancer growth. Material and methods. Medical databases and conference proceedings were searched to identify articles, abstracts and clinical trials that have or are investigating denosumab and bisphosphonates in cancer therapy. Our search included a predefined focus on bone-modifying therapies in early and advanced breast cancer. Results and discussion. Bisphosphonates (BPs) have an established role both in the prevention and treatment of CTIBL and have been studied in the adjuvant setting for early breast cancer (EBC). Denosumab is a monoclonal antibody directed against RANK ligand and thereby inhibits osteoclastogenesis and bone resportion. It is the newest agent approved for the treatment of postmenopausal osteoporosis and the prevention of skeletal-related events (SRE) in cancer patients with solid tumors and bone metastases. Denosumab has a favorable toxicity profile in comparison to BPs and has the potential to improve cancer outcomes. Conclusion. This review examines the existing role of denosumab in the treatment of bone complications of breast cancer and its potential role as adjuvant therapy.
Breast cancer is the most common cancer among females on all continents and the most rapidly increasing [Citation1]. Early detection and advances in systemic therapy have improved clinical outcomes [Citation2]. There are approximately 2.6 million survivors in the USA with a prior diagnosis of breast cancer [Citation3]. Women with both early breast cancer (EBC) and metastatic breast cancer (MBC) are surviving longer [Citation4]. Many women in both populations have increased bone fragility either from treatment-induced bone loss or secondary to bony metastases. There already exists substantial data to support a role for bone-conserving therapy in patients with EBC to prevent treatment related bone loss [Citation5–10]. Currently the World Health Organization, the National Osteoporosis Foundation and the American Society of Clinical Oncology (ASCO) recommend pharmacologic intervention based on a women's bone mineral density (BMD) T-score and associated risk factors for osteoporosis.
In advanced breast cancer, bone-modifying agents are important adjuncts to care in patients with metastatic bone disease. Skeletal-related complications of MBC include pathologic fractures, pain, spinal cord compression, and hypercalcemia of malignancy. By preventing SRE, bone-modifying agents can improve patient quality of life and performance status – metrics, which are central to palliation at the end of life.
After BPs were shown to preserve bone density and reduce fracture risk in osteoporosis, they became the first bone-targeting agents in breast cancer. Of the bone-modifying drugs now available, BPs were the first agents demonstrated to prevent chemotherapy-induced bone loss in premenopausal women with breast cancer [Citation8,Citation9]. Three subsequent trials, the Zometa-Femara Adjuvant Synergy Trials (Z-FAST, ZO-FAST, and E-ZO-FAST), established a role for intravenous zoledronate (ZOL) to prevent BMD loss in postmenopausal women on aromatase inhibitor (AI) therapy for breast cancer [Citation6,Citation11,Citation12]. Oral BP therapy was later shown to be efficacious in preventing AI-induced bone loss [Citation13,Citation14]. In the MBC setting, oral and intravenous BPs reduce skeletal complications, palliate bone pain, and improve quality of life [Citation15–17]. New recommendations supporting the use of BPs in MBC were outlined in the ASCO 2011 guidelines [Citation18].
In addition to improving morbidity and mortality outcomes by stabilizing the bone matrix, bone-modifying therapies may have direct antitumor effects. Multiple in vivo studies support the belief that bone dynamics can be modulated to interrupt cancer progression [Citation19–21]. Preclinical studies first reported that BPs could affect cancer outcomes [Citation22–25], a finding that was supported by the Austrian Breast and Colorectal Study Group trial 12 (ABCSG-12) [Citation9]. However, updated results from the Adjuvant Zoledronic Acid to Reduce Recurrence (AZURE) trial do not support the ABCSG-12 findings [Citation26]. At this time, further study is needed to clarify if BPs affect disease-free survival (DFS) or overall survival (OS) in breast cancer.
Denosumab is the newest agent that targets bone metabolism and has been investigated in breast cancer patients. Denosumab is a monoclonal antibody active against the Receptor Activator of Nuclear factor Kappa B ligand (RANKL) and is approved by the United States Food and Drug Administration (FDA) for treating postmenopausal osteoporosis and metastatic bone disease. By interfering with the RANKL pathway, denosumab prevents osteoclast activation and inhibits bone resorption. Denosumab therefore preserves bone density by increasing cortical and trabecular bone mass, volume and strength [Citation27].
Clinical indications and utility of denosumab in cancer patients are evolving. In 2010 denosumab was approved by the FDA for the prevention of SREs in patients with bony metastases after randomized Phase III trials demonstrated non-inferiority of denosumab compared to ZOL and improved tolerability [Citation28–30]. Like BPs both preclinical studies and clinical data suggest that denosumab may have anticancer effects. Herein, we review the mechanisms of bone loss and discuss how denosumab may preserve bone integrity and improve clinical outcomes in patients with breast cancer.
Material and methods
Search strategy and selection criteria
We have conducted a literature search through PubMed using relevant search terms: breast cancer, denosumab, bisphosphonates, skeletal-related events, and cancer treatment-induced bone loss. From the PubMed search we identified articles of interest and reviewed citations within the article to identify additional literature pertaining to review. Conference abstracts were also referenced from the most recent national conference hosted by the ASCO (2011) and from the most recent San Antonio Breast Cancer Symposium (2010) that pertained to our topic of interest. Active/ongoing clinical trials investigating denosumab for breast cancer therapy, its efficacy to prevent and treat CTIBL in early cancer, and its role in reducing SRE for metastatic cancer were identified using clinicaltrials.org and included in this review.
Results and discussion
Mechanisms of bone loss in breast cancer
Patients with EBC often develop bone loss secondary to cancer treatment itself, while in MBC metastases cause bone fragility and associated complications. Three mechanisms of bone loss due to cancer treatment have been identified. The first is as a result of estrogen deprivation therapies. Second, chemotherapies and supportive drugs, such as steroids, affect bone density directly or do so indirectly by the induction of premature ovarian failure. Therapeutic ovarian ablation, whether medically or surgically induced, also results in premature menopause with consequent bone loss.
In postmenopausal women there is on average a 2.6% loss of bone density in the first year of breast cancer treatment when treated with an aromatase inhibitor (AI) [Citation31]. In premenopausal women bone density loss averages 8% in the first year of treatment with premature ovarian suppression [Citation32]. In contrast bone loss during natural menopause is typically 1% per year [Citation31]. To date, no study has correlated bone loss in EBC with adverse clinical outcomes although indirect evidence shows that osteoporotic women with breast cancer have a higher incidence of fractures and mortality compared to age-matched controls [Citation33].
Endocrine therapies may interfere with estrogen signaling (e.g. tamoxifen) or inhibit estrogen production (e.g. AIs); both of which may precipitate bone loss depending on a woman's menopausal status. Tamoxifen was the first antiestrogen therapy used for treating breast cancer and is a mixed estrogen agonist/antagonist [Citation34]. Tamoxifen effects on bone are dependent on the ambient estrogen concentrations; tamoxifen causes bone loss in premenopausal women, but is bone protective in postmenopausal women [Citation35]. AIs, which have a role in treating postmenopausal women with breast cancer, cause bone resorption and a higher fracture risk compared to tamoxifen [Citation36,Citation37]. However, since AIs significantly reduce the risk of breast cancer recurrence in postmenopausal women at five years compared to tamoxifen, and overall have a more favorable side effect profile, AIs are preferred for adjuvant treatment among postmenopausal patients. Within the class, the impact of different AIs on bone density is still being studied. Recent data suggest that the steroidal AI exemestane may result in less BMD loss and potentially reduced fracture risk compared to the non-steroidal AIs, anastrozole and letrozole [Citation38].
Cytotoxic chemotherapy is the only standard adjuvant treatment option for women with hormone-receptor negative breast cancer and is also used in women with high-risk hormone receptor positive disease. Chemotherapy treatment causes bone loss by directly damaging bone architecture or inducing early menopause in premenopausal women, and/or through concomitant steroid use.
In MBC, tumor cells can affect bone by secreting growth factors that stimulate bone resorption [Citation19]. Bone resorption releases factors that subsequently promote tumor growth and propagate a “vicious cycle” of tumor expansion and bone destruction [Citation19]. Bone-modifying agents like BPs and denosumab have the potential to break this cycle and prevent bone loss [Citation39].
Clinical outcomes of bone metastases in breast cancer
Bone is the most common site to which breast cancer metastasizes and is associated with considerable morbidity and mortality [Citation40,Citation41]. Bone metastases in breast cancer are predominately osteolytic and can cause hypercalcemia, increased fracture risk, skeletal instability, skeletal pain and spinal cord compression [Citation42]. These complications can require palliative surgery, palliative radiation and hospitalization, and all correspond to poor quality of life outcomes [Citation43]. Hypercalcemia of malignancy is more common in patients with MBC (30–65%) than in other cancers and generally portends a worse prognosis [Citation44]. After a pathologic fracture, the relative risk of death is increased by 32% in breast cancer compared to patients without breast cancer and similar fractures (p< 0.01) [Citation45]. This risk is notably higher for breast cancer than multiple myeloma or prostate cancer, which have a 20% relative increase in mortality [Citation45]. These differences are unexplained, but suggest that MBC causes more complications than other malignancies when metastatic to bone.
Denosumab: Mechanism of action
Bone is a dynamic system, balancing bone formation and resorption. When this balance is disrupted, clinical disease develops. Within bone, tumor cells secrete growth factors, cytokines, and hormones, such as PTHrP, that stimulate osteoblasts to release RANKL, which in turn activates osteoclasts to resorb bone (). In the absence of cancer, the balance of bone formation and resorption is regulated by osteoprotergerin, a natural decoy that binds to the RANK receptor, but does not activate osteoclasts. Bone resorption releases factors from bone that nurture tumor growth and propel tumor proliferation and bone destruction (). Agents that target RANKL or upregulate osteoprotergerin will theoretically disrupt and halt this process. Denosumab is a human IgG2 antibody designed to bind with a high affinity and specificity to RANKL. Upon binding free RANKL, denosumab inhibits osteoclast function and survival. It therefore has similar downstream effects to osteoprotergerin and preserves bone. Denosumab may also affect inflammation and immunity via RANKL/RANK signaling. RANKL is produced by CD4 + T cells and the RANK receptor is expressed on antigen presenting cells (monocytes, macrophages and dendritic cells) [Citation46]. Thus cell signaling that affects immunity may influence cancer cell migration [Citation47,Citation48] and modulation of the OPG/RANKL/RANK system prevents skeletal metastases in vivo [Citation48]. RANK signaling plays a role in the development of breast tissue [Citation49], angiogenesis and endothelial permeability [Citation50], and inhibition of RANK on these processes may affect breast cancer pathogenesis.
Figure 1. Vicious cycle of osteolytic metastases. Tumor cells secrete cytokines, growth factors, and hormones including parathyroid-hormone related peptide (PTHrP) that stimulate osteoblasts (OB) to secrete RANKL. RANKL activates osteoclasts (OC) to resorb bone, which releases factors: bone morphogenic protein (BMP), fibroblast growth factor (FGF), insulin-like growth factor (IGF), platelet-derived growth factor, and transforming-growth factor-α (TNFα); which promote tumor growth. Both denosumab and bisphosphonates target the bone and interrupt this vicious cycle that supports tumor cells and damages bone. Denosumab binds to RANKL and prevents osteoclast differentiation and activation that therefore halts bone resorption and prevents the release of factors that promote tumor growth. Bisphosphonates inhibit osteoclast activity and induce cellular apoptosis.
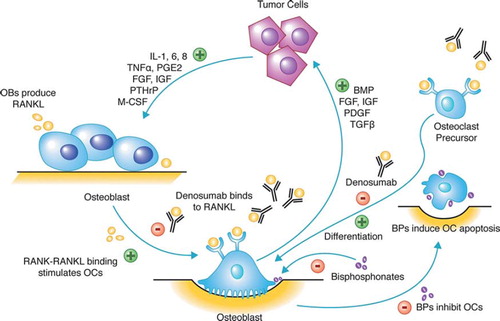
Denosumab versus bisphosphonates
Efficacy for prevention of cancer treatment-induced bone loss. The utility of both oral and intravenous BP administration for the prevention of CTIBL has been investigated in women with breast cancer. Although the evidence for oral therapy in the premenopausal setting has not been uniform [Citation51–53], two trials did show that oral BPs prevented AI-induced bone loss in postmenopausal women [Citation13,Citation14]. In contrast, intravenous BP use has consistently been shown to prevent CTIBL [Citation6,Citation8,Citation11,Citation12,Citation54,Citation55]. Although BPs have been shown to prevent CTIBL, whether or not the reduction in bone loss translates into reduced fracture rates has yet to be definitively demonstrated [Citation56].
To date denosumab has not as yet been compared in randomized control trial with BPs for the prevention of fracture caused by CTIBL. However, the efficacy of denosumab compared to BPs has been evaluated for postmenopausal osteoporosis. In comparison to intravenous BPs, denosumab is equally efficacious [Citation57], and appears superior to oral BP treatment for osteoporosis [Citation58]. A head-to-head trial examining denosumab and oral alendronate therapy showed that denosumab increases BMD better than alendronate [Citation59]. Denosumab also more profoundly suppressed N-telopeptide, a marker of bone destruction and proxy for fracture risk [Citation60], compared to intravenous BP [Citation59]. In concept if denosumab is more effective than BPs in preventing osteoporotic bone loss, one can postulate that denosumab will be superior to BPs in the prevention of CTIBL.
Preliminary results regarding the efficacy of denosumab in the oncology setting have confirmed a role for the prevention of CTIBL [Citation61]. In the Hormone Ablation Therapy Trial for EBC (HALT-BC), 252 women with hormone receptor-positive EBC treated with AI therapy and documented osteopenia were randomized to denosumab every six months or placebo. At 12 and 24 months, denosumab increased BMD in total hip, total body, femoral neck and radius. Other trials confirmed that denosumab enhances suppression of N-telopeptide levels compared to intravenous pamidronate among patients with bony metastases [Citation62]. Increased suppression of N-telopeptide levels corresponds with bone preservation and reduced fracture risk [Citation63–65]. Currently, a second Phase 3 trial is underway to investigate denosumab on CTIBL in patients with EBC on AI therapy [Citation66]. This trial, known as ABCSG-18, is recruiting patients with EBC and will be the first trial to examine if denosumab impacts clinical outcomes (fracture rates) for CTIBL [Citation66]. Of note, denosumab was demonstrated to reduce the number of vertebral fractures in men with early prostate cancer receiving androgen deprivation therapy [Citation67].
In summary, it is clear that early bone-conserving therapy prevents CTIBL, however the best agent, dose and duration of therapy are still uncertain. Considering the current clinical data, biannual administration of ZOL is recommended to prevent CTIBL in both pre- and postmenopausal women with breast cancer and low BMD. However, denosumab will likely have an emerging role in CTIBL since early studies indicate that denosumab is more efficacious that BPs in suppressing bone turnover.
Efficacy in the metastatic setting. BPs have a well-established role in the prevention of SREs secondary to bone metastases in MBC [Citation15]. Intravenous BPs have improved efficacy compared to oral administration and are therefore preferred in oncologic practice. In addition to preventing SREs, BPs palliate bone pain and improve quality of life [Citation16]. ASCO Clinical Practice Guidelines recommend all patients with MBC to be treated with a bone-modifying agent and advise physicians to consider intravenous BP therapy if renal function is preserved [Citation18].
Denosumab also has a role in the metastatic setting and now has an approved indication for the treatment of bony metastases from breast cancer [Citation18]. Denosumab may be preferable to intravenous BPs for all metastatic cancers involving bone [Citation30,Citation68]. Three Phase 3 trials have compared the efficacy of denosumab versus intravenous ZOL for delaying or preventing SREs in metastatic cancer () [Citation28–30]. Stopeck et al. randomized women with MBC to either subcutaneous denosumab 120 mg or intravenous ZOL 4 mg every four weeks [Citation28]. The trial enrolled 2046 women and measured time to first SRE (either pathologic fracture, radiation or surgery to the bone, or spinal cord compression). Denosumab was superior to ZOL in delaying time to first SRE by 18% (HR 0.82, p < 0.01) and reduced risk of first and subsequent SRE by 23% (rate ratio 0.77, p = 0.001). Consistent with prior Phase 2 studies, denosumab resulted in greater suppression of bone turnover markers compared to ZOL. Secondary endpoints including overall survival (OS) and disease progression were similar between groups [Citation28].
Table I. Summary of key clinical trials examining the use of denosumab in cancer therapy.
Denosumab's efficacy has been compared to ZOL in two further Phase 3 trials. Henry et al. randomized patients with metastatic solid tumors (excluding breast and prostate cancer) or multiple myeloma to denosumab or ZOL [Citation29]. Denosumab was as effective as ZOL in delaying time to first and subsequent SRE, but was not superior [Citation29]. In Fizazi et al., denosumab was superior to ZOL in metastatic prostate cancer as measured by time to first SRE [Citation30].
Three additional trials have investigated denosumab in metastatic disease [Citation69–71]. One trial found that denosumab suppressed urinary N-telopeptide levels similarly to intravenous BPs among women with MBC who had not previously received BP therapy [Citation69]. A second study showed that the addition of denosumab to intravenous bisphosphonate therapy normalized urinary N-telopeptide levels more frequently than continuing intravenous BP therapy alone [Citation70]. A final study is underway and will investigate the effect of denosumab on rates of hypercalcemia of malignancy [Citation71]. Preliminary results from the pending trials will soon be available and will help to define the role of densosumab for the prevention and treatment of MBC.
Adverse effects profile. In clinical trials, denosumab has a favorable toxicity profile (). Compared to oral aledronate treatment in Phase 2 trial, denosumab resulted in less dyspepsia (10.5% vs. 26.1% for aledronate) and less osteoarthritis (1.9% vs. 10.9%) [Citation72]. In the early osteoporosis trials, there was a nonsignificant trend toward more community-acquired infections in the denosumab group [Citation74], but this trend was not reproduced in the Phase 3 oncology trials [Citation28–30,Citation73]. The reported incidence of osteonecrosis of the jaw (ONJ) is low (approximately 2%) and is not statistically different to the rate with ZOL therapy in most Phase 3 trials [Citation28–30]. Importantly denosumab has less renal toxicity than BPs. In Stopeck et al. where women with MBC were exclusively studied, the incidence of renal adverse effects was only 5.9% with denosumab compared to 20% with ZOL in patients with baseline renal insufficiency (CrCl < 60 ml/min) [Citation28]. Furthermore, denosumab does not require dose-adjustment for reduced creatinine clearance. Subcutaneous denosumab administrations might therefore be particularly useful for patients with MBC who require treatment with nephrotoxic chemotherapy, such as platinum compounds, or for patients with renal insufficiency.
Antitumor effects of bone-targeting agents. The “seed and soil” hypothesis of cancer metastasis was proposed over a century ago, with the idea that cancer cells might remain dormant yet viable in certain “nurturing” microenvironments for long periods of time. Clinical observation has established that breast tumor cells have a tropism for bone and new research suggests that the natural history of breast cancer may be modulated by targeting bone dynamics [Citation75–77].
Three observational studies looked at whether oral BP use lower breast cancer risk. Chlebowski et al. observed lower rates of breast cancer incidence among postmenopausal women taking oral BPs [Citation78]. The analysis that included 154,768 participants found that women receiving oral BPs for osteoporosis (n = 2816) had a 32% relative risk reduction of invasive breast cancer compared to those who did not receive BPs (HR = 0.68, 95% CI: 0.52–0.88) [Citation78]. Two smaller studies also associated BP use with a lower breast cancer incidence [Citation79,Citation80], and although these studies contain possible confounding factors, the concordance of their findings (a similar risk reduction of ∼30% in the incidence of breast cancer) supports the hypothesis that BPs may have an antitumor effect.
Further evidence for an antitumor effect was suggested by ABCSG-12, which randomized 1803 premenopausal women with endocrine-responsive EBC to tamoxifen or anastrozole without finding a significant efficacy advantage for either arm [Citation8]. A further randomization within the study assigned a subset of patients to receive ZOL. Subjects receiving ZOL not only experienced fewer bone and extra-osseous metastases, but also an improvement in DFS (94% with ZOL versus 90.8% without ZOL, p = 0.01). This absolute increase of 3.2% in DFS represented a 36% risk reduction in disease progression [Citation8,Citation9]. In postmenopausal women, the ZO-FAST trial has similar results to suggest that upfront ZOL reduces the risk of local and distant disease recurrence at 36 months follow-up [Citation11] and was confirmed by the Z-FAST study [Citation6]. While these data are provocative, ascertainment bias may account for these findings because patients were restaged at the advent of a SRE and suppression of pain by the BP may have led to earlier detection of occult disease in organs other than bone. Indeed results presented from the AZURE study contradict the ABCSG-12 findings [Citation26]. A total of 3360 pre- and postmenopausal patients with stage II/III breast cancer were randomized to receive standard adjuvant therapy (chemotherapy and/or endocrine therapy) or standard therapy plus ZOL for five years. At a median follow-up of 59 months, there was no significant difference in DFS between the control patients and the ZOL group (HR = 0.98; p = 0.79 for DFS), OS (HR = 0.85, p = 0.07), or in the type of recurrence (locoregional, distant, contralateral breast, etc) [Citation26]. A predefined analysis of OS by menopausal status demonstrated no benefit of ZOL treatment in patients who were pre- or perimenopausal or of unknown menopausal status, however ZOL therapy did improve OS for patients who were > 5 years postmenopausal (adjusted HR = 0.74; p = 0.04) [Citation26]. The authors hypothesize that adjuvant BP efficacy may be dependent on a low concentration of estrogen within the bone microenvironment. Of note in a subgroup analysis (n = 205), patients receiving both neoadjuvant chemotherapy and ZOL had reduced tumor size (43% relative reduction, 15.5 vs. 27.4 mm, p = 0.006) and higher rates of pathologic complete response compared to chemotherapy alone (6.9% vs. 11.7%, p = 0.146) [Citation81]. These subset analyses need confirming in a prospective study before being interpretable. Currently, ZOL is not approved for adjuvant treatment of EBC.
Mechanisms of putative anticancer activity of BPs remain investigational, however they may relate to their effects on the mevalonate pathway. Nitrogen-containing BPs inhibit farnesyl pyrophosphate synthase (FPPS) in the mevalonate pathway (also known as the HMG-CoA reductase pathway) and prevents the activation of G-proteins [Citation82]. Downstream effects include decreased tumor invasion, proliferation, adhesion and impaired angiogenesis [Citation83]. Through FPPS inhibition, one study demonstrated that zoledronate treatment results in an accumulation of mevalonate metabolites in breast cancer cells in vivo [Citation83]. These metabolites are subsequently secreted by the cancer cells and promote γδ -T-cell chemotaxis to the tumor, which are well known for their anticancer activity [Citation83,Citation84]. At this time it is unclear whether BPs have an antitumor effect alone or if BPs synergize with the effects of standard therapy – at the cellular level, BPs potentiate apoptosis during chemotherapy and modulate growth factors that propel cancer growth [Citation85–87].
No clinical trial has yet investigated if denosumab prolongs DFS or OS in EBC. There is, however, convincing evidence that denosumab impacts the course prostate cancer [Citation73] and experimental data suggests that denosumab will have anticancer effects against breast tumor cells. The RANK-RANKL pathway is strictly regulated during mammary gland development and overexpression results in increased proliferation and decreased apoptosis, both of which are downstream effects commonly implicated in breast tumorigenesis [Citation88]. In fact, RANKL activity may explain in the higher incidence of breast cancer noted after pregnancy [Citation89,Citation90]. To further support this belief, RANKL inhibition in vivo was shown to reduce hormone-induced proliferation of mammary epithelium and attenuate tumorigenesis [Citation91]. Inactivation of the RANKL receptor also decreases the incidence and delays the onset of progestin-driven breast cancer [Citation92]. Activation of RANKL stimulates metalloproteinases to breakdown bone matrix and release vascular endothelial growth factors that promote angiogenesis and nurture cancer growth [Citation93]. Furthermore in animal models of MBC, RANKL inhibition reduces the burden of tumor in bone [Citation94].
Future directions
There are three potential roles for denosumab in breast cancer. The first is to prevent or ameliorate CTIBL and data currently suggest that denosumab is superior to bisphosphonate therapy in this regard. As yet no trial has compared denosumab and bisphosphonates head-to-head for prevention of CTIBL and we await future investigations to determine if one agent has superior efficacy in preventing bone loss in breast cancer.
Denosumab has an established role in MBC involving bone. ASCO clinical practice guidelines recommend either denosumab or BP therapy and state there is insufficient evidence to demonstrate that one bone-modifying agent has greater efficacy [Citation18]. However recently the FDA has approved densoumab for MBC and recognized clinical trial results that showed superior efficacy compared to BPs. Denosumab is the preferred choice in patients with impaired renal function as it does not require creatinine monitoring. In the metastatic setting, the optimal dosing intervals for both bisphosphonates and denosumab need to be characterized since less frequent dosing will improve the cost-effectiveness of treatment.
Most important is the possibility that denosumab can reduce rates of distant metastases by manipulation of the bone microenvironment or potentially by a direct antitumor effect. To address this question, a Phase 3 study has recently opened and will examine denosumab as adjuvant treatment for women with EBC at high risk of recurrence (D-CARE) [Citation95] (). In this trial 4500 women with high-risk EBC (Stage II and III) will be randomized to denosumab or placebo for five years. The primary endpoint compares bone metastasis-free survival between denosumab and placebo. Secondary endpoints include DFS, OS and distance recurrence-free survival.
Further well-designed clinical trials are needed to fully evaluate the potential roles of denosumab in breast cancer therapy. As outlined by Hudis et al., standard definitions for events and endpoints need to be carefully defined with consideration given to the use of surrogate endpoints in place of overall survival to contain costs and length of clinical trials [Citation96]. Disease-free survival will continue to be a relevant endpoint and as more therapies emerge which target a specific microenvironment, lack of disease in a given tissue may be a valuable surrogate. In the case of denosumab, an impact on bone-metastasis-free survival may extend quality of life and even be an acceptable proxy for overall survival. Given the possible benefit of denosumab as a breast cancer prevention therapy, it will be interesting to follow outcomes of women with ductal carcinoma in situ and the incidence of contra-lateral breast cancers in adjuvant trials.
Conclusions
There is a need for bone-targeting agents in breast cancer to prevent and treat therapy-induced bone loss, to lower the incidence of skeletal-related events among patients with established bony metastases and, potentially, to change the natural history of the disease by modulating the bone microenvironment. Denosumab is the only licensed drug that targets RANKL and is approved for the treatment of postmenopausal osteoporosis and more recently for the prevention of skeletal-related events in patients with metastatic solid tumors involving bone. The drug is superior to zoledronate for the prevention of skeletal complications in metastatic disease, and offers a more attractive adverse effect profile and easier route of administration to bisphosphonates. Preclinical data suggests that RANKL inhibition with denosumab may alter the pathogenesis of breast cancer, and therefore it is being investigated as an adjuvant agent for the prevention of breast cancer relapse and may in time be studied as a breast cancer chemopreventive agent. At this time, we await further clinical results to help define the role of denosumab therapy in the prevention and treatment of breast cancer.
Notice of Correction
The Early Online version of this article published online ahead of print on 12 Dec 2012 contained an error on page 5. The reference “64” should have read “66” in Table 1.
This has been corrected for the current version.
Acknowledgements
We would like to thank Mauro Martino for his assistance with preparation. Paul Goss is supported by the Avon Foundation, New York. Paul Goss is the Chair of the D-Care clinical trial. None of the authors have any conflicts of interest to disclose.
Declaration of interest: The authors report no conflicts of interest. The authors alone are responsible for the content and writing of the paper.
References
- American Cancer Society. Global cancer facts and figures, 2nd ed. Atlanta: American Cancer Society; 2011.
- U.S. Cancer Statistics Working Group. United States Cancer Statistics: 1999–2006 incidence and mortality web-based report. Atlanta: Department of Health and Human Services, Centers for Disease Control and Prevention, and National Cancer Institute; 2010.
- Howlader N, Noone AM, Krapcho M, Neyman N, Aminou R, Waldron W, . SEER Cancer Statistics Review, 1975–2008, National Cancer Institute. Bethesda, MD, 2011. [cited 2011 Nov 21]. Available from: http://seer.cancer.gov/statfacts/html/breast.html.
- Desantis C, Siegel R, Bandi P, Jemal A. Breast cancer statistics, 2011. CA Cancer J Clin Epub 2011 Oct 3.
- Brufsky AM. Cancer treatment-induced bone loss: Pathophysiology and clinical perspectives. Oncologist 2008;13: 187–95.
- Brufsky AM, Bosserman LD, Caradonna RR, Haley BB, Jones CM, Moore HC, . Zoledronic acid effectively prevents aromatase inhibitor-associated bone loss in postmenopausal women with early breast cancer receiving adjuvant letrozole: Z-FAST study 36-month follow-up results. Clin Breast Cancer 2009;9:77–85.
- Carlson RW, Allred DC, Anderson BO, Burstein HJ, Carter WB, Edge SB, . Breast cancer. Clinical practice guidelines in oncology. J Natl Compr Canc Netw 2009;7:122–92.
- Gnant M, Mlineritsch B, Luschin-Ebengreuth G, Kainberger F, Kassmann H, Piswanger-Solkner JC, . Adjuvant endocrine therapy plus zoledronic acid in premenopausal women with early-stage breast cancer: 5-year follow-up of the ABCSG-12 bone-mineral density substudy. Lancet Oncol 2008;9:840–9.
- Gnant M, Mlineritsch B, Schippinger W, Luschin-Ebengreuth G, Postlberger S, Menzel C, . Endocrine therapy plus zoledronic acid in premenopausal breast cancer. N Engl J Med 2009;360:679–91.
- Higgins MJ, Park BH. Expanding role of bisphosphonates in the management of early breast cancer. Expert Rev Anticancer Ther 2009;9:1051–4.
- Eidtmann H, de Boer R, Bundred N, Llombart-Cussac A, Davidson N, Neven P, . Efficacy of zoledronic acid in postmenopausal women with early breast cancer receiving adjuvant letrozole: 36-month results of the ZO-FAST Study. Ann Oncol 2010;21:2188–94.
- Llombarto A, Frassoldati A, Paija O, Sleeboom HP, Jerusalem G, Mebis J, . Effect of zoledronic acid on aromatase inhibitor-associated bone loss in postmenopausal women with early breast cancer receiving adjuvant letrozole: E-ZO-FAST 36-month follow up. Presented at the ASCO 2009 Breast Cancer Symposium. San Francisco, CA, October 8–10, 2009.
- Van Poznak C, Hannon RA, Mackey JR, Campone M, Apffelstaedt JP, Clack G, . Prevention of aromatase inhibitor-induced bone loss using risedronate: The SABRE trial. J Clin Oncol 2010;28:967–75.
- Lester JE, Dodwell D, Purohit OP, Gutcher SA, Ellis SP, Thorpe R, . Prevention of anastrozole-induced bone loss with monthly oral ibandronate during adjuvant aromatase inhibitor therapy for breast cancer. Clin Cancer Res 2008;14: 6336–42.
- Pavlakis N, Schmidt R, Stockler M. Bisphosphonates for breast cancer. Cochrane Database Syst Rev 2005; CD003474.
- Costa L, Major PP. Effect of bisphosphonates on pain and quality of life in patients with bone metastases. Nat Clin Pract Oncol 2009;6:163–74.
- Body JJ, Diel IJ, Lichinitzer M, Lazarev A, Pecherstorfer M, Bell R, . Oral ibandronate reduces the risk of skeletal complications in breast cancer patients with metastatic bone disease: Results from two randomised, placebo-controlled phase III studies. Br J Cancer 2004;90:1133–7.
- Van Poznak CH, Temin S, Yee GC, Janjan NA, Barlow WE, Biermann JS, . American Society of Clinical Oncology executive summary of the clinical practice guideline update on the role of bone-modifying agents in metastatic breast cancer. J Clin Oncol 2011;29:1221–7.
- Roodman GD. Mechanisms of bone metastasis. N Engl J Med 2004;350:1655–64.
- Oyajobi BO, Mundy GR. Receptor activator of NF-kappaB ligand, macrophage inflammatory protein-1alpha, and the proteasome: Novel therapeutic targets in myeloma. Cancer 2003;97:813–7.
- Morony S, Warmington K, Adamu S, Asuncion F, Geng Z, Grisanti M, . The inhibition of RANKL causes greater suppression of bone resorption and hypercalcemia compared with bisphosphonates in two models of humoral hypercalcemia of malignancy. Endocrinology 2005; 146:3235–43.
- Aviles A, Nambo MJ, Neri N, Castaneda C, Cleto S, Huerta-Guzman J. Antitumor effect of zoledronic acid in previously untreated patients with multiple myeloma. Med Oncol 2007;24:227–30.
- Hirsh V, Major PP, Lipton A, Cook RJ, Langer CJ, Smith MR, . Zoledronic acid and survival in patients with metastatic bone disease from lung cancer and elevated markers of osteoclast activity. J Thorac Oncol 2008;3:228–36.
- Mystakidou K, Katsouda E, Parpa E, Kelekis A, Galanos A, Vlahos L. Randomized, open label, prospective study on the effect of zoledronic acid on the prevention of bone metastases in patients with recurrent solid tumors that did not present with bone metastases at baseline. Med Oncol 2005;22:195–201.
- Zaghloul MS, Boutrus R, El-Hossieny H, Kader YA, El-Attar I, Nazmy M. A prospective, randomized, placebo-controlled trial of zoledronic acid in bony metastatic bladder cancer. Int J Clin Oncol 2010;15:382–9.
- Coleman RE, Marshall H, Cameron D, Dodwell D, Burkinshaw R, Keane M, . Breast-cancer adjuvant therapy with zoledronic acid. N Engl J Med 2011;365:1396–1405.
- Kostenuik PJ, Nguyen HQ, McCabe J, Warmington KS, Kurahara C, Sun N, . Denosumab, a fully human monoclonal antibody to RANKL, inhibits bone resorption and increases BMD in knock-in mice that express chimeric (murine/human) RANKL. J Bone Miner Res 2009;24:182–95.
- Stopeck AT, Lipton A, Body JJ, Steger GG, Tonkin K, de Boer RH, . Denosumab compared with zoledronic acid for the treatment of bone metastases in patients with advanced breast cancer: A randomized, double-blind study. J Clin Oncol 2010;28:5132–9.
- Henry DH, Costa L, Goldwasser F, Hirsh V, Hungria V, Prausova J, . Randomized, double-blind study of denosumab versus zoledronic acid in the treatment of bone metastases in patients with advanced cancer (excluding breast and prostate cancer) or multiple myeloma. J Clin Oncol 2011;29:1125–32.
- Fizazi K, Carducci M, Smith M, Damiao R, Brown J, Karsh L, . Denosumab versus zoledronic acid for treatment of bone metastases in men with castration-resistant prostate cancer: A randomised, double-blind study. Lancet 2011;377:813–22.
- Hadji P. Aromatase inhibitor-associated bone loss in breast cancer patients is distinct from postmenopausal osteoporosis. Crit Rev Oncol Hematol 2009;69:73–82.
- Shapiro CL, Manola J, Leboff M. Ovarian failure after adjuvant chemotherapy is associated with rapid bone loss in women with early-stage breast cancer. J Clin Oncol 2001;19: 3306–11.
- Hadji P, Body JJ, Aapro MS, Brufsky A, Coleman RE, Guise T, . Practical guidance for the management of aromatase inhibitor-associated bone loss. Ann Oncol 2008; 19:1407–16.
- Colleoni M, O'Neill A, Goldhirsch A, Gelber RD, Bonetti M, Thurlimann B, . Identifying breast cancer patients at high risk for bone metastases. J Clin Oncol 2000;18:3925–35.
- Lonning PE. Endocrine therapy and bone loss in breast cancer: Time to close in the RANK(L)? J Clin Oncol 2008; 26:4859–61.
- Perez EA, Josse RG, Pritchard KI, Ingle JN, Martino S, Findlay BP, . Effect of letrozole versus placebo on bone mineral density in women with primary breast cancer completing 5 or more years of adjuvant tamoxifen: A companion study to NCIC CTG MA.17. J Clin Oncol 2006;24:3629–35.
- Lonning PE, Geisler J, Krag LE, Erikstein B, Bremnes Y, Hagen AI, . Effects of exemestane administered for 2 years versus placebo on bone mineral density, bone biomarkers, and plasma lipids in patients with surgically resected early breast cancer. J Clin Oncol 2005;23:5126–37.
- Hershman DL, Cheung AM, Chapman JW, Ingel JN, Ahmed F, Hu H, . Effects of adjuvant exemestane versus anastrozole on bone mineral density: Two-year results of the NCIC CTG MA.27 bone companion study. Presented at the 2011 ASCO Annual Meeting. Chicago, IL, June 3–7, 2011.
- Fornier MN. Denosumab: Second chapter in controlling bone metastases or a new book? J Clin Oncol 2010;28:5127–31.
- James JJ, Evans AJ, Pinder SE, Gutteridge E, Cheung KL, Chan S, . Bone metastases from breast carcinoma: Histopathological – radiological correlations and prognostic features. Br J Cancer 2003;89:660–5.
- Solomayer EF, Diel IJ, Meyberg GC, Gollan C, Bastert G. Metastatic breast cancer: Clinical course, prognosis and therapy related to the first site of metastasis. Breast Cancer Res Treat 2000;59:271–8.
- Coleman RE, Smith P, Rubens RD. Clinical course and prognostic factors following bone recurrence from breast cancer. Br J Cancer 1998;77:336–40.
- Weinfurt KP, Castel LD, Li Y, Timbie JW, Glendenning GA, Schulman KA. Health-related quality of life among patients with breast cancer receiving zoledronic acid or pamidronate disodium for metastatic bone lesions. Med Care 2004;42: 164–75.
- Brufsky AM. The evolving role of bone-conserving therapy in patients with breast cancer. Semin Oncol 2010;37(Suppl 1): S12–9.
- Saad F, Lipton A, Cook R, Chen YM, Smith M, Coleman R. Pathologic fractures correlate with reduced survival in patients with malignant bone disease. Cancer 2007;110:1860–7.
- Ferrari-Lacraz S, Ferrari S. Do RANKL inhibitors (denosumab) affect inflammation and immunity? Osteoporos Int 2011;22:435–46.
- Fouque-Aubert A, Chapurlat R. Influence of RANKL inhibition on immune system in the treatment of bone diseases. Joint Bone Spine 2008;75:5–10.
- Canon JR, Roudier M, Bryant R, Morony S, Stolina M, Kostenuik PJ, . Inhibition of RANKL blocks skeletal tumor progression and improves survival in a mouse model of breast cancer bone metastasis. Clin Exp Metastasis 2008; 25:119–29.
- Fernandez-Valdivia R, Mukherjee A, Ying Y, Li J, Paquet M, DeMayo FJ, . The RANKL signaling axis is sufficient to elicit ductal side-branching and alveologenesis in the mammary gland of the virgin mouse. Dev Biol 2009;328:127–39.
- Min JK, Cho YL, Choi JH, Kim Y, Kim JH, Yu YS, . Receptor activator of nuclear factor (NF)-kappaB ligand (RANKL) increases vascular permeability: Impaired permeability and angiogenesis in eNOS-deficient mice. Blood 2007;109:1495–502.
- Delmas PD, Balena R, Confravreux E, Hardouin C, Hardy P, Bremond A. Bisphosphonate risedronate prevents bone loss in women with artificial menopause due to chemotherapy of breast cancer: A double-blind, placebo-controlled study. J Clin Oncol 1997;15:955–62.
- Hines SL, Mincey BA, Sloan JA, Thomas SP, Chottiner E, Loprinzi CL, . Phase III randomized, placebo-controlled, double-blind trial of risedronate for the prevention of bone loss in premenopausal women undergoing chemotherapy for primary breast cancer. J Clin Oncol 2009;27:1047–53.
- Saarto T, Vehmanen L, Blomqvist C, Elomaa I. Ten-year follow-up of 3 years of oral adjuvant clodronate therapy shows significant prevention of osteoporosis in early-stage breast cancer. J Clin Oncol 2008;26:4289–95.
- Hershman DL, McMahon DJ, Crew KD, Cremers S, Irani D, Cucchiara G, . Zoledronic acid prevents bone loss in premenopausal women undergoing adjuvant chemotherapy for early-stage breast cancer. J Clin Oncol 2008;26:4739–45.
- Fuleihan Gel H, Salamoun M, Mourad YA, Chehal A, Salem Z, Mahfoud Z, . Pamidronate in the prevention of chemotherapy-induced bone loss in premenopausal women with breast cancer: A randomized controlled trial. J Clin Endocrinol Metab 2005;90:3209–14.
- Valachis A, Polyzos NP, Georgoulias V, Mavroudis D, Mauri D. Lack of evidence for fracture prevention in early breast cancer bisphosphonate trials: A meta-analysis. Gynecol Oncol 2010;117:139–45.
- Khosla S. Increasing options for the treatment of osteoporosis. N Engl J Med 2009;361:818–20.
- Burkiewicz JS, Scarpace SL, Bruce SP. Denosumab in osteoporosis and oncology. Ann Pharmacother 2009;43:1445–55.
- Brown JP, Prince RL, Deal C, Recker RR, Kiel DP, de Gregorio LH, . Comparison of the effect of denosumab and alendronate on bone mineral density and biochemical markers of bone turnover in postmenopausal women with low bone mass: A randomized, blinded, phase 3 trial. J Bone Miner Res 2009;24:153–161.
- Rogers A, Hannon RA, Eastell R. Biochemical markers as predictors of rates of bone loss after menopause. J Bone Miner Res 2000;15:1398–404.
- Ellis GK, Bone HG, Chlebowski R, Paul D, Spadafora S, Smith J, . Randomized trial of denosumab in patients receiving adjuvant aromatase inhibitors for nonmetastatic breast cancer. J Clin Oncol 2008;26:4875–82.
- Body JJ, Facon T, Coleman RE, Lipton A, Geurs F, Fan M, . A study of the biological receptor activator of nuclear factor-kappaB ligand inhibitor, denosumab, in patients with multiple myeloma or bone metastases from breast cancer. Clin Cancer Res 2006;12:1221–8.
- Bergmann P, Body JJ, Boonen S, Boutsen Y, Devogelaer JP, Goemaere S, . Evidence-based guidelines for the use of biochemical markers of bone turnover in the selection and monitoring of bisphosphonate treatment in osteoporosis: A consensus document of the Belgian Bone Club. Int J Clin Pract 2009;63:19–26.
- Reginster JY, Henrotin Y, Christiansen C, Gamwell-Henriksen E, Bruyere, Collette J, . Bone resorption in post-menopausal women with normal and low BMD assessed with biochemical markers specific for telopeptide derived degradation products of collagen type I. Calcif Tissue Int 2001;69:130–7.
- Melton LJ, 3rd, Khosla S, Atkinson EJ, O'Fallon WM, Riggs BL. Relationship of bone turnover to bone density and fractures. J Bone Miner Res 1997;12:1083–91.
- Amgen. Study to determine treatment effects of denosumab in patients with breast cancer receiving aromatase inhibitor therapy. Identifier: NCT00556374. [cited on 2011 Jun 28]. Available from: http://www.clinicaltrials.gov.
- Smith MR, Saad F, Egerdie B, Szwedowski M, Tammela TL, Ke C, . Effects of denosumab on bone mineral density in men receiving androgen deprivation therapy for prostate cancer. J Urol 2009;182:2670–5.
- Henry DH, Costa L, Goldwasser F, Hirsh V, Hungria V, Prausova J, . Randomized, double-blind study of denosumab versus zoledronic acid in the treatment of bone metastases in patients with advanced cancer (excluding breast and prostate cancer) or multiple myeloma. J Clin Oncol 2011;29:1125–32.
- Lipton A, Steger GG, Figueroa J, Alvarado C, Solal-Celigny P, Body JJ, . Extended efficacy and safety of denosumab in breast cancer patients with bone metastases not receiving prior bisphosphonate therapy. Clin Cancer Res 2008;14:6690–6.
- Fizazi K, Lipton A, Mariette X, Body JJ, Rahim Y, Gralow JR, . Randomized phase II trial of denosumab in patients with bone metastases from prostate cancer, breast cancer, or other neoplasms after intravenous bisphosphonates. J Clin Oncol. 2009;27:1564–71.
- Amgen. Study of denosu mab in the treatment of hypercalcemia of malignancy in subjects with elevated serum calcium. Identifier: NCT00896454. [cited on 2011 Jun 28]. Available from: http://www.clinicaltrials.gov.
- Lewiecki EM, Miller PD, McClung MR, Cohen SB, Bolognese MA, Liu Y, . Two-year treatment with denosumab (AMG 162) in a randomized phase 2 study of postmenopausal women with low BMD. J Bone Miner Res 2007;22:1832–41.
- Smith MR, Saad F, Coleman R, Shore N, Fizazi K, Tombal B, . Denosumab and bone-metastases-free survival in men with castration-resistant prostate cancer: results of a phase 3, randomized, placebo-controlled trial. Lancet 2011 Nov 15 Epub ahead of print.
- Miller PD, Bolognese MA, Lewiecki EM, McClung MR, Ding B, Austin M, . Effect of denosumab on bone density and turnover in postmenopausal women with low bone mass after long-term continued, discontinued, and restarting of therapy: A randomized blinded phase 2 clinical trial. Bone 2008;43:222–9.
- Diel IJ, Jaschke A, Solomayer EF, Gollan C, Bastert G, Sohn C, . Adjuvant oral clodronate improves the overall survival of primary breast cancer patients with micrometastases to the bone marrow: A long-term follow-up. Ann Oncol 2008; 19:2007–11.
- Ha TC, Li H. Meta-analysis of clodronate and breast cancer survival. Br J Cancer 2007;96:1796–801.
- Powles T, Paterson A, McCloskey E, Schein P, Scheffler B, Tidy A, . Reduction in bone relapse and improved survival with oral clodronate for adjuvant treatment of operable breast cancer [ISRCTN83688026]. Breast Cancer Res 2006;8:R13.
- Chlebowski RT, Chen Z, Cauley JA, Anderson G, Rodabough RJ, McTiernan A, . Oral bisphosphonate use and breast cancer incidence in postmenopausal women. J Clin Oncol 2010;28:3582–90.
- Newcomb PA, Trentham-Dietz A, Hampton JM. Bisphosphonates for osteoporosis treatment are associated with reduced breast cancer risk. Br J Cancer 2010;102:799–802.
- Rennert G, Pinchev M, Rennert HS. Use of bisphosphonates and risk of postmenopausal breast cancer. J Clin Oncol 2010; 28:3577–81.
- Coleman RE, Winter MC, Cameron D, Bell R, Dodwell D, Keane MM, . The effects of adding zoledronic acid to neoadjuvant chemotherapy on tumour response: Exploratory evidence for direct anti-tumour activity in breast cancer. Br J Cancer 2010;102:1099–105.
- Clezardin P. Bisphosphonates’ antitumor activity: An unravelled side of a multifaceted drug class. Bone 2011;48:71–9.
- Benzaid I, Monkkonen H, Stresing V, Bonnelye E, Green J, Monkkonen J, . High phosphoantigen levels in bisphosphonate-treated human breast tumors promote Vgamma9Vdelta2 T-cell chemotaxis and cytotoxicity in vivo. Cancer Res 2011;71:4562–72.
- Santini D, Martini F, Fratto ME, Galluzzo S, Vincenzi B, Agrati C, . In vivo effects of zoledronic acid on peripheral gammadelta T lymphocytes in early breast cancer patients. Cancer Immunol Immunother 2009;58:31–8.
- Yuasa T, Kimura S, Ashihara E, Habuchi T, Maekawa T. Zoledronic acid – a multiplicity of anti-cancer action. Curr Med Chem 2007;14:2126–35.
- Kohno N. Treatment of breast cancer with bone metastasis: Bisphosphonate treatment – current and future. Int J Clin Oncol 2008;13:18–23.
- Ottewell PD, Lefley DV, Cross SS, Evans CA, Coleman RE, Holen I. Sustained inhibition of tumor growth and prolonged survival following sequential administration of doxorubicin and zoledronic acid in a breast cancer model. Int J Cancer 2010;126:522–32.
- Gonzalez-Suarez E, Branstetter D, Armstrong A, Dinh H, Blumberg H, Dougall WC. RANK overexpression in transgenic mice with mouse mammary tumor virus promoter-controlled RANK increases proliferation and impairs alveolar differentiation in the mammary epithelia and disrupts lumen formation in cultured epithelial acini. Mol Cell Biol 2007; 27:1442–54.
- Lambe M, Hsieh C, Trichopoulos D, Ekbom A, Pavia M, Adami HO. Transient increase in the risk of breast cancer after giving birth. N Engl J Med 1994;331:5–9.
- Asselin-Labat ML, Vaillant F, Sheridan JM, Pal B, Wu D, Simpson ER, . Control of mammary stem cell function by steroid hormone signalling. Nature 2010;465:798–802.
- Gonzalez-Suarez E, Jacob AP, Jones J, Miller R, Roudier-Meyer MP, Erwert R, . RANK ligand mediates progestin-induced mammary epithelial proliferation and carcinogenesis. Nature 2010;468:103–7.
- Schramek D, Leibbrandt A, Sigl V, Kenner L, Pospisilik JA, Lee HJ, . Osteoclast differentiation factor RANKL controls development of progestin-driven mammary cancer. Nature 2010;468:98–102.
- Rucci N, Millimaggi D, Mari M, Del Fattore A, Bologna M, Teti A, . Receptor activator of NF-kappaB ligand enhances breast cancer-induced osteolytic lesions through upregulation of extracellular matrix metalloproteinase inducer/CD147. Cancer Res 2010;70:6150–60.
- Morony S, Capparelli C, Sarosi I, Lacey DL, Dunstan CR, Kostenuik PJ. Osteoprotegerin inhibits osteolysis and decreases skeletal tumor burden in syngeneic and nude mouse models of experimental bone metastasis. Cancer Res 2001;61:4432–6.
- Amgen. Study of denosumab as adjuvant treatment for women with high risk early breast cancer receiving neoadjuvant or adjuvant therapy (D-CARE). Identifier: NCT01077154. [cited on 2011 Jun 28]. Available from: http://www.clinicaltrials.gov.
- Hudis CA, Barlow WE, Costantino JP, Gray RJ, Pritchard KI, Chapman JA, . Proposal for standardized definitions for efficacy end points in adjuvant breast cancer trials: The STEEP system. J Clin Oncol 2007;25:2127–32.