Abstract
Background and purpose. The purpose of this study was to evaluate the performance of gantry angle optimisation (GAO) compared to equidistant beam geometry for two inverse treatment planning systems (TPSs) by utilising the information obtained from a range of treatment plans. Material and methods. The comparison was based on treatment plans generated for four different head and neck (H&N) cancer cases using two inverse treatment planning systems (TPSs); Varian Eclipse™ representing dynamic MLC intensity modulated radiotherapy (IMRT) and Oncentra® Masterplan representing segmented MLC-based IMRT. The patient cases were selected on the criterion of representing different degrees of overlap between the planning target volume (PTV) and the investigated organ at risk, the ipsilateral parotid gland. For each case, a number of ‘Pareto optimal’ plans were generated in order to investigate the trade-off between the under-dosage to the PTV (VPTV,D < 95%) or the decrease in dose homogeneity (D5-D95) to the PTV as a function of the mean absorbed dose to the ipsilateral parotid gland (<D>parotid gland). Results. For the Eclipse system, GAO had a clear advantage for the cases with smallest overlap (Cases 1 and 2). The set of data points, representing the underlying trade-offs, generated with and without using GAO were, however, not as clearly separated for the cases with larger overlap (Cases 3 and 4). With the OMP system, the difference was less pronounced for all cases. The Eclipse GAO displays the most favourable trade-off for all H&N cases. Conclusions. We have found differences in the effectiveness of GAO as compared to equidistant beam geometry, in terms of handling conflicting trade-offs for two commercial inverse TPSs. A comparison, based on a range of treatment plans, as developed in this study, is likely to improve the understanding of conflicting trade-offs and might apply to other thorough comparison techniques.
The aim of gantry angle optimisation (GAO) in inverse treatment planning systems (TPSs) is to generate the most advantageous set of gantry angles to produce a three-dimensional (3D) dose distribution as close as possible to the prescribed dose [Citation1]. It has previously been concluded that in-house developed GAO algorithms provide better sparing of organs at risk (OARs) compared to equidistant beam geometry for different treatment sites [Citation1,Citation2].
The comparison of different TPSs and/or treatment modalities is not a trivial task. The ultimate goal is an unbiased approach that considers the entire system, including beam delivery limitations. Accomplishing such an unbiased comparison has proven to be difficult [Citation3]. Previous studies often compare Dose Volume Histograms (DVHs) [Citation1,Citation2] for single generated treatment plans. Using one single plan may compromise the scope of the comparison since it does not elucidate the whole range of plans in an optimisation situation given by multiple conflicting objectives [Citation4,Citation5]. Hence, it is not a complete representation of the TPS. To make the comparison more powerful, information from multiple plans is required. Several treatment plans could be generated to represent one TPS and one delivery technique and eventually result in a set of plans, which characterise that particular system [Citation5–7]. It has been suggested that the so-called Pareto front evaluation may be useful towards such thorough comparisons [Citation8]. A solution is Pareto optimal if it is feasible and if one objective can only be improved by degrading another objective. If several Pareto optimal solutions exist, then there is a trade-off between the objectives [Citation9]. In practice, however, when comparing commercial TPSs, there are many unknown factors out of control of the user, and it is rarely known whether an actual optimised treatment plan represents a Pareto- optimal solution [Citation6]. The possibility of using Pareto front evaluation as a comparative tool between TPSs has recently been supported by several studies [Citation4,Citation6,Citation10]. In these studies, Pareto front evaluation was applied to a range of treatment sites [prostate, head and neck (H&N) and lung cancer], enabling comparisons between different dose calculation algorithms, beam energies [Citation4,Citation6], number of beams [Citation10], gantry angle geometries [Citation6] and also between different delivery techniques [Citation10]. In the studies by Ottoson et al. [Citation4,Citation6] the comparisons were based on convex problems and not GAO, which is likely an example of global non-convex optimisation [Citation11]. Strictly speaking, purely Pareto-optimal solutions are therefore not obtained using GAO. However in this study the features of the Pareto concept as a comparative tool were utilised rather than making in-depth analyses of the non-convex nature of GAO. The notation Pareto front evaluation will hereafter refer to the former with the non-convexity being regarded as beyond the scope of the rather simple and practical implementation used in this study.
In this study, the performance of GAO for two commercial inverse TPSs, Varian Eclipse™ and Oncentra® Masterplan were compared. More specifically, the comparison was based on the information from a range of treatment plans and focused on the effectiveness of GAO in handling two conflicting trade-offs. The performance of each TPS was evaluated comparing the GAO with equidistant beam geometry, resulting in four systems (Eclipse, Eclipse GAO, OMP and OMP GAO) studied. The aim was to compare the entire TPSs and each TPS was therefore assumed to represent a system including the complete chain from the optimisation to the final absorbed dose calculation. Therefore, we base our comparisons on a set of deliverable plans, produced by the system's built-in optimiser, without the requirement that they are strictly Pareto-optimal. Neither do we in this study discuss the accuracy of the delivery of the various plans.
Material and methods
Patient data and treatment planning considerations
In this study, the comparison was based on four H&N cancer cases () previously treated with IMRT for H&N cancer. This treatment site was chosen due to the plan complexity and the challenging anatomy [Citation12], where GAO is anticipated to be valuable. The cases were selected to represent patient groups with different anatomic architecture in terms of different overlap extent between the planning target volume (PTV) and the ipsilateral parotid gland. The fraction of the parotid gland that was overlapping the PTV was 0.20 (0.8%), 0.40 (1.6%), 0.80 (8.6%) and 6.4 (29%) cm3, for Cases 1, 2, 3 and 4, respectively (). A large overlap makes the OAR sparing more challenging. For Cases 1, 2 and 3 the main treatment planning concern was the trade-off between the mutually conflicting objectives for the right parotid gland and PTV of the primary tumour. For Case 4 the main trade-off was associated with the objectives for the left parotid gland and the PTV including the lymph nodes. A mean parotid dose of 26 Gy is commonly proposed as planning goal when aiming for substantial sparing of the parotid gland function [Citation13]. In agreement with these suggestions and the restrictions within the dose protocol applied [Citation14], the maximum permitted mean absorbed dose to the contra lateral parotid gland was 26 Gy to reduce the risk of developing xerostomia. The under-dosage to the PTV was defined as the relative volume receiving less than 95% of the prescribed dose (VPTV,D < 95%) [Citation14] according to the International Commission on Radiation Units and measurements (ICRU) criterion [Citation15]. The trade-off between VPTV,D < 95% and the mean absorbed dose to the overlapping ipsilateral parotid gland (<D>parotid gland) was studied. In addition, we investigated the trade-off between the <D>parotid gland and the dose homogeneity within the PTV by introducing a simple measure for the latter as the difference between the dose to 5% of the PTV volume (D5) and the dose to 95% of the PTV volume (D95) [Citation7], denoted as (D5-D95).
Table I. Dose planning specific parameters: prescribed dose to the PTV (Gy), PTV volume (cm3), parotid gland volume (cm3), overlap between the parotid gland volume and the PTV (cm3, %).
Approximately five to nine beams have shown to be sufficient to achieve acceptable dose homogeneity within the target volumes along with substantial parotid sparing. A further increase in the number of beams yields no apparent improvements in the dose distribution [Citation12,Citation16]. For this reason the beam arrangement in this study consisted of seven coplanar beams spread over 360°, either with a constant angular interval or with angles generated by the GAO in each TPS. The prescribed dose to the PTV for the primary tumour was either 66 Gy (Cases 2 and 4) or 68 Gy (Cases 1 and 3) delivered in 2 Gy fractions. The collimator angle was set to be 2°, the beam energy was 6 MV x-rays and the absorbed dose was calculated with pencil-beam convolution.
For each case, several plans were generated with different relative weighting of the objectives. The number of plans required to describe the system's trade-off in a complete way depends on the number of objectives involved and the uncertainty that can be accepted. In prior work, it was estimated that the number of plans should be at least N + 1 (where N is the number of objectives) [Citation17], but it has later been demonstrated that the number of required plans may increase drastically with higher number of objectives [Citation8]. We have therefore restricted the trade-off analysis to the VPTV,D < 95% and the <D>parotid gland altering only one objective (i.e. the objective for the ipsilateral parotid gland). It was previously hypothesised that even in two-dimensional (2D) cases, the shape of the Pareto surfaces is likely to be of important use for clinical decision-making [Citation8]. The objectives of the remaining structures were monitored such that they fulfilled the dose protocol applied [Citation14]. Details on the treatment planning procedure and the evaluation of GAO versus equidistant beam geometry are described in the following sections.
Inverse dose planning using IMRT
The intensity modulation of each beam is achieved using the MLC and can either be delivered with segmented MLC (SMLC), i.e. the absorbed dose is only delivered while the leaves are stationary combining static fields [Citation18,Citation19], or through dynamic MLC (DMLC) where the beam is on during the movement of the leaves [Citation20–22]. For the optimisation in Eclipse™ (Eclipse 8.0, Varian Medical Systems Inc., Palo Alto, CA, USA), DMLC was used. The fluence maps in Eclipse were generated in two steps, first by the optimal fluence and subsequently by the actual fluence. The optimal fluence represents the ideal modulation of the fields, not considering physical or mechanical limits of the MLC [Citation20–22]. To achieve less modulated DMLC sequences, priorities on the so-called smoothing parameters, x and y, were used (). The smoothing affects the separation between the two MLC banks (x direction) and helps minimise tongue and groove effects (y direction) [Citation22].
Table II. Machine specific parameters: minimum segment size (cm3) in OMP, smoothing parameters (x,y) in Eclipse, number of monitor units, MU with and without GAO in Eclipse and OMP, and the decrease in number of monitor units, MU, (%) by using GAO in both Eclipse and OMP.
In Oncentra® Masterplan (OMP v3.1 SP3, Nucletron B.V., Veenendaal, The Netherlands) the technique of SMLC was used, along with what is denoted as Direct Step-and-Shoot, i.e. the physical limitations for the MLC are incorporated into the optimisation process in order to satisfy the defined objectives [Citation19]. OMP supports direct optimisation of the MLC leaf positions. This removes the segmentation step where the MLC control points are created [Citation18,Citation19]. The physical limitations include the maximum number of segments along with the minimum allowed segment area () and also the minimum number of open MLC leaves per segment [Citation19].
The treatment planning in both Eclipse and OMP was performed according to standard procedure at each institution. For more details on this procedure we refer to references [Citation6] and [Citation10] where similar methods has been used for Eclipse and OMP, respectively. The optimisation continued for a preset number of iterations but was stopped as no further improvements in the objective function were seen. In each TPS, a treatment plan fulfilling the restrictions according to the clinical protocol [Citation14] and the ICRU guidelines [Citation15] was first created. In order to investigate the trade-off between one of the PTVs and the ipsilateral parotid gland, only the volume criterion on the latter structure was altered in a systematic way by subsequently increasing the relative weighting of its objective.
Gantry angle optimisation (GAO)
In Eclipse, the GAO was performed with the global optimisation following the local optimisation procedure. The global optimisation creates new coplanar beam geometries while the local optimisation only alters the gantry angles without changing the number of beams [Citation22]. The number of beams was restricted to seven and the most favourable angle combination considering the relative weighting on the objectives was generated. As the optimal angles were found, a new fluence optimisation was performed.
In OMP, only local optimisation is available which means that GAO proceeds from the original beam geometry, i.e. those angles used for the fluence optimisation with the procedure of the equidistant beam geometry. The gantry angles are updated during the GAO to improve the treatment plan [Citation19]. Due to this, a new fluence optimisation was performed with the angles generated from GAO.
It should be stressed that in both Eclipse and OMP only the relative weighting of the objectives for the ipsilateral parotid gland were altered with equal importance as with the fixed beam arrangement.
DVH evaluation
The <D>parotid gland was investigated as a function of the VPTV, D < 95% or D5-D95 for the PTV in the trade-off [Citation7]. A full coverage of the Pareto curve would require an infinite number of Pareto-optimal treatment plans created [Citation5]. Hence, due to lack of information in the region between the generated set of Pareto optimal data points collected for each of the four studied systems (Eclipse, Eclipse GAO, OMP and OMP GAO), a definition describing only the location of the Pareto optimal data points was introduced, the System Efficient Pareto Set (SEPS). This definition is a fairly simple approximation to the definition previously formulated by Craft [Citation8] although without any precautions on the non-convex nature of GAO. The SEPS was introduced in order to define a set of data points for each system studied such that the SEPS intrinsically includes all specific properties of each system (including the entire optimisation chain) and describes the prevalent trade-off through the generated treatment plans. More specifically, the SEPS for the four systems studied was defined by the set of data points generated for each particular system. In this way the SEPS allows an understanding of the mutual interaction of the different objectives in the trade-off. Different relative weighting on the set of objectives in the original treatment plans were considered being Pareto optimal data points as well and belonged to the same SEPS.
Number of monitor units (MU)
In order to assess the treatment plan complexity the number of monitor units, MU was recorded for all the generated plans for every patient case for both the DMLC (Eclipse) and the SMLC (OMP) delivery techniques. The number of MU calculated for the GAO plans were compared to the number of MU calculated for the plans with equidistant beam geometry with both DMLC and SMLC.
Results
DVH evaluation
The generated SEPS from the four different H&N cases are summarised in . They all display the general inverse relation between the PTV dose coverage (VPTV, D < 95%) and the mean OAR dose (<D>parotid gland). In , the PTV vs. OAR trade-off is instead shown as PTV homogeneity (D5-D95) as a function of the mean OAR dose (<D>parotid gland). The SEPS with, and without, GAO are separated for Cases 1 and 2 in the Eclipse system (). Using the OMP system however, the distinction is not so obvious. Focusing on the PTV homogeneity (), the behaviour of the SEPS is rather similar. The Eclipse GAO SEPS displays the most favourable trade-off for all H&N cases. There is a large difference in the scale on the x-axis (i.e. either VPTV, D < 95% or D5-D95) between the cases representing a larger PTV-parotid gland overlap (Cases 3 and 4) and the cases with less overlap (Cases 1 and 2). Hence, the steepness in the SEPS is much more pronounced for the cases with less overlap.
Figure 1. The average absorbed dose (Gy) to the parotid gland, <D>parotid gland, in the trade-off as a function of the under-dosage to the PTV, VPTV,D < 95%, with equidistant beam geometry and GAO in Eclipse and OMP.
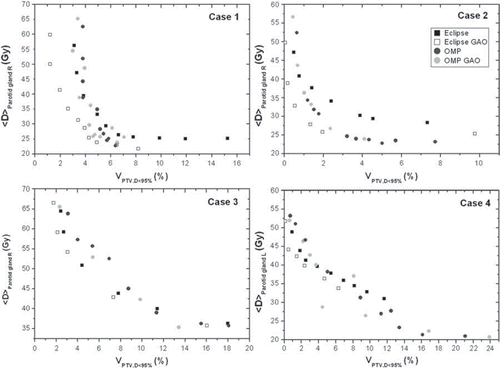
Figure 2. The average absorbed dose (Gy) to the parotid gland, <D>parotid gland, in the trade-off as a function of the dose homogeneity (D5-D95) in the PTV.
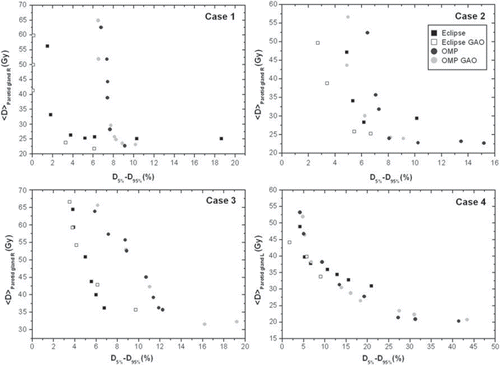
The number of acceptable plans (i.e. the plans not violating the criteria in [Citation14] and [Citation15] for the remaining structures only) created using GAO vs. equidistant beam geometry was 30 vs. 29 and 35 vs. 37 for Eclipse and OMP, respectively (). For the cases with a larger PTV-parotid gland overlap, an increase in the number of plans created was seen using GAO. It can be seen from that GAO resulting from the Eclipse system produces beams that are adjacent to the ipsilateral parotid gland for one case (Case 3) whereas the GAO in the OMP system produces beams that penetrate the parotid for three of the cases (Cases 1, 2 and 3) and is adjacent to the parotid for one case (Case 4). For two of the cases (Cases 1 and 3) the beam geometry obtained from the latter system penetrates the parotid for two of the seven beams.
Table III. The number of treatment plans generated with and without GAO in Eclipse and OMP.
Figure 3. The beam geometries obtained using GAO in the OMP system (black dots), in the Eclipse system (black dashed lines) and the equidistant beam geometry (grey solid lines) for the four cases calculated as average beam geometries for all treatment plans generated. The colours for the ipsilateral parotid/PTV in the trade-off are for Case 1 (upper left) blue/ red, Case 2 (upper right) blue/red, Case 3 (lower left) pink/red and Case 4 (lower right) green/red.
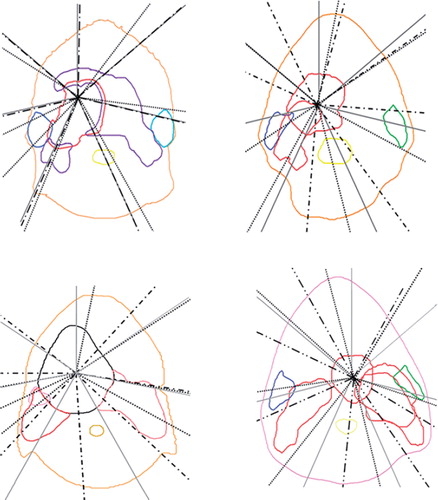
Number of monitor units
Utilising GAO, the number of MU decreased compared to the equidistant beam geometry () for both systems. On average the decrease in the number of MU from that of the equidistant beam geometry is 6% less for GAO in OMP and 18% less for GAO in Eclipse. Using Eclipse the decrease in the number of MU was 26% for the cases representing a larger PTV-parotid gland overlap (Cases 3 and 4) while only 11% for the cases with less overlap. The corresponding decrease using OMP was 8% and 7%, respectively. Overall, the required number of MU was considerably lower for SMLC (OMP) than DMLC (Eclipse).
Discussion
We have presented a method to compare two different beam arrangements, GAO vs. equidistant beam geometry, based on Pareto front evaluation for two commercial TPSs. For two different TPSs with different dose calculation algorithms, different delivery techniques and other different system properties it is almost impossible to create completely equal reference treatment plans and equal prerequisites [Citation5]. With this in mind, we aimed to restrict the number of degrees of freedom and keeping some of the parameters/properties as similar as possible by for instance limiting the number of beams to seven and restricting the trade-off situation to include only two conflicting structures-the PTV and the ipsilateral parotid gland. The differences related to clinical use of the two systems and their respective features are of course allowed in order to make a clinically relevant comparison.
For the four H&N cases studied, the SEPS created with the two TPSs using their different delivery techniques, exhibit different patterns for the GAO and the equidistant beam geometries though not always with an apparent separation between the SEPS. In general, a separation between the SEPS with and without GAO is more evident with the Eclipse system than with the OMP system ( and ). In addition, focusing on the decrease in the number of MU, the advantage of using GAO was much more pronounced for Eclipse (). These results are also somewhat reflected in the beam geometries obtained from GAO where the beams produced by the Eclipse system are only adjacent to the parotid gland for one case while GAO in the OMP system produces beams that penetrate the parotid gland for three cases and are adjacent for one case (). Interestingly, Ottosson and colleagues [Citation6] applied Pareto front evaluation to compare optimal fluence with deliverable fluence on several H&N cases using DMLC-based IMRT [Citation22]. Similar to some of the findings in this study, no obvious separation was found between the generated SEPS [Citation6]. Further, Pareto optimal plans were also created for an older version of OMP, however, lack of results for fair comparisons by the time of publication of that study [Citation6] limits the possibility for a complete comparison with our results.
The SEPS generated using GAO in Eclipse are generally more favourable as compared to the SEPS using GAO in OMP. The separation of the SEPS showed a different pattern when studying the under-dosage in the PTV rather than focusing on the homogeneity in the PTV ( and ). However, the order in terms of a better/worse SEPS was equal for both evaluation methods. The patient cases represent different PTV-parotid gland overlap. This is to some extent confirmed by the slope of the SEPS, with a more steep slope for the ‘less complex’ cases with a minor overlap (Cases 1 and 2) than for the cases with a larger overlap, i.e. the ‘complex’ cases (Cases 3 and 4), resulting in different PTV dose coverage and parotid sparing. For one of the complex cases (Case 3) the GAO obtained from the OMP system additionally penetrates the ipsilateral parotid gland for two of the seven beams (). Comparing the performance of using GAO for the complex and the less complex cases, the decrease in the number of MU was more evident with Eclipse (). In a recent study [Citation10] where similar methods have been applied on three H&N cases, the dependence of volume overlap was confirmed. It was demonstrated that GAO (OMP) was more effective than equidistant beam geometry when a larger PTV-parotid gland overlap was presented. Investigating the PTV-rectum overlap for three prostate cancer patients, the dependence of volume overlap was however less pronounced. This may be due to differences in prescription dose and the use of a different treatment site relative to the current study. As part of a back-up solution for a specific rotational IMRT TPS, Pareto front evaluation was used to investigate the effect of a varied number of beams (7, 11 and 15 beams) [Citation10]. Using the previously mentioned three H&N cases, it was revealed that an increase in the number of beams was more advantageous for the cases with a larger PTV-parotid gland overlap. Even though five to nine beams have previously been shown to be sufficient to achieve acceptable dose homogeneity within the target volumes along with substantial parotid sparing [Citation12,Citation16], the findings in the study by Petersson et al [Citation10] would support the use of further increase in the number of beams in this study at least for the complex cases (Cases 3 and 4). However, an increased number of beams implies a larger total number of beam segments, and in the interest of treatment efficacy, it may therefore be valuable to find the optimal beam angles.
The average number of treatment plans created for both DMLC and SMLC with the equidistant beam geometry vs. the GAO geometry was equivalent (33 vs. 33 plans) (). For the complex cases (Cases 3 and 4) GAO results in a slightly higher number of acceptable treatment plans. This may indicate the advantage of using GAO when the anatomic architecture is rather complicated although we acknowledge the overall sparse number of acceptable treatment plans [Citation6,Citation8].
The use of GAO may lead to a plan with new gantry angles, more suited to fulfil the objectives and constraints set for the current case [Citation10]. Nevertheless since the GAO in OMP only searches for new angles close to the current gantry angles, the result of the GAO is dependent on the initial angles selected by the planner [Citation19–21]. In contrast, the GAO optimisation in Eclipse searched through all possible gantry angles [Citation16,Citation22]. Hence, the latter solution of solving the GAO might have been more advantageous for the cases used in this study ().
The interpretation of the SEPS separation is somewhat arbitrary. An important improvement would therefore be to introduce a scalar derived from the SEPS, allowing for a quantitative comparison between different systems, particularly useful for situations where there is no apparent knee in the Pareto curves [Citation5,Citation8,Citation11,Citation23]. One possible way to facilitate such a scalar measure could be to use normalised quantities for the PTV coverage and OAR sparing, respectively. We acknowledge that the SEPS might include suboptimal plans, i.e. locally Pareto-optimal or even non-Pareto optimal plans; hence, better solutions might exist [Citation6,Citation11,Citation24]. This non-convexity could be solved practically by generating Pareto surfaces for a large set of beam configurations and simultaneously providing a method to enable navigation between the collections of surfaces [Citation11]. Alternatively, the properties of preserved local convexity of GAO could be utilised to solve the optimisation problem as proposed by Teichert et al. [Citation24]. Anyhow investigating the Pareto sub-optimality has not been part of this initial study but might be addressed in the further developments. Finally, the optimisation generally includes multiple target structures and OARs, resulting in a large amount of effective trade-offs. Using higher dimensional Pareto surfaces rather than using 2D Pareto fronts is likely to be helpful towards a more clinical setting. This might open up for studying different trade-offs, different amount of sparing of sensitive structures not chosen for the trade-off and other adverse end-points in the parotid gland [Citation6,Citation8].
In the present study we have compared GAO to equidistant beam geometry based on the information from multiple treatment plans. We have found differences in the effectiveness of GAO compared to equidistant beam geometry in handling conflicting trade-offs for two commercial inverse TPSs (Eclipse and OMP). The SEPS with versus without GAO was more separated with the Eclipse system than with the OMP system. The SEPS showed that Eclipse GAO displays the most favourable trade-off in all cases. Besides, using GAO an overall decrease in number of monitor units was observed. The understanding of conflicting trade-offs and the future developments of thorough comparison techniques are likely to benefit from extension of the scope of the comparison to include information from a range of treatment plans as developed in this study.
Acknowledgements
The authors are thankful for financial support from the John and Augusta Persson foundation.
Declaration of interest: The authors report no conflicts of interest. The authors alone are responsible for the content and writing of the paper.
References
- Djajaputra D, Wu Q, Wu Y, Mohan R. Algorithm and performance of a clinical IMRT beam-angle optimization system. Phys Med Biol 2003;48:3191–212.
- Nazareth DP, Brunner S, Jones MD, Malhotra HK, Bakhtiari M. Optimization of beam angles for intensity modulated radiation therapy treatment planning using genetic algorithm on a distributed computing platform. J Med Phys 2009; 34:129–32.
- Censor Y. Mathematical optimization for the inverse problem of intensity-modulated radiation therapy. In: Palta JR, Mackie TR, editors. Intensity-modulated radiation therapy: The state of the art: AAPM Medical Physics Monograph 29. Madison, WI: Medical Physics Publishing; 2003. p. 25–49.
- Ottosson RO, Karlsson A, Behrens CF. Pareto front analysis of 6 and 15 MV dynamic IMRT for lung cancer using pencil beam, AAA and Monte Carlo. Phys Med Biol 2010;55: 4521–33.
- Holdsworth C, Kim M, Liao J, Phillips MH. A hierarchical evolutionary algorithm for multiobjective optimization in IMRT. Med Phys 2010;37:4986–97.
- Ottosson RO, Engström PE, Sjöstrom D, Behrens CF, Karlsson A, Knöös T . The feasibility of using Pareto fronts for comparison of treatment planning systems and delivery techniques. Acta Oncol 2009;48:233–7.
- Steneker M, Lomax A, Schneider U. Intensity modulated photon and proton therapy for the treatment of head and neck tumors. Radiother Oncol 2006;80:263–7.
- Craft D. Calculating and controlling the error of discrete representations of Pareto surfaces in convex multi-criteria optimization. Physica Medica 2010;26:184–91.
- Boyd S, Vadenberghe L. Convex optimization. Cambridge: Cambridge University Press; 2004. p. 177–87.
- Petersson K, Ceberg C, Engström P, Benedek H, Nilsson P, Knöös T. Conversion of helical tomotherapy plans to step-and-shoot IMRT plans-Pareto front evaluation of plans from a new treatment planning system. Med Phys 2011;38: 3130–8.
- Craft D, Monz M. Simultaneous navigation of multiple Pareto surfaces, with an application to multicriteria IMRT planning with multiple beam angle configurations. Med Phys 2010;37:736–41.
- Wu Q, Manning M, Schmidt-Ullrich R, Mohan R. The potential for sparing of parotids and escalation of the biologically effective dose with intensity-modulated radiation treatments of head and neck cancers: A treatment design study – A concept of equivalent uniform dose. Int J Radiat Oncol Biol Phys 2000;46:195–205.
- Eisbruch A, Ten Haken RK, Kim HM, Marsh LH, Ship JA. Dose, volume, and function relationships in parotid salivary glands following conformal and intensity-modulated irradiation of head and neck cancer – The critical volume model. Int J Radiat Oncol Biol Phys 1999;45:577–87.
- Danish Head and Neck Cancer Group. Retningsliner for strålebehandling af hoved-hals cancer (Cavum Oris, Pharynx, Larynx) inklusiv IMRT vejledning. Aarhus: DAHANCA; 2004. p. 1–30.
- Prescribing, recording and reporting photon-beam intensity-modulated radiation therapy (IMRT). ICRU Report 83. J ICRU 2010.
- de Kruijf WJM, Heijmen BJM, Levendag PC. Quantification of trade-off between parotid gland sparing and planning target volume underdosages in clinically node-negative head-and-neck intensity-modulated radiotherapy. Int J Radiat Oncol Biol Phys 2007;68:136–43.
- Craft D, Bortfeld T. How many plans are needed in an IMRT multi-objective plan database? Phys Med Biol 2008;53: 2785–96.
- Van Asselen B, Dehnad H, Terhaard CHJ, Lagendijk JJW, Raaijmakers CPJ. Segmental IMRT for oropharyngeal cancer in a clinical setting. Radiother Oncol 2003;69:259–66.
- Oncentra® Masterplan V.3.1-Physics and algorithms 2008.
- Chui CS, Chan MF, Yorke E, Spirou S, Ling CC. Delivery of intensity-modulated radiation therapy with a conventional multileaf collimator: Comparison of dynamic and segmental methods. Med Phys 2001;28:2441–9.
- Alaei P, Higgins PD, Weaver R, Nguyen N. Comparison of dynamic and step-and-shoot intensity-modulated radiation therapy planning and delivery. Med Dosim 2004;29:1–6.
- Varian Medical Systems. Eclipse Algorithms Reference Guide (P/N B500298R01C). Palo Alto, CA: V M Systems; 2007.
- Montero JP, Fenwick JD. An approach to multiobjective optimization of rotational therapy. II. Pareto optimal surfaces and linear combinations of modulated blocked arcs for prostate geometry. Med Phys 2010;37:2606–16.
- Teichert K, Süss P, Serna JI, Monz M, Küfer KH, Thieke C. Comparative analysis of Pareto surfaces in multi-criteria IMRT planning. Phys Med Biol 2011;56:3669–84.