Abstract
Determination of optimal clinical target volume (CTV) margins around gross tumour volume (GTV) for modern radiotherapy techniques, requiring more precise target definitions, is controversial and complex. Tumour localisation has been greatly improved using molecular imaging integrated with conventional imaging techniques. However, the exact incidence and extent of microscopic disease, to be encompassed by CTV, cannot be visualised by any techniques developed to date and remain uncertain. As a result, the CTV is generally determined by clinicians based on their experience and patients’ histopathological data. In this article we review histopathological studies addressing the extent of subclinical disease and its possible correlation with tumour characteristics in various tumour sites. The data have been tabulated to facilitate a comparison between proposed margins by different investigations and with current margins generally accepted for each tumour site. It is concluded that there is a need for further studies to reach a consensus on the optimal CTV pertaining to each tumour site.
The recent technological advances in treatment techniques have led to further development of three dimensional conformal radiotherapy (3DCRT), leading to intensity modulated radiation therapy (IMRT), image guided radiation therapy (IGRT), and gated radiotherapy. These techniques offer the advantage of decreasing the volume of irradiated normal tissue and a more conformal dose distribution to irradiated target volumes. However, improved treatment outcomes from these new techniques depend strongly on the accurate determination of intended target volumes.
In order to treat the disease successfully, the entire population of clonogenic cells within the gross tumour and its microscopic extension (ME), i.e. parts of tumour which are not within the resolution of imaging techniques, has to be eradicated. According to ICRU report 50, the “clinical target volume (CTV) is a volume encompassing visible gross tumour volume (GTV) and subclinical malignant disease” [Citation1]. As a result of imaging modalities limitations, the exact incidence and extent of microscopic disease remains uncertain and the delineation of a CTV that contains the original lesion including any possible invasion beyond gross tumour or nodal microscopic spread is empirical in most instances. Indeed, there is no consensus on whether CTV margins are unnecessarily generous [associated with toxicity of normal tissue and increased normal tissue complication probability (NTCP)] or inadequate [associated with poor treatment efficacy and therefore reduced tumour control probability (TCP)]. Therefore, both large and tight margins could yield local failure and an optimal CTV that keeps NTCP and TCP in balance is desired. Hence CTV represents the most controversial irradiation volume to be defined.
Current radiotherapy treatment modalities like IMRT especially require precise determination of target volumes. In addition, due to more accurate organ specification provided by IGRT the set up errors have been minimised, allowing the PTV margin to be reduced. Despite these advances in treatment modalities and imaging techniques, the uncertainty regarding microscopic extension incorporated within the CTV margin limits further reduction of irradiated volumes since the risk of missing a part or even a few cells of malignant disease is increased. To have an estimation of the extent of the risk, it is worth noting that the Poisson distribution model of TCP (i.e. TCP = e2n(D) where n(D) is the expected number of surviving clonogens) suggests that one single cell surviving radiation therapy will reduce TCP to 37%. While this definition is a crude approach to TCP assessment, as it does not consider host contribution to eradication of last few cells (such as immune response, bystander effect, and vascular damage), it is the most widely accepted cell-survival model for clinical use. Overall, to take the advantage of current treatment modalities and their capacity to reduce irradiation target volumes, an understanding of distribution patterns of microscopic growth is required.
The aim of this study is to review and aggregate histopathological studies evaluating microscopic extension of tumours quantitatively in various tumour sites for potential use for bio-mathematical modelling. Predictions of the pattern of subclinical disease provided by developed models (current and future) can serve as a guideline for clinicians to define the CTV margin as precisely as possible and to improve therapeutic ratio. Moreover, this information can be considered for future inclusion into treatment planning systems (TPSs). Literature search was performed over the past 12 months. US National Library of Medicine, PubMed, was explored with the following keywords used either separately or in various combinations: tumour microscopic extension; clinical target volume; subclinical disease; infiltration; extracapsular extension. In addition, references of articles found in the library were searched seeking for relevant studies.
Clinical findings
A number of studies have investigated microscopic extension of gross tumours in various tumour sites. In this article, we review the histopathological studies addressing the incidence and extent of microscopic disease and also its correlation to tumour characteristics such as anatomic location, tumour size, histologic type and grade in different organs. The most commonly reported sites in the literature are lung, brain, prostate and breast and the findings regarding the ME are presented in the following sections.
Lung carcinoma
Lung carcinoma has become the top of 10 leading cancer types in terms of reported death among both male and females with slightly higher incidence among men compared to that of women [Citation2]. Despite recent advances in radiotherapy, the survival rate has continued to be relatively low with little improvement in the course of the past 30 years [Citation2,Citation3], particularly for unresectable and non-localised tumours. This statistics implies that the local control cannot be achieved in the majority of cases and thus research to define appropriate target volume which encompasses the whole tumour including its subclinical contents (without compromising critical normal tissue protection) is still needed. Several studies have examined infiltration beyond macroscopic tumour and the discrepancy between the real extent of tumour and what is detected by imaging techniques. In addition, possible correlation of the incidence and extent of ME with biological parameters such as nuclear grade, tumour size and type was investigated. These correlations can be used as prognostic factors and illustrate the complexity of microscopic tumour extension.
Giraud et al. [Citation4] has undertaken a histopathological investigation of the incidence and extent of microscopic extension beyond GTV in 70 patients having non-small cell lung cancer (NSCLC). Forty-five percent of all patients were diagnosed with adenocarcinoma (ADC) and 55% with squamous cell carcinoma (SCC). No correlation was found between the extent of microscopic invasion and the size of gross tumour. The mean microscopic extension was found to be 2.69 mm (0–12 mm) for ADC and 1.48 (0–13 mm) for SCC. Given the distribution of microscopic extension, a margin of 8 mm for ADC and 6 mm for SCC around GTV was proposed to be adequate to encompass 95% of disease. These results do not agree with the conventionally used CTV margin reported in the literature to be 5–7 mm for ADC [Citation5,Citation6] and 5 mm for SCC [Citation7]. In particular, for ADC patients, the current treatment covers only 80% of subclinical disease (based on histograms generated in the study of Giraud) which is clearly associated with poor local control.
In the study of Li et al. [Citation8] the mean ME measured on surgical specimens of 43 patients with NSCLC, was 2.18 mm for ADC and 1.33 mm for SCC. It was also concluded that a margin of 7 mm for ADC and 5 mm for SCC is required to cover 95% of the disease. These margins are in sensible agreement with those proposed by Giraud.
In a more elaborate analysis of ADC, Grills et al. [Citation9] histopathologically measured surgical specimens of 35 patients with adenocarcinoma of lung. Along with ME, gross tumour size, nuclear grade and the extent of lepidic growth were analysed so as to be correlated with ME. Bronchioloalveolar carcinoma (BAC) is a subtype of adenocarcinoma which “shows growth of neoplastic cells along pre-existing alveolar structures (lepidic growth) without evidence of stromal, vascular or pleural invasion” [Citation10]. A negative correlation between ME and both nuclear grade and BAC involvement was found. Margins of 9 mm, 7 mm and 4 mm were proposed for grade 1, 2, and 3 of NSCLC adenocarcinoma, respectively, to encompass the ME with 90% confidence.
Another histopathological study on surgical resection specimens of 243 patients with NSCLC was conducted by Yuan et al. [Citation11] to quantify the extent of ME beyond nodal GTV. The extent of nodal extra capsular extension (ECE) on pathologically-dissected lymph nodes was measured and the correlation between ECE and various lymph node sizes (<10 mm, 10–19 mm, 20–30 mm), nodal stage, nodal station, histological type, and tumour cell differentiation (well, moderate, poor) was studied. The ECE incidence positively related to lymph node size and inversely correlated to distance from capsule. Also, positive correlations between ECE extension and lymph node size, differentiation and nodal stage were observed. For lymph node sizes ≤20 mm and ≥20 mm, margins of 3 mm and 8 mm around GTV were proposed, respectively, as indicated in the Supplementary Appendix Table I to be found online at http://informahealthcare.com/doi/abs/10.3109/0284186X.2012.720381. For comparison, nodal CTV margin around GTV applied for NSCLC typically ranges from 0–25 mm at various institutions [Citation12]. Some of the limitations of Yuan's study to be mentioned here are: 1) 2D measurement of ECE (thus part of ECE would have been missed); 2) tissue shrinkage during fixation; and 3) inter-observer variations not being considered.
Investigations of bronchial extension of lung cancer have also resulted in quite varying conclusions. Cotton et al. [Citation13] examined surgical specimens of 100 patients with lung cancer and showed that at least a margin of about 1.9 cm should be allowed around gross tumour. However, Kara et al. [Citation14] reported maximum ME of 3 cm for ADC and proposed a margin of 1.5 cm around GTV to encompass the disease in 93% of cases.
Prostate cancer
Prostate carcinoma is the second leading cause of death among cancer cases and the most common cancer among men, accounting for around one third of all diagnosed cancer incidences [Citation2]. The high survival rates for localised prostate cancer which drops substantially for cases with distal disease spread indicate that while localised disease is amenable to treatment, the tumour control is not achieved for tumours with infiltration. More importantly, despite technological advances in radiotherapy, the survival rate for infiltrative cases has further declined in recent years [Citation2,Citation3]. This could have been caused as a result of the microscopic cells being missed following the reduction of irradiation target volumes in modern treatment techniques. The microscopic extension of prostate tumours or extra prostatic extension (EPE), generated by tumour either piercing through capsule or extending out through surrounding tissue, is associated with poor prognosis and increased recurrence rate. Therefore, to provide an efficient treatment with either external beam radiotherapy or brachytherapy, EPE has to be taken into account in the course of target planning. However, the incidence and extent of EPE is unknown and is estimated based on pathological findings. It is known that the incidence and extent of EPE is correlated with certain pre-treatment characteristics like prostate-specific antigen (PSA), T stage and biopsy Gleason score (bGs) [Citation15–17].
The clinico-histopathological studies aiming to quantify the extent of EPE in prostate cancer started two decades ago by early works of Stamey et al. [Citation18] and Epstein et al. [Citation19]. Later, Davis et al. [Citation20] examined the radial distances of EPE (EPEr) and their correlations with pre-operative and pre-treatment parameters in a series of 376 radical prostatectomy (RP) specimens of patients with organ-confined prostate cancer. Among those, 105 specimens contained measurable disease for those patients with a mean PSA level of 17.9 and clinical stage of T1–2. The EPE was found in the range of 0.04–4.4 mm and it was concluded that a 3–5 mm margin is a valid criterion for early stage prostate cancer to be treated with brachytherapy.
Sohayda et al. [Citation21] evaluated extracapsular extension by performing histopathological measurements on 265 RP specimens, among which 92 contained EPE. Prostate tumours were classified as favourable (Stage T1–2, PSA ≤10, bGs ≤6) and unfavourable (Stage T3, PSA ≥10, bGs ≥7). The median extent of EPE for the unfavourable group was evaluated to be 1.3 mm which was higher than that of favourable group, i.e. 0.6 mm. However, the range of EPE was significantly higher for the unfavourable group (0.1–5 mm vs. 0.1–10 mm) thus a margin of 4 mm was proposed with approximately 90% coverage of the disease.
An investigation of prostatectomy specimens from 712 patients among which 185 specimens contained measurable radial extent of disease was conducted by Teh et al. [Citation22,Citation23] to quantify the extent of EPE aimed to be used in target planning for IMRT treatment. The mean value of PSA level was 13.73 and 89% of patients had T1 and T2 stage tumours. In contrast with previous studies, the shrinkage factor due to fixation was taken into account in this study. Compared to the study of Davis, a greater range of EPEs were found in the work of Teh (0.5–12 mm vs. 0.04–4.4 mm). This result could have been due to the inclusion of patients with stage T3 and also greater mean PSA values in the study of Teh compared to those of Davis's. Teh concluded that a margin of 5 mm is sufficient to encompass subclinical EPE in 97.2% of patients.
Chao et al. [Citation17] performed a histopathological review of 371 prostatectomy specimens for ADC prostate cancers stage 1 and 2 (stage 3 was excluded) to correlate linear EPE with clinical features. The same classification as that of Sohayda [Citation21] for favourable and unfavourable tumours was considered. The radial EPE occurred primarily posterolaterally with the range of 0.05–5 mm and 0.05–7 mm for favourable and unfavourable groups, respectively. It can be inferred that the exclusion of stage T3 in Chao's study underlies the discrepancy in the range of EPE for the unfavourable group when compared to Sohayda's work.
Schwartz et al. [Citation24] investigated the correlation between EPEr with pre-operative and pre-treatment findings to develop a model predicting EPEr with respect to pre-treatment factors. The RP specimens of 404 patients were histopathologically analysed to identify determinant variables of EPEr. Histological T-grade, PSA level, and the percentage of cancer involvement in biopsy cores were found to be correlated with EPEr. On the basis of the measured median of EPEr, which was 0.6 mm (0–5.7 mm range), a three-category mathematical model was developed: no EPE, near median EPE (range: 0–0.59 mm), and equal or greater than medium (far) EPE (≥0.6 mm). A hierarchical predictive structure was designed for each of these EPEr categories. The first three levels of each hierarchy are PSA (0–4, 4.1–10, 10.1–20, >20 ng/mL), T-stage (T1–T2a, T2b, T3), and percentage of cancer in biopsy cores (10%, 50%, 90%) followed by the last level predicting the risk that a patient would have an EPEr associated with that hierarchy. These data are useful when defining the CTV for external beam radiotherapy as well as permanent prostate brachytherapy based on known pre-treatment factors. However, further investigation to identify factors associated with EPE beyond 3–5 mm is yet required.
Brain cancer
Gliomas, the most common brain tumours, are notorious for their aggressive proliferation and extensive invasion to normal tissue before any symptoms are revealed. Glioblastomas are the most aggressive gliomas with a very high fatality rate (i.e. at best scenario, 27.2% survival rate at two years when concomitant and adjuvant chemotherapy and radiotherapy are administered [Citation25]). The high rate of fatalities imply inadequate treatments and since the decision of how far CTV is extended from the border of GTV is probably the most uncertain step in radiotherapy planning, CTV delineation appears to be even more controversial in brain cancer and requires an extensive investigation. It is worth noting that, apart from microscopic extension uncertainty, intrinsic radioresistance of glioblastoma stem cells is also another major factor of tumour relapse. Jansen et al. [Citation26] reviewed the literature regarding high grade gliomas and accumulated pre- and/or post-radiotherapy computed tomography (CT)/magnetic resonance imaging (MRI) images together with associated recurrence patterns and tumour delineations. The data were tabulated and studies were categorised based on two treatment modalities: whole brain irradiation (WBI) and partial brain irradiation (PBI). It was demonstrated that the recurrence pattern is roughly independent of the modes of treatment used (WBI and PBI). Given this finding and that WBI is associated with higher risk of normal tissue toxicity compared to PBI, switching from WBI to limited field irradiation was recommended. A successful implementation of PBI, however, requires more critically understanding of the exact extent of microscopic extension of a tumour. The analysis of above-mentioned data showed that 90% of tumour extension is within CTV (contrast-enhancing mass) plus 2 cm margin. For large tumours (CTV >250 cm3), it was suggested to define a second target volume (TV) to GTV by 1 cm (i.e. TV is smaller than CTV). The reason for choosing another target volume (TV) was to enable delivering a boost dose to TV (e.g. >40–50 Gy) so as to improve local control (TCP) while limiting normal brain tissue toxicity. Jansen argued against the previously-suggested CTV of 2–3 cm beyond GTV with the boost field of 2–2.5 cm [Citation27], on the grounds that the majority of local recurrence is at the primary site of tumour so the expansion of CTV just leads to increased normal brain tissue toxicity.
For target definition of glioblastoma in radiation therapy planning, several studies have used the contrast enhanced area on MRI image and/or peritumoral edema area on CT image [Citation28–30]. For example, in the study of Urtasun et al. [Citation29], the CTV was defined as the visible lesion (GTV) plus peritumoral edema enclosed by 2 cm margin. The boost volume was considered as the contrast-enhanced area (GTV) plus 2.5 cm margin. Matsuo et al. [Citation31] conducted a study to quantify the extent of viable GBM tumour cells by comparing MRI and carbon-11-labelled Methionine PET ([11C] MET-PET). The MRI and PET images were acquired for 32 patients diagnosed with GBM within two weeks after surgery. Since the uptake of [11C] MET is distinctively lower by normal brain parenchyma, [11C] MET-PET is known to have high sensitivity for detection of gliomas [Citation32]. Therefore, the [11C] MET-PET images of patients served as the standard for evaluation of MRI-based defined CTVs. CTV-T2 (x mm) and CTV-T1 (x mm) which were defined as x-mm margin outside Gd-enhanced T1-weighted MRI and high intensity area on T2-weighted MRI, respectively, were compared with PET image-based CTV and corresponding sensitivity and specificity parameters were derived. It was demonstrated that a margin of 2 cm to the T2-MRI (i.e. which is post-operative peritumoral edema plus 2 cm) is necessary to achieve optimal tumour control and prevent local failure (this margin results in 96% sensitivity). However, another study by Minniti et al. [Citation33] evaluating different target volume definitions in terms of their associated recurrence patterns in 105 GBM patients, concluded that plans including expansion of edema by 2 cm are associated with greater risk of late radiation-induced toxicity without offering a considerable improvement in recurrence patterns as compared to plans where CTV includes residual tumour and resection cavity plus 2 cm.
Stereotactic radiosurgery (SRS) of the brain is a surgical intervention that utilises a 3D coordinate system to locate particular targets inside a brain and to ensure immobility and proper positioning of a patient. Multiple radiation beams converge on the target in question and deliver a high-dose radiation in a single fraction to eradicate the disease, while sparing adjacent normal tissue. The accurate definition of CTV is of crucial importance in the successful implementation of this technique. There are discrepant opinions between institutions regarding the necessity and the extension of CTV for brain metastasis to be treated with SRS. Among studies focusing on the evaluation of SRS alone or together with WBRT in terms of its associated TCP and recurrence rates, some authors reported a margin of 2 mm beyond a contrast-enhancing lesion [Citation34,Citation35] whereas others considered no further margin around visible tumour margin on CT/MRI images [Citation36,Citation37]. More recently, an analysis conducted by Noel et al. [Citation38] on 61 patients’ data assessed the merit of different definition of CTV (GTV + 1 mm and GTV + 0 mm) with respect to their corresponding TCP. It was concluded that when a margin of 1 mm was added to GTV, the local tumour control was significantly improved, from 54% to 92% at 18 months, while the incidence of complications remained unchanged. This is in contrast with the results of a recent analysis performed by Nataf et al. [Citation39] who compared the outcome of SRS with 2 mm margin (51 patients) and without margin (42 patients). They found that adding 2 mm margin not only lacked better local tumour control but also resulted in severe parenchymal complications. Hence, this approach was not recommended. In contrast, based on the study of Soltys et al. [Citation40], post-operative SRS need not only to target the surgical bed but also a 2 mm margin beyond. This recommendation was supported by an evaluation study of the effect of target volume margin on tumour control conducted by Choi et al. [Citation41].
Baumert et al. [Citation42] histopathologically analysed specimens from 45 patients with brain metastases (intra-parenchyma) in order to quantify the extent of infiltration and to suggest a reliable margin for CTV to be used in SRS. The correlation between depth of infiltration and histologic origin was also investigated. It was observed that microscopic growth patterns and infiltration differed for brain metastasis with different histological origins. Therefore, depending on histology, appropriate margins were proposed, as indicated in the Supplementary Appendix Table I to be found online at http://informahealthcare.com/doi/abs/10.3109/0284186X.2012.720381. According to the results, addition of a margin of almost 1 mm around the GTV using MRI-based planning was recommended. The result of Baumert's study supports the outcome of Noel's investigation (i.e. the addition of 1 mm margin to the GTV in patients with brain metastasis improves TCP) [Citation38]. There are other studies in favour of adding a margin for brain metastasis, e.g. Yoo et al. [Citation43] demonstrated that even after en bloc resection with 5 mm margin, the risk of local relapse remained significant and was corrected by WBRT.
In contrast, a more recent study by Raore et al. [Citation44] evaluating brain infiltration of metastatic tumour cells to be used in CTV planning in SRS led to differing results. Microscopic examination of 12 resection specimens revealed no evidence of infiltrating malignant glial cells, except for glioblastoma. Hence, given the adverse side effects of SRS (normal brain tissue toxicity) this study suggests that there is no need for CTV and only 1–2 mm margin for set-up and planning uncertainties is sufficient.
Breast cancer
Breast cancer is the most commonly diagnosed cancer type and the second high cause of cancer death among women [Citation2,Citation3]. The standard treatment for breast carcinoma has been either simple/radical mastectomy or breast-conserving therapy (BCT) which is the removal of the primary tumour followed by radiotherapy to eradicate residual subclinical disease. The decision on whether a patient is eligible for BCT is highly disputable since the extent of subclinical disease, in form of residual invasion, intraductal carcinoma in situ (DCIS), and invasive lobular carcinoma, is unknown. To overcome this shortcoming and provide a scientific basis for determination of the extent of surgery resection as well as CTV margin for following RT, several investigations have been carried out. These studies aimed to correlate microscopic extension in its various forms with clinico-pathological factors such as the presence or absence of micro calcification or DCIS and age.
Aiming to study tumour multifocality of breast cancer, Holland et al. [Citation45] examined mastectomy specimens of 406 patients. The probability distribution of tumour foci with respect to the distance from pathologically estimated gross tumour for different gross tumour sizes (<2 cm, <4 cm, <5 cm) were acquired. This ranged from 0 cm and decreased, approximately exponentially, to 9 cm from gross tumour. It was found that 90% of residual disease is encompassed within 30 mm margin. Given that surgeons allow a wide excision margin of around 10–20 mm, it is concluded from this data that a margin of 10–20 mm from tumour bed (TB) is sufficient [Citation46].
Based on clinical data analysis, it has been postulated that the presence of an extensive intraductal component (EIC) is associated with increased risk of local recurrence [Citation47–50]. A positive EIC is defined as a DCIS when 25% or more carcinoma cells are growing in intraductal compartments. To justify this observation, Holland et al. [Citation51,Citation52] differentiated between specimens with and without EIC and examined the presence of microscopic disease (tumour multi-foci) in each group. EIC( + ) tumours were found to be more likely to have microscopic residual extension as compared to EIC(-) specimens (74% vs. 42%). Hence, the underlying reason for association of EIC and recurrence was found to be subclinical disease which was more probable to occur for tumours with EIC. This study also reports the presence of microscopic disease at distances >2 cm from gross tumour in 29% of cases. More specifically, microscopic disease at distances >2 cm from gross tumour was found in 47% and 16% of cases for two histologically sub classified groups in terms of nuclear grade, the extent of necrosis and architectural pattern: micropapillary and comedo (corresponding to high nuclear grade and substantial necrosis) types, respectively.
In contrast to the studies of Holland [Citation45,Citation52], more recent publications reveal that the microscopic disease is rarely beyond 1 cm [Citation53,Citation54]. For example, Ohtake et al. [Citation53] examined quadrantectomy specimens of 20 patients with invasive breast cancer to quantify the extent of microscopic DCIS and found residual DCIS with the median extension of 6 mm (0–28 mm range). Also, data analysis of the distance of extensions corresponding to various clinico-pathological factors revealed that intraductal extension was inversely correlated to the age of patients, ranging from 8 mm for women older than 50 years to 23 mm for women between 30 and 39 years old.
Breast conserving therapy has been embraced as the mode of treatment to manage early-stage breast cancer patients due to comparable outcome of BCT with that of radical mastectomy in terms of tumour control and recurrence rate [Citation55]. However, the long course of whole breast irradiation following lumpectomy does not seem to be necessary since the incidences of recurrence mostly occur at close proximity to the TB [Citation55,Citation56]. Hence, an alternative mode of treatment was proposed in which lumpectomy is followed by RT restricted to the TB with appropriate margins at a higher dose over a shorter time [Citation57]. This technique is called accelerated partial breast irradiation (APBI) and is found to be a very effective mode of treatment illustrated by the five years follow-up (e.g. tumour control) equivalent to conventional BCT [Citation57,Citation58]. Nonetheless, the optimal CTV of breast tissue requiring post-lumpectomy irradiation has not been clearly defined.
Vicini et al. [Citation59] histopathologically examined specimens of 333 patients who underwent reexcision after lumpectomy and before RT, to quantify the distance and amount of subclinical disease on reexcision specimens. A classification for margins, defined as negative vs. positive, was the major parameter determining the extent of invasion. The original lumpectomy was inked. Invasive carcinoma (IC) margin on original lumpectomy was classified as negative when IC is situated more than half low-power field (LPF) (i.e. the area of a slide visible under the low magnification system of a microscope) from inked margin ( = 4.2 mm), and positive when IC is present at inked margin. Patients with positive margins were excluded due to protocol restrictions whereby APBI is not allowed for patients with positive margins in their lumpectomy. Reexcision specimens were classified into two groups based on the type of residual disease they contain: DCIS only, invasive carcinoma, and either invasive carcinoma or DCIS. It was shown that among 134 patients having negative margin, 90% had an invasion <10 mm from the edge of the original lumpectomy (TB) so a margin of 10 mm beyond TB will be adequate to encompass the disease for these patients. Moreover, a correlation between features of original excision (e.g. the extent of microscopic invasion and the number of DCIS ducts) with the likelihood of subclinical disease (DCIS or invasive carcinoma) in reexcision specimens was found. This correlation allowed identifying patients with disease extending beyond 10 mm based on their initial excision features, such that patients whose lumpectomies contain more than 3 mm invasive carcinoma or more than 13 DCIS ducts near the margin are likely to have microscopic disease beyond 10 mm. This study supports Holland's data [Citation45] which was the basis for the current protocol for TB-CTV of 15 mm to be applied in APBI. However, this criterion has recently been argued by Kirby et al. [Citation60] on the ground that uniform 15 mm margin for TB-CTV could lead either to missing a part of subclinical disease or impose irradiation risk on healthy tissue unnecessarily encompassed (since this criterion is valid as long as excision margin is uniform in three dimensions). Since current histopathological analysis of surgical resections has revealed that 3D excision margins are often asymmetric [Citation61], it was recommended to consider an asymmetric CTV which is defined as 30 mm minus the surgical excision margins in three dimensions.
Such asymmetrically-defined CTV margins were applied in the study of Stroom et al. [Citation62]. In this study, a histopathological examination was performed on 38 specimens of 10 lumpectomy patients treated by external RT. As the recurrence occurs in the tumour bed in the majority of cases, in post-lumpectomy external radiation therapy, in addition to whole breast irradiation, a radiation boost is applied to the tumour bed to improve TCP. The volume of the radiation boost (boost volume) consists of the TB or excision cavity enclosed by a CTV margin which accounts for subclinical disease present after surgery. The boost volume should be reduced to minimise side effects to normal tissue while encompassing the whole ME. However, the uncertainty in the extent of microscopic disease resulting in an uncertain CTV does not allow achieving an optimal boost volume. This study aimed to quantify the incidence and extent of subclinical disease beyond GTV to be applied for a more confident CTV determination and consequently optimisation of the boost volume. The microscopic disease was found beyond 1 cm in 53% and beyond 2 cm in 19% of cases, respectively. A 19% of microscopic disease was found to be beyond current standard margin (i.e. asymmetric excision margin plus symmetric CTV margin as shown in a).
Figure 1. Schematic diagram of the boost volume in post-lumpectomy external radiotherapy, a) current approach that considers a symmetric volume to the asymmetric excision margin, as compared to b) new proposed approach with asymmetrically defined CTV to the excision cavity, suggested to reduce the boost volume in the current approach (on the left).
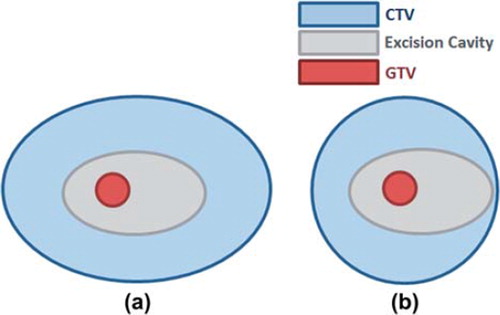
In the second part of the study [Citation62], given the distribution of ME derived in the first stage of study, an alternative asymmetric boost CTV margin, yielding the same probability of ME outside the margin, was defined. In this configuration, as schematically illustrated in b, CTV margin compensates for the asymmetry of excision margin (i.e. CTV is not uniform but the addition of CTV and excision margin is constant, e.g. given that the smallest boost volume has a margin of 15 mm beyond GTV, if the excision margin in one direction is 7 mm, then the CTV margin will be 15–7 = 8 mm). The alternative boost volume was smaller by 27% compared to current boost volume with symmetric margins for CTV without compromising the coverage of microscopic disease.
Other organs
While pathology studies are generally concentrating on the four above-discussed tumour sites which constitute the majority of cases encountered, other organs, though more sparsely, have also been addressed by a number of authors.
Head & Neck.
Radiation therapy has been the common approach in the management of head and neck carcinoma due to the complex anatomy of head and neck which renders many tumours unresectable. The routine clinical use of RT for head and neck tumours has become even more convincing after the development of advanced treatment techniques such as IMRT which offers a better conformity to irregular target volumes. However, the effectiveness of these techniques requires a precisely-delineated target volume which encompasses the whole disease without compromising the risk to critical normal tissue. To our knowledge, there is only one study by Ho et al. [Citation63] examining the microscopic extension in head and neck cancer. The submucosal tumour extensions on resected specimens of 57 patients with hypopharyngeal cancer were evaluated in the superior, medial, lateral and inferior directions. Skip metastases (when submucosal tumour is completely separated from tumour bulk) were observed up to 37 mm from primary for patients who had previously received radiotherapy.
A number of authors conducted studies to determine the optimal CTV margin around gross nodal disease in head and neck cancer [Citation64–66]. At present, there is no consensus for the delineation of nodal CTV margin and it actually ranges from 5–20 mm in different institutions (e.g. CTV is reported 20 mm in the Chao's study [Citation64]). The determination of CTV becomes particularly challenging when the probability of tumour extension outside the nodal capsule, i.e. ECE, is increased. The presence of ECE is troublesome due to the adverse impact of microscopic disease associated with ECE on the incidences of local recurrences [Citation66–69].
Apisarnthanarax et al. [Citation66] conducted a histopathological study on 96 specimens of 48 patients with squamous cell carcinoma of the head and neck to quantify the ECE for potential application in CTV determination. ECE inversely correlated with the distance from the gross tumour and a 10 mm margin was found to be sufficient to encompass microscopic extension of lymph nodes <3 cm to be treated with IMRT. For patients receiving 3DCRT, however, a margin of 13–15 mm was recommended. Unlike the previous studies which reported a positive correlation between the incidence of ECE and nodal size (when the nodal size is less than 1 cm the incidence of ECE ranged 17–40% but when it exceeds 3 cm then incidence is increased to >75%), the data of Apisarnthanarax [Citation66] does not show any correlation between ECE and size of lymph nodes. Nevertheless, since the samples included in their study were quite small (mostly <2 cm), a more generous margin for CTV could be allowed if lymph node size is larger than 3 cm.
Bladder.
The bladder is another pathological site which was investigated in relation to ME. The extension of microscopic disease outside nodal capsule in bladder cancer is defined by the extra-vesical extension (EVE). Jenkins et al. [Citation70] examined 80 cystectomy specimens of patients with bladder cancer to measure the incidence and extent of EVE, whereby to define the appropriate CTV margin. Patients were differentiated based on whether EVE is seen on their CT images (i.e. when there is an irregular interface between a tumour and perivesical fat or an overt solid EVE is seen) or not. A margin of 10 mm and 6 mm beyond the outer wall of bladder was found to be sufficient to encompass 90% of ME in patients with and without EVE on their CT images, respectively. Furthermore, radiological and histopathological data were compared to find a correlation between the extent of histological EVE and radiological information [e.g. tumour size and lymphovascular invasion (LVI)]. LVI and tumour size were found to be associated with more extensive EVE.
Liver.
The liver, among other sites, by having relatively low tolerance to radiation warrants more detailed investigations. Wang et al. [Citation71] examined specimens of 76 patients with hepatocellular cancer to evaluate the presence and extent of microscopic disease and also to correlate these values to pre-treatment factors. ME status (positive or negative) was found to be associated with elevated α-fetoprotein (AFP) levels and tumour grade while the extent of microscopic disease was only related to tumour grade. No correlation between tumour size and ME was observed. The range of microscopic disease and the proposed margins for all three grades of liver cancer are indicated in the Supplementary Appendix Table I to be found online at http://informahealthcare.com/doi/abs/10.3109/0284186X.2012.720381. A previous study of this group on 149 patients’ specimens suggested that a margin of 4 mm will encompass the whole microscopic disease with 100% confidence level [Citation72].
Quantitative evaluation of the extent of subclinical invasion of a tumour into adjacent tissue has been carried out for three other cancer sites, including melanoma of the skin, vulva, and ovarian cancer [Citation73–75]. The latter study, however, was aimed to provide a guideline for the optimal margin beyond visual ovarian tumour to be resected [Citation75].
To our knowledge, histopathological evaluation of ME in other tumour sites such as colon, rectum, uterus, and oral cavity, has not been addressed in the literature. It is worth noting that a number of these tumour sites have high incidence and cancer-related death rates which further justifies ME studies.
Discussion
The pattern of microscopic extension of a tumour is a crucial piece of information when determining CTV for modern radiotherapy techniques which restrict the high dose regions to defined irradiation volumes. Nowadays, advanced treatment techniques such as IMRT and IGRT rely upon molecular imaging modalities such as positron emission tomography (PET) integrated with CT to detect areas with altered functionality on its respective anatomical location depicted by CT. The accuracy of detection of suspicious lesions, however, is constrained by the resolution of PET/CT scanners. In other words, subclinical disease to be incorporated in CTV cannot be detected by imaging modalities developed to date. In addition to imaging uncertainty, clinician variability in CTV definition is also considerable. This issue was explicitly illustrated in the results of a head and neck IMRT survey conducted by Hong et al. [Citation76] where the need for consensus guidelines for effective implementation of modern treatment techniques was confirmed. In this article we reviewed studies concerning the pathological assessment of the incidence and extension of microscopic disease beyond the visible part of tumours and also identifying possible correlations between pre-treatment factors and the extent of ME.
Despite the fact that the issue of CTV uncertainty is probably one of the reasons for treatment failure even after technological advances in RT, the number of studies which address the ME is either very limited or inexistent for many cancer sites. To our knowledge there is only one study investigating tumour microscopic extension for several tumour sites: ovarian cancer [Citation75], bladder [Citation70], skin [Citation74], head and neck, and vulva [Citation73]. While it seems there are more than one study related to ME in head and neck, there is actually one study focusing on the submucosal extension [Citation63] and another on ECE outside nodal capsule [Citation66] which are clearly not addressing the same condition. More investigations regarding tumour subclinical spread of these sites are required to confirm the results of above-mentioned studies. Also several cancer sites, such as colon and rectum, have not been addressed in any clinical studies so far, thus the tumour microscopic extension for these sites is currently in the dark.
Furthermore, as shown in the Supplementary Appendix Table I to be found online at http://informahealthcare.com/doi/abs/10.3109/0284186X.2012.720381, discrepancies can be seen between the outcomes of different studies for the same organ (e.g. brain) in contrast to other organs showing a better consistency in proposed margins (e.g. lung and prostate). The evaluations of the extent of infiltration beyond macroscopic tumour for NSCLC by different studies have led to fairly conclusive results for ADC and SCC histologic types [Citation4,Citation8,Citation9] with the study of Grills et al. [Citation9] further stratifying ADC patients and proposing different margins for grades 1, 2, and 3 ADC, NSCLC. However, evaluations of bronchial extension of lung cancer have not reached the consensus (1.9 cm margin proposed by Cotton et al. [Citation13] vs. 1.5 cm margin proposed by Kara et al. [Citation14]). Also the extent of microscopic nodal ECE (addressed by only one study [Citation11]) and microscopic extension in SCLC (no data available) remains uncertain. In the light of information acquired for ECE of prostate cancer being investigated by plenty of studies which have resulted in quite consistent proposed CTV margins for favourable and unfavourable groups (refer to Supplementary Appendix Table I to be found online at http://informahealthcare.com/doi/abs/10.3109/0284186X.2012.720381), a conclusive decision on the extent of CTV margin can be made.
For breast cancer, the data does not seem to be conclusive since the studies have been done for different scenarios (e.g. different selection criteria for PBI in current studies, as compared to those applied in the 1980s [Citation45], results in a group of samples with smaller tumour sizes which will affect the pattern of subclinical disease distribution). While the results of two studies [Citation53,Citation54] on the evaluation of ductal carcinoma extension and its correlation with different determinant factors (e.g. age and tumour size) are in approximate agreement, they do not correspond to those acquired by Vicini et al. [Citation59] in terms of median value and range of microscopic extension. On top of that, more recent studies [Citation46,Citation62] argue the effectiveness of symmetric CTV and believe that since the excision margin is not typically uniform, the optimal CTV must be defined asymmetric such that the addition of CTV and excision margin remain constant in all directions.
Regarding brain cancer, a significant contradiction was revealed between expert opinions. With reference to CTV margin definition for GBM, the study of Matsuo et al. [Citation31] concluded that a margin of 2 cm to the enhanced area on T2-MRI (i.e. edema + 2 cm) is required to achieve tumour control. On the other hand, in a related work by Minniti et al. [Citation33] it was demonstrated that 2 cm margin to T2-MRI leads to late radiation-induced toxicity without bringing about improved recurrence patterns as compared to plans with smaller margins where CTV includes residual tumour and resection cavity plus 2 cm. The controversy persists for delineation of CTV for brain metastasis treated with SRS. The study of Noel et al. [Citation38] showed that adding a margin of 1 mm to GTV would increase tumour local control significantly. This result was supported by the study of Baumert et al. [Citation42] who stratified brain metastasis in terms of their histological origin and came to the same conclusion that 1 mm margin outside GTV will suffice proper coverage of tumour. In contrast, the study of Soltys et al. [Citation40] demonstrated that SRS should target the tumour bed and 2 mm beyond. This idea was supported by the analysis performed by Choi et al. [Citation41], while it was strongly disagreed by Nataf et al. [Citation39] whose study indicated that addition of 2 mm margin would result in severe parenchymal toxicity without offering a better tumour control. Another study [Citation43] showed that even the addition of 5 mm did not improve tumour control and WBI rectified the low TCP.
In summary, we conclude that further histopathological studies are required to reach a consensus for each individual organ site regarding CTV, particularly for those sites with inexistent data as well as those showing discrepancies among different studies (such as brain and breast). Additionally, these data could serve as valuable tools in the development and validation of bio-mathematical models. These models, based on a sequence of observations or development of a hypothesis can assume various links between biological mechanisms involved in cancer development and progression to provide quantitative or qualitative measures of tumour behaviour as well as tumour response to treatment. The determinant parameters correlated with the extension of microscopic disease could be considered as model parameters. Alternatively sensitivity studies can be performed by developing a model of a specific tumour and using these data to predict TCPs and NTCPs associated with current and proposed CTV margins. The outcome of these models could serve as guidelines for clinicians to define optimal CTVs.
Supplementary Appendix Table I
Download PDF (126.3 KB)Declaration of interest: The authors report no conflicts of interest. The authors alone are responsible for the content and writing of the paper.
References
- International commission of radiation units and measurements. Prescribing, Recording, and Reporting Photon Beam Therapy, Report no. 50, ICRU: Bethesda;1993.
- Siegel R, Naishadham D, Jemal A. Cancer statistics, 2012.CA Cancer J Clin 2012;62:10–29.
- Jemal A, Siegel R, Ward E, Hao Y, Xu J, Thun MJ. Cancer statistics 2009.CA Cancer J Clin 2009;59: 225–49.
- Giraud P, Antoine M, Larrouy A, Milleron B, Callard P, De Rycke Y, . Evaluation of microscopic tumor extension in non-small-cell lung cancer for three-dimensional conformal radiotherapy planning. Int J Radiat Oncol Biol Phys 2000;48:1015–24.
- Graham MV, Matthews JW, Harms Sr WB, Emami B, Glazer HS, Purdy JA. Three-dimensional radiation treatment planning study for patients with carcinoma of the lung. Int J Radiat Oncol Biol Phys 1994;29:1105–17.
- Graham MV, Purdy JA, Emami B, Matthews JW, Harms WB. Preliminary results of a prospective trial using three dimensional radiotherapy for lung cancer. Int J Radiat Oncol Biol Phys 1995;33:993–1000.
- Ekberg L, Holmberg O, Wittgren L, Bjelkengren G, Landberg T. What margins should be added to the clinical target volume in radiotherapy treatment planning for lung cancer?Radiother Oncol 1998;48:71–7.
- Li WL, Yu JM, Liu GH, Zhong WX, Li WW, Zhang BJ. [A comparative study on radiology and pathology target volume in non-small-cell lung cancer]. Zhonghua Zhong Liu Za Zhi 2003;25:566–8.
- Grills IS, Fitch DL, Goldstein NS, Yan D, Chmielewski GW, Welsh RJ, . Clinicopathologic analysis of microscopic extension in lung adenocarcinoma: defining clinical target volume for radiotherapy. Int J Radiat Oncol Biol Phys 2007;69:334–41.
- Travis WD, Brambilla E, Muller-Hermelink HK, Harris C. Pathology and genetics of tumours of the lung, pleura, thymus and heart. Lyon, France: IARC Press; 2004.
- Yuan S, Meng X, Yu J, Mu D, Chao KS, Zhang J, . Determining optimal clinical target volume margins on the basis of microscopic extracapsular extension of metastatic nodes in patients with non-small-cell lung cancer. Int J Radiat Oncol Biol Phys 2007;67:727–34.
- Dong MJ, Liu ZF, Zhao K, Ruan LX, Wang GL, Yang SY, . Value of 18F-FDG-PET/PET-CT in differentiated thyroid carcinoma with radioiodine-negative whole-body scan: A meta-analysis. Nucl Med Commun 2009;30:639–50.
- Cotton RE. The bronchial spread of lung cancer. Br J Disease Chest 1959;53:142–50.
- Kara M, Sak SD, Orhan D, Yavuzer S. Changing patterns of lung cancer; (3/4 in.) 1.9 cm; still a safe length for bronchial resection margin?Lung Cancer 2000;30:161–8.
- Partin AW, Kattan MW, Subong EN, Walsh PC, Wojno KJ, Oesterling JE, . Combination of prostate-specific antigen, clinical stage, and Gleason score to predict pathological stage of localized prostate cancer. A multi-institutional update. JAMA 1997;277:1445–51.
- Pisansky TM, Davis BJ. Predictive factors in localized prostate cancer: Implications for radiotherapy and clinical trial design. Semin Urol Oncol 2000;18:93–107.
- Chao KK, Goldstein NS, Yan D, Vargas CE, Ghilezan MI, Korman HJ, . Clinicopathologic analysis of extracapsular extension in prostate cancer: Should the clinical target volume be expanded posterolaterally to account for microscopic extension?Int J Radiat Oncol Biol Phys 2006;65: 999–1007.
- Stamey TA, McNeal JE, Freiha FS, Redwine E. Morphometric and clinical studies on 68 consecutive radical prostatectomies. J Urol 1988;139:1235–41.
- Epstein JI, Carmichael MJ, Pizov G, Walsh PC. Influence of capsular penetration on progression following radical prostatectomy: A study of 196 cases with long-term followup. J Urol 1993;150:135–41.
- Davis BJ, Pisansky TM, Wilson TM, Rothenberg HJ, Pacelli A, Hillman DW, . The radial distance of extraprostatic extension of prostate carcinoma: Implications for prostate brachytherapy. Cancer 1999;85:2630–7.
- Sohayda C, Kupelian PA, Levin HS, Klein EA. Extent of extracapsular extension in localized prostate cancer. Urology 2000;55:382–6.
- Teh BS, Bastasch MD, Mai WY, Butler EB, Wheeler TM. Predictors of extracapsular extension and its radial distance in prostate cancer: Implications for prostate IMRT, brachytherapy, and surgery. Cancer J 2003;9:454–60.
- Teh BS, Bastasch MD, Wheeler TM, Mai WY, Frolov A, Uhl BM, . IMRT for prostate cancer: Defining target volume based on correlated pathologic volume of disease. Int J Radiat Oncol Biol Phys 2003;56:184–91.
- Schwartz DJ, Sengupta S, Hillman DW, Sargent DJ, Cheville JC, Wilson TM, . Prediction of radial distance of extraprostatic extension from pretherapy factors. Int J Radiat Oncol Biol Phys 2007;69:411–8.
- Stupp R, Hegi ME, Mason WP, van den Bent MJ, Taphoorn MJ, Janzer RC, . Effects of radiotherapy with concomitant and adjuvant temozolomide versus radiotherapy alone on survival in glioblastoma in a randomised phase III study: 5-year analysis of the EORTC-NCIC trial. Lancet Oncol 2009;10:459–66.
- Jansen EP, Dewit LG, van Herk M, Bartelink H. Target volumes in radiotherapy for high-grade malignant glioma of the brain. Radiother Oncol 2000;56:151–6.
- Seither RB, Jose B, Paris KJ, Lindberg RD, Spanos WJ. Results of irradiation in patients with high-grade gliomas evaluated by magnetic resonance imaging. Am J Clin Oncol 1995;18:297–9.
- Nelson DF, Curran Jr WJ, Scott C, Nelson JS, Weinstein AS, Ahmad K, . Hyperfractionated radiation therapy and bis-chlorethyl nitrosourea in the treatment of malignant glioma—Possible advantage observed at 72.0 Gy in 1.2 Gy B.I.D. fractions: Report of the radiation therapy oncology group protocol 8302. Int J Radiat Oncol Biol Phys 1993; 25:193–207.
- Urtasun RC, Kinsella TJ, Farnan N, DelRowe JD, Lester SG, Fulton DS. Survival improvement in anaplastic astrocytoma, combining external radiation with halogenated pyrimidines: Final report of RTOG 86-12, phase I–II study. Int J Radiat Oncol Biol Phys 1996;36:1163–7.
- Chang EL, Akyurek S, Avalos T, Rebueno N, Spicer C, Garcia J, . Evaluation of peritumoral edema in the delineation of radiotherapy clinical target volumes for glioblastoma. Int J Radiat Oncol Biol Phys 2007;68: 144–50.
- Matsuo M, Miwa K, Tanaka O, Shinoda J, Nishibori H, Tsuge Y, . Impact of [(11)C]methionine positron emission tomography for target definition of glioblastoma multiforme in radiation therapy planning. Int J Radiat Oncol Biol Phys 2012;82:83–90.
- Ogawa T, Shishido F, Kanno I, Inugami A, Fujita H, Murakami M, . Cerebral glioma: Evaluation with methionine PET. Radiology 1993;186:45–53.
- Minniti G, Amelio D, Amichetti M, Salvati M, Muni R, Bozzao A, . Patterns of failure and comparison of different target volume delineations in patients with glioblastoma treated with conformal radiotherapy plus concomitant and adjuvant temozolomide. Radiother Oncol 2010; 97:377–81.
- Engenhart R, Kimmig BN, Hover KH, Wowra B, Romahn J, Lorenz WJ, . Long-term follow-up for brain metastases treated by percutaneous stereotactic single high-dose irradiation. Cancer 1993;71:1353–61.
- Pirzkall A, Debus J, Lohr F, Fuss M, Rhein B, Engenhart-Cabillic R, . Radiosurgery alone or in combination with whole-brain radiotherapy for brain metastases. J Clin Oncol 1998;16:3563–9.
- Breneman JC, Warnick RE, Albright RE, Kukiatinant N, Shaw J, Armin D, . Stereotactic radiosurgery for the treatment of brain metastases. Cancer 1997;79:551–7.
- Aoyama H, Shirato H, Tago M, Nakagawa K, Toyoda T, Hatano K, . Stereotactic radiosurgery plus whole-brain radiation therapy vs stereotactic radiosurgery alone for treatment of brain metastases: A randomized controlled trial. JAMA 2006;295:2483–91.
- Noel G, Simon JM, Valery CA, Cornu P, Boisserie G, Hasboun D, . Radiosurgery for brain metastasis: Impact of CTV on local control. Radiother Oncol 2003;68:15–21.
- Nataf F, Schlienger M, Liu Z, Foulquier JN, Gres B, Orthuon A, . Radiosurgery with or without a 2-mm margin for 93 single brain metastases. Int J Radiat Oncol Biol Phys 2008;70:766–72.
- Soltys SG, Adler JR, Lipani JD, Jackson PS, Choi CY, Puataweepong P, . Stereotactic radiosurgery of the postoperative resection cavity for brain metastases. Int J Radiat Oncol Biol Phys 2008;70:187–93.
- Choi CY, Chang SD, Gibbs IC, Adler JR, Harsh GRt, Lieberson RE, . Stereotactic radiosurgery of the postoperative resection cavity for brain metastases: Prospective evaluation of target margin on tumor control. Int J Radiat Oncol Biol Phys Epub2012 May 30.
- Baumert BG, Rutten I, Dehing-Oberije C, Twijnstra A, Dirx MJ, Debougnoux-Huppertz RM, . A pathology-based substrate for target definition in radiosurgery of brain metastases. Int J Radiat Oncol Biol Phys 2006;66:187–94.
- Yoo H, Kim YZ, Nam BH, Shin SH, Yang HS, Lee JS, . Reduced local recurrence of a single brain metastasis through microscopic total resection. J Neurosurg 2009;110:730–6.
- Raore B, Schniederjan M, Prabhu R, Brat DJ, Shu HK, Olson JJ. Metastasis infiltration: An investigation of the postoperative brain-tumor interface. Int J Radiat Oncol Biol Phys 2010;81:1075–80.
- Holland R, Veling SHJ, Mravunac M, Hendriks JHCL. Histologic multifocality of tis, T1–2 breast carcinomas implications for clinical trials of breast-conserving surgery. Cancer 1985;56:979–90.
- Kirby AM, Coles CE, Yarnold JR. Target volume definition for external beam partial breast radiotherapy: Clinical, pathological and technical studies informing current approaches. Radiother Oncol 2010;94:255–63.
- Recht A, Connolly JL, Schnitt SJ, Silver B, Rose MA, Love S, . The effect of young age on tumor recurrence in the treated breast after conservative surgery and radiotherapy. Int J Radiat Oncol Biol Phys 1988;14:3–10.
- Healey EA, Osteen RT, Schnitt SJ, Gelman R, Stomper PC, Connolly JL, . Can the clinical and mammographic findings at presentation predict the presence of an extensive intraductal component in early stage breast cancer?Int J Radiat Oncol Biol Phys 1989;17:1217–21.
- Eberlein TJ, Connolly JL, Schnitt SJ, Recht A, Osteen RT, Harris JR. Predictors of local recurrence following conservative breast surgery and radiation therapy. The influence of tumor size. Arch Surg 1990;125:771–5; discussion 775–7.
- Vicini FA, Eberlein TJ, Connolly JL, Recht A, Abner A, Schnitt SJ, . The optimal extent of resection for patients with stages I or II breast cancer treated with conservative surgery and radiotherapy. Ann Surg 1991;214:200–4; discussion 204–5.
- Holland R, Connolly JL, Gelman R, Mravunac M, Hendriks JH, Verbeek AL, . The presence of an extensive intraductal component following a limited excision correlates with prominent residual disease in the remainder of the breast. J Clin Oncol 1990;8:113–8.
- Holland R, Hendriks JH, Vebeek AL, Mravunac M, Schuurmans Stekhoven JH .Extent, distribution, and mammographic/histological correlations of breast ductal carcinoma in situ. Lancet 1990;335:519–22.
- Ohtake T, Abe R, Kimijima I, Fukushima T, Tsuchiya A, Hoshi K, . Intraductal extension of primary invasive breast carcinoma treated by breast-conservative surgery. Computer graphic three-dimensional reconstruction of the mammary duct-lobular systems. Cancer 1995;76: 32–45.
- Imamura H, Haga S, Shimizu T, Watanabe O, Kinoshita J, Nagumo H, . Relationship between the morphological and biological characteristics of intraductal components accompanying invasive ductal breast carcinoma and patient age. Breast Cancer Res Treat 2000;62:177–84.
- Swanson TA, Vicini FA. Overview of accelerated partial breast irradiation. Curr Oncol Rep 2008;10:54–60.
- Boyages J, Recht A, Connolly JL, Schnitt SJ, Gelman R, Kooy H, . Early breast cancer: Predictors of breast recurrence for patients treated with conservative surgery and radiation therapy. Radiother Oncol 1990;19:29–41.
- Vicini FA, Baglan KL, Kestin LL, Mitchell C, Chen PY, Frazier RC, . Accelerated treatment of breast cancer. J Clin Oncol 2001;19:1993–2001.
- Vicini FA, Kestin L, Chen P, Benitez P, Goldstein NS, Martinez A. Limited-field radiation therapy in the management of early-stage breast cancer. J Natl Cancer Inst 2003;95:1205–10.
- Vicini FA, Kestin LL, Goldstein NS. Defining the clinical target volume for patients with early-stage breast cancer treated with lumpectomy and accelerated partial breast irradiation: A pathologic analysis. Int J Radiat Oncol Biol Phys 2004;60:722–30.
- Kirby AM, Evans PM, Nerurkar AY, Desai SS, Krupa J, Devalia H, . How does knowledge of three-dimensional excision margins following breast conservation surgery impact upon clinical target volume definition for partial-breast radiotherapy?Radiother Oncol 2010;94:292–9.
- Hanbeukers B, Borger J, van den Ende P, van der Ent F, Houben R, Jager J, . Customized computed tomography-based boost volumes in breast-conserving therapy: Use of three-dimensional histologic information for clinical target volume margins. Int J Radiat Oncol Biol Phys 2009; 75:757–63.
- Stroom J, Schlief A, Alderliesten T, Peterse H, Bartelink H, Gilhuijs K. Using histopathology breast cancer data to reduce clinical target volume margins at radiotherapy. Int J Radiat Oncol Biol Phys 2009;74:898–905.
- Ho CM, Ng WF, Lam KH, Wei WJ, Yuen AP. Submucosal tumor extension in hypopharyngeal cancer. Arch Otolaryngol Head Neck Surg 1997;123:959–65.
- Chao KS, Wippold FJ, Ozyigit G, Tran BN, Dempsey JF. Determination and delineation of nodal target volumes for head-and-neck cancer based on patterns of failure in patients receiving definitive and postoperative IMRT. Int J Radiat Oncol Biol Phys 2002;53:1174–84.
- Gregoire V, Levendag P, Ang KK, Bernier J, Braaksma M, Budach V, . CT-based delineation of lymph node levels and related CTVs in the node-negative neck: DAHANCA, EORTC, GORTEC, NCIC, RTOG consensus guidelines. Radiother Oncol 2003;69:227–36.
- Apisarnthanarax S, Elliott DD, El-Naggar AK, Asper JA, Blanco A, Ang KK, . Determining optimal clinical target volume margins in head-and-neck cancer based on microscopic extracapsular extension of metastatic neck nodes. Int J Radiat Oncol Biol Phys 2006;64:678–83.
- Johnson JT, Barnes EL, Myers EN, Schramm VL, Jr., Borochovitz D, Sigler BA. The extracapsular spread of tumors in cervical node metastasis. Arch Otolaryngol 1981;107:725–9.
- Johnson JT, Myers EN, Bedetti CD, Barnes EL, Schramm VL, Jr., Thearle PB. Cervical lymph node metastases. Incidence and implications of extracapsular carcinoma. Arch Otolaryngol 1985;111:534–7.
- Snyderman NL, Johnson JT, Schramm VL, Jr., Myers EN, Bedetti CD, Thearle P. Extracapsular spread of carcinoma in cervical lymph nodes. Impact upon survival in patients with carcinoma of the supraglottic larynx. Cancer 1985;56:1597–9.
- Jenkins P, Anjarwalla S, Gilbert H, Kinder R. Defining the clinical target volume for bladder cancer radiotherapy treatment planning. Int J Radiat Oncol Biol Phys 2009;75: 1379–84.
- Wang W, Feng X, Zhang T, Jin J, Wang S, Liu Y, . Prospective evaluation of microscopic extension using whole-mount preparation in patients with hepatocellular carcinoma: Definition of clinical target volume for radiotherapy. Radiat Oncol 2010;5:73.
- Wang MH, Ji Y, Zeng ZC, Tang ZY, Fan J, Zhou J, . Impact factors for microinvasion in patients with hepatocellular carcinoma: Possible application to the definition of clinical tumor volume. Int J Radiat Oncol Biol Phys 2010;76:467–76.
- Hoffman MS, Gunesakaran S, Arango H, DeCesare S, Fiorica JV, Parsons M, . Lateral microscopic extension of squamous cell carcinoma of the vulva. Gynecol Oncol 1999;73:72–5.
- Choo R, Woo T, Assaad D, Antonyshyn O, Barnes EA, McKenzie D, . What is the microscopic tumor extent beyond clinically delineated gross tumor boundary in nonmelanoma skin cancers?Int J Radiat Oncol Biol Phys 2005;62:1096–9.
- Fennimore IA, Simon NL, Bills G, Dryfhout VL, Schniederjan AM. Extension of ovarian tissue into the infundibulopelvic ligament beyond visual margins. Gynecol Oncol 2009;114:61–3.
- Hong TS, WA. Tome, Harari PM. Heterogeneity in head and neck IMRT target design and clinical practice. Radiother Oncol 2012;103:92–8.