Abstract
Background. In lung cancer radiotherapy, planning on the midventilation (MidV) bin of a four-dimensional (4D) CT scan can reduce the systematic errors introduced by respiratory tumour motion compared to conventional CT. In this study four different methods for MidV bin selection are evaluated. Material and methods. The study is based on 4DCT scans of 19 patients with a total of 23 peripheral lung tumours having peak-to-peak displacement ≥ 5 mm in at least one of the left-right (LR), anterior-posterior (AP) or cranio-caudal (CC) directions. For each tumour, the MidV bin was selected based on: 1) visual evaluation of tumour displacement; 2) rigid registration of tumour position; 3) diaphragm displacement in the CC direction; and 4) carina displacement in the CC direction. Determination of the MidV bin based on the displacement of the manually delineated gross tumour volume (GTV) was used as a reference method. The accuracy of each method was evaluated by the distance between GTV position in the selected MidV bin and the time-weighted mean position of GTV throughout the bins (i.e. the geometric MidV error). Results. Median (range) geometric MidV error was 1.4 (0.4–5.4) mm, 1.4 (0.4–5.4) mm, 1.9 (0.5–6.9) mm, 2.0 (0.5–12.3) mm and 1.1 (0.4–5.4) mm for the visual, rigid registration, diaphragm, carina, and reference method. Median (range) absolute difference between geometric MidV error for the evaluated methods and the reference method was 0.0 (0.0–1.2) mm, 0.0 (0.0–1.7) mm, 0.7 (0.0–3.9) mm and 1.0 (0.0–6.9) mm for the visual, rigid registration, diaphragm and carina method. Conclusion. The visual and semi-automatic rigid registration methods were equivalent in accuracy for selecting the MidV bin of a 4DCT scan. The methods based on diaphragm and carina displacement cannot be recommended without modifications.
In radiotherapy planning of lung cancer, respiratory tumour motion during conventional computed tomography (CT) acquisition can cause image artefacts in tumour shape as well as an arbitrary representation of tumour position, thereby leading to a risk of systematic errors in the radiation treatment [Citation1,Citation2]. Four-dimensional (4D) CT has the potential to reduce motion artefacts compared to conventional CT [Citation3–7], even though motion artefacts can still be present [Citation8–10]. In a 4DCT scan the images are over-sampled and divided into a number of bins, each related to a different phase of the respiration cycle. The midventilation (MidV) bin of a 4DCT scan is the bin best representing the time-weighted mean position of the tumour over the respiration cycle. By using the MidV bin for radiotherapy planning, the risk of introducing systematic errors can be reduced compared to planning on a conventional CT scan [Citation11].
So far no commercial treatment planning systems provides the ability to select the MidV bin of a 4DCT scan. Therefore, in the implementation process of MidV-based treatment planning, radiotherapy clinics have to develop a method themselves.
In this study, four different methods to select the MidV bin were compared to a reference method based on the displacement of a manually delineated gross tumour volume (GTV). The methods were based on: 1) Visual evaluation of tumour displacement; 2) Rigid registration of tumour position; 3) Diaphragm displacement in the cranio-caudal (CC) direction; and 4) Carina displacement in the CC direction.
Material and methods
Patient selection
At the Department of Radiation Oncology at Rigshospitalet, Denmark, we started using 4DCT scans for planning of lung cancer radiotherapy in February 2009. In our clinical protocol all patients referred for curative radiotherapy of locally advanced non-small cell lung cancer or stereotactic radiotherapy for early stage non-small cell lung cancer are subject to a PET/CT and a 4DCT scan on two consecutive days. Contrast enhancement is used for the PET/CT scan. Based on the 4DCT scan, tumour displacement is visually evaluated in the left-right (LR), anterior-posterior (AP) and CC directions. If peak-to-peak tumour displacement is ≥ 5 mm in at least one of the directions, the MidV bin of the 4DCT scan is used for treatment planning. Otherwise the CT part (conventional free breathing CT) of the PET/CT scan is used for treatment planning. The distinction of 5 mm is based on the recommendations of the AAPM Task Group 76, stating that the extra effort of motion management techniques is unwarranted if peak-to-peak tumour displacement is < 5 mm, unless significant normal tissue sparing is gained [Citation12].
This retrospective study was based on patients with early stage or locally advanced non-small cell lung cancer, subject to the inclusion criteria described below. All patients were referred for radiotherapy between March 2009 and June 2010 at Rigshospitalet, Denmark. Only patients with a peak-to-peak tumour displacement ≥ 5 mm in at least one direction, measured on the 4DCT scans, were included in the study. Furthermore, only patients with peripheral tumours separable from mediastinal tumour mass, and without atelectasis, were included. Nineteen patients (with a total of 23 peripheral tumours) were eligible for the study. Patient characteristics are summarised in .
Table I. Patient characteristics.
4DCT scanning and reconstruction
A Somatom Sensation Open (Siemens Medical Solutions, Erlangen, Germany) CT scanner was used for 4DCT acquisition with the following image acquisition parameters: tube voltage 120 kV, rotation time 1 s, pitch 0.1 and total scan time 99 s. The current-time product was 600 or 800 mAs depending on the size of the patient. The patients were positioned in a VacFix immobilisation device with both arms above their head. The Real-time Position Management™ (RPM) system (Varian Medical Systems, Palo Alto, CA, USA) was used to generate an external respiratory signal, with the RPM marker block placed on the upper abdomen of the patients. The patients were scanned during free breathing and without use of abdominal compression.
For each patient, the raw scan data was retrospectively sorted into 10 bins based on the phase of the respiratory signal. The bins were equally distributed in time over each respiratory cycle. The reconstructed slice thickness was 2 mm.
Method 1: Visual evaluation
For all tumours, selection of the MidV bin by visual evaluation was done in Eclipse™ version 8.6 (Varian Medical Systems) by the same physicist. The bins representing the end-inspiration and end- expiration tumour position were identified visually. The MidV bin was selected as the bin where the tumour by visual inspection was assessed to be in a mean position between its end-inspiration and end-expiration position, taking into consideration visual assessment of both the distribution of bins between inspiration and expiration as well as hysteresis.
Method 2: Rigid registration
The software used for rigid registration of tumour position was developed in MatLab® (The MathWorks, Inc., Natick, MA, USA). For the bin representing the end-inspiration phase, a region of interest (ROI) encompassing the tumour was manually defined, and based on the gradient of the Hounsfield Units (HU) of the voxels within the ROI, an automatically registered tumour volume (ATV) was found. The ATV was automatically defined in the remaining nine bins of the 4DCT by an automatic rigid registration algorithm. The ATV registrations were visually validated. The position of each ATV was represented by its centre of volume (CoV), and the MidV bin was identified as the bin where the CoV of the ATV was closest to its time-weighted mean position.
Method 3: Diaphragm displacement
For each bin, the position of the diaphragmatic dome ipsilateral to the tumour was registered as the CC-coordinate of the first transversal plane, in the CC direction, where the diaphragm was visible. All registrations were done manually by the same clinical oncologist. The MidV bin was identified as the bin where the diaphragmatic dome was closest to its time-weighted mean position.
In case the diaghragmatic dome had the same distance to its time-weighted mean position for several bins, the following selection rules were applied: The 10 bins of each 4DCT scan were listed consecutively, with bin number one corresponding to the end-inspiration. If the diaphragmatic dome had the same distance to its time-weighted mean position for two consecutive bins, the bin with the lowest number was chosen as the MidV bin. In case of more than two consecutive bins, the one in the middle was chosen as MidV bin. In case of two non-consecutive bins, the bin with the lowest number was chosen as the MidV bin.
Method 4: Carina displacement
For each bin, the position of the carina in each bin of the 4DCT was registered as the CC- coordinate of the first transversal plane, in the CC direction, where the septum of the carina divided the trachea into the left and right main bronchus. All registrations were done manually by the same clinical oncologist. The MidV bin was identified as the bin where the carina was closest to its time-weighted mean position.
In case the carina had the same distance to its time-weighted mean position for several bins, the selection criteria were the same as for the diaphragm method.
Reference method
For all tumours, the GTV was manually delineated in Eclipse™ by the same clinical oncologist, using a fixed broad lung window (–1000 HU to 700 HU). The delineation of all bins for each tumour was done in a single procedure to minimise inconsistency. The reference MidV bin was identified as the bin where the CoV of the GTV was closest to its time-weighted mean position.
Accuracy in selecting the MidV bin
The accuracy of each investigated method to select the MidV bin was evaluated by the 3D distance between the CoV of the GTV in the MidV bin selected by the specific method and the time-weighted mean position of the CoV of GTV for the individual 4DCT bins in the reference method. This distance will be referred to as the geometric MidV error.
Statistics
Non-parametric statistics were used as data were not Gaussian distributed. Spearman's correlation tests, with correlation coefficient rS and probability value pS, and Wilcoxon signed rank tests, with probability value pW, were performed in SPSS Statistics 15.0 (IBM, SPSS Inc., Chicago, IL, USA). A probability level (two-tailed) of < 0.05 was considered statistically significant.
Results
Of the 23 tumours, the visual and the rigid registration method selected the same MidV bin as the reference method for 14 and 16 tumours, respectively. For the methods based on diaphragm and carina displacement, this was true for six and three tumours, respectively.
The geometric MidV errors for the evaluated methods versus the reference method are shown in . The median (range) values for the geometric MidV error are listed in . Furthermore, lists the median (range) of the absolute differences between geometric MidV error for the evaluated methods and the reference method.
Figure 1. Geometric MidV errors for the evaluated methods versus the reference method. The lines illustrate where the geometric MidV error for the evaluated methods equals that of the reference method.
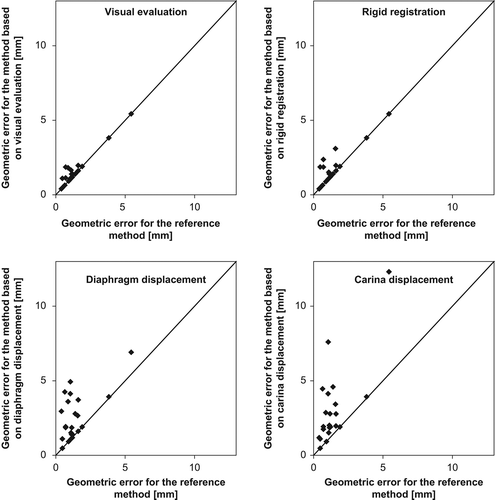
Table II. Median (range) of the geometric MidV errors for the evaluated methods and the reference method. Median (range) of the absolute differences between the geometric MidV errors for the evaluated methods and the reference method.
For one patient (with one tumour) it was not possible to select a MidV bin based on carina displacement, since the carina had the same distance to its time-weighted mean position for all 10 bins.
The geometric MidV error for the reference method was > 0 for all the tumours, i.e. the CoV of the GTV for the reference MidV bin and the time-weighted mean position of the CoV of the GTV for the individual 4DCT bins were not coincident. This was due to hysteresis and the limited time resolution of the 10 bins for each 4DCT scan. By definition, in this study the geometric MidV error of the reference method was the smallest possible geometric MidV error. The median (range) largest possible geometric MidV error (i.e. the largest possible distance between the CoV of the GTV in any of the 10 bins and the time-weighted mean position of the CoV of the GTV thoughout the bins) was 6.1 (3.0–22.0) mm.
For two patients the reference geometric MidV errors were as much as 3.8 mm and 5.4 mm (see ).
The geometric MidV errors for both the visual and the rigid registration method were more than twice that of the reference method for three tumours each. The same was true for nine and 13 tumours for the methods based on diaphragm and carina displacement, respectively.
The geometric MidV errors for the visual method versus the rigid registration method are shown in .
The median geometric MidV errors for both the visual and the rigid registration method were significantly smaller than for the methods based on diaphragm and carina displacement (pW < 0.020). The difference between the median geometric MidV error for the visual and the rigid registration method was not significant, just as there was no significant difference between median geometric MidV error for the methods based on diaphragm and carina displacement.
The peak-to-peak displacements of the ATVs (from method 2) and GTVs in the LR, AP and CC directions are shown in . The median (range) values are listed in .
Figure 3. Peak-to-peak displacements of the ATV and GTV in the LR, AP and CC directions. AP, anterior-posterior; ATV, automatically registered tumour volume, CC, cranio-caudal; GTV, gross tumour volume; LR, left-right.
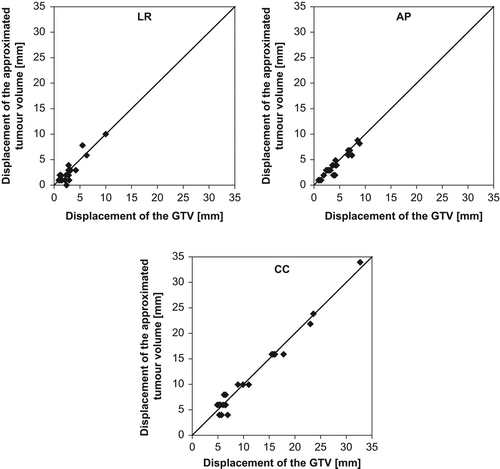
Table III. Median (range) peak-to-peak displacements of ATV and GTV in the LR, AP and CC directions.
The peak-to-peak displacements of the ATVs and the GTVs were significantly correlated in all three directions (LR: pS < 0.001, rS = 0.662, AP: pS < 0.001, rS = 0.885, CC: pS < 0.001, rS = 0.883).
Two patients had two tumours each and one patient had three tumours. For one of the patients with two tumours, the visual and the rigid registration method selected the same MidV bin as the reference method regardless of the tumour evaluated. For the other two patients with multiple tumours, the MidV bins selected by the visual, rigid registration, and reference methods were highly dependent on the tumour evaluated.
Excluding all three patients with multiple tumours from the analyses had only minor impact on the results.
Discussion
The accuracy of the visual and the rigid registration methods were generally equally good, and for more than half of the evaluated tumours, these two methods selected the same MidV bin as the reference method. No overall difference in accuracy was found between the visual and the rigid registration method. Therefore, the clinical consequences of choosing one method over the other would be minor. The accuracy of both the diaphragm and the carina methods were generally inferior to the visual and the rigid registration methods. The diaphragm and the carina methods selected the same MidV bin as the reference method in significantly fewer cases than the visual and the rigid registration methods, and the absolute differences to the reference geometric MidV error were as large as 4–7 mm.
The reference geometric MidV error was notably high for two tumours (3.8 mm and 5.4 mm). This was due to large tumour displacement in the CC direction as well as a motion pattern affected by hysteresis.
Selection of a specific phase bin from a 4DCT as representation of the midposition of the tumour is related to an inherent geometric MidV error because of hysteresis of the tumour trajectory (e.g. no points on a circle coincide with the midposition) and due to the limited number of bins, which defines the time resolution. However, the clinical importance of this inherent geometric MidV error is normally small compared to other uncertainties impacting the process of planning and delivery of radiotherapy, e.g. delineation errors [Citation13], image artefacts [Citation8,Citation14], positioning [Citation15], etc.
Of the four evaluated methods, the visual method would be the simplest to implement in a clinical setting, since it only requires a treatment planning system able to handle a 4DCT data set and does not acquire access to inhouse developed software for 4D image processing. For all tumours in this study the visual method was performed by the same person, thus the results do not reflect possible inter-observer variability in a clinical setting. Furthermore, the method was performed using the same specific procedure for all the tumours, as described earlier. Thus, the accuracy of the visual method is possibly overestimated and this may hamper the translation of our results to the clinical situation.
Contrary to the visual method, the rigid registration method requires development of dedicated software for implementation in a clinical setting. However, the rigid registration method could easily be implemented in commercial treatment planning systems. Furthermore, the inter-observer variability of the definition of the volume of interest is presumably providing a smaller impact on the rigid registration method compared to the inter-observer variability of the visual method. The accuracy of the rigid registration method relies on the ability of estimating respiratory tumour motion by rigid registration of the ATV. For a few tumours the deviation between the displacement of the ATV and GTV was considerable compared to the GTV displacement itself. Most likely, this deviation is due to the fact that the rigid registration method is based on a rigid registration of the ATV defined for a single bin. Therefore, the method does not take into account respiratory-related tumour deformation or, more importantly, motion artefacts within the different bins. Nevertheless, based on the general correlation between the ATV and the GTV displacements, the rigid registration method is found to be a convincing method to evaluate respiratory tumour motion and thereby to select the MidV bin. A method using derformabable registration to minimise the impact of artefacts on determination of the trajectory and shape and size of the tumour in the time-weighted midposition has been developed by Wolthaus et al. [Citation16], but needs to be commercialised and accessible. A free ware and easily accessible deformable registration software is being explored for the same purpose showing promising results [Citation17].
A possible drawback of the rigid registration method is that is relies on the HU in the proces of rigid registration. In case the tumour is located in close vicinity to the mediastinum or the thoracic wall the rigid registration throughout the bins may not be so consistent. This is not evaluated in the present study.
We used the displacement CoV of the GVT as a reference for tumour trajectory and to calculate the time-weighted midposition. This choice provides some weaknesses, as the CoV is also impacted by breathing-related deformations of the tumour as well as image artefacts, the latter probable being the most important [Citation8,Citation14,Citation18]. Also the manual delineation of the GTV has some inherent uncertainty [Citation13]. However, since the aim of the present study was to investigate easy assessments of the midposition, we chose CoV as these coordinates are accessible in most treatment planning systems making the method pragmatic and easy to transfer to other clinics using 4DCT.
The diaphragm and the carina methods seem to be very much affected by motion artefacts on the 4DCT scans compared to the visual and the rigid registration methods, and this might be the reason for the lower accuracy (). Since the registrations of the position of the diaphragmatic dome and the carina were based on a single CT slice, motion artefacts can be expected to have a greater impact on the evaluated diaphragm and carina displacement than on the displacement of the ATV and the GTV. In addition, the evaluated diaphragm and [Citation18] carina displacement were limited by the reconstructed slice thickness of 2 mm, as the spatial resolution was smallest in the CC direction. This often resulted in the diaphragmatic dome and the carina having the same distance to their time-weighted mean position for several bins. Therefore, even though the displacements of the diaphragm and the carina generally correlate well with the tumour displacement according to Cervino et al., van der Weide et al. and Spoelstra et al. [Citation19–21], the methods used in the present study to evaluate diaphragm and carina displacement seem to be too fragile. With a more sophisticated method to evaluate diaphragm and carina displacement, the accuracy of the associated methods may be improved. However, diaphragm and carina displacements are primarily related to the CC tumour displacement, i.e. even with a more sophisticated methods to evaluate the diaphragm and carina displacements, potential hysteresis in tumour motion patterns will not be accounted for.
Figure 4. CC peak-to-peak displacement of the diaphragm and the carina versus GTV. CC, cranio-caudal; GTV, gross tumour volume.
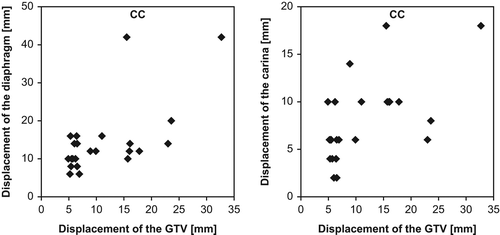
Based on these current data we conclude that the visual and semi-automatic rigid registration methods were equivalent in accuracy for selecting the MidV bin of a 4DCT scan. The methods based on diaphragm and carina displacement cannot be recommended without modifications.
Acknowledgements
The results of the present study were presented in part in a poster at the ICCR meeting 2010 in Amsterdam.
Declaration of interest: The authors report no conflicts of interest. The authors alone are responsible for the content and writing of the paper.
This work has been supported by a research grant from the Danish Center for Interventional Research in Radiation Oncology (CIRRO). Stine Korreman was supported by a research grant from Varian Medical Systems, Inc, up to 2010, and Carsten Brink was supported by a research grant from Electa AB.
References
- Chen GT, Kung JH, Beaudette KP. Artifacts in computed tomography scanning of moving objects. Semin Radiat Oncol 2004;14:19–26.
- Rietzel E, Pan T, Chen GT. Four-dimensional computed tomography: Image formation and clinical protocol. Med Phys 2005;32:874–89.
- Ford EC, Mageras GS, Yorke E, Ling CC. Respiration- correlated spiral CT: A method of measuring respiratory-induced anatomic motion for radiation treatment planning. Med Phys 2003;30:88–97.
- Keall P. 4-dimensional computed tomography imaging and treatment planning. Semin Radiat Oncol 2004;14:81–90.
- Low DA, Nystrom M, Kalinin E, Parikh P, Dempsey JF, Bradley JD, et al. A method for the reconstruction of four-dimensional synchronized CT scans acquired during free breathing. Med Phys 2003;30:1254–63.
- Pan T, Lee TY, Rietzel E, Chen GT. 4D-CT imaging of a volume influenced by respiratory motion on multi-slice CT. Med Phys 2004;31:333–40.
- Vedam SS, Keall PJ, Kini VR, Mostafavi H, Shukla HP, Mohan R. Acquiring a four-dimensional computed tomography dataset using an external respiratory signal. Phys Med Biol 2003;48:45–62.
- Persson GF, Nygaard DE, af Rosenschold PM, Vogelius IR, Josipovic M, Specht L, et al. Artifacts in conventional computed tomography (CT) and free breathing four- dimensional CT induce uncertainty in gross tumor volume determination. Int J Radiat Oncol Biol Phys 2011;80: 1573–80.
- Rietzel E, Pan T, Chen GT. Four-dimensional computed tomography: Image formation and clinical protocol. Med Phys 2005;32:874–89.
- Yamamoto T, Langner U, Loo BW Jr, Shen J, Keall PJ. Retrospective analysis of artifacts in four-dimensional CT images of 50 abdominal and thoracic radiotherapy patients. Int J Radiat Oncol Biol Phys 2008;72: 1250–8.
- Wolthaus JW, Schneider C, Sonke JJ, van HM, Belderbos JS, Rossi MM, et al. Mid-ventilation CT scan construction from four-dimensional respiration-correlated CT scans for radiotherapy planning of lung cancer patients. Int J Radiat Oncol Biol Phys 2006;65:1560–71.
- Keall PJ, Mageras GS, Balter JM, Emery RS, Forster KM, Jiang SB, et al. The management of respiratory motion in radiation oncology report of AAPM Task Group 76. Med Phys 2006;33:3874–900.
- Persson GF, Nygaard DE, Hollensen C, Munck af Rosensch ld P, Mouritsen LS, Due AK, et al. Interobserver delineation variation in lung tumour stereotactic body radiotherapy. Br J Radiol 2012;85:e654–e66.
- Persson GF, Nygaard DE, Brink C, Jahn JW, Munck af RP, Specht L, et al. Deviations in delineated GTV caused by artefacts in 4DCT. Radiother Oncol 2010;96: 61–6.
- Josipovic M, Persson GF, Logadottir A, Smulders B, Westmann G, Bangsgaard JP. Translational and rotational intra- and inter-fractional errors in patient and target position during a short course of frameless stereotactic body radiotherapy. Acta Oncol 2012;51:610–7.
- Wolthaus JW, Sonke JJ, van HM, Damen EM. Reconstruction of a time-averaged midposition CT scan for radiotherapy planning of lung cancer patients using deformable registration. Med Phys 2008;35:3998–4011.
- Brink C, Nielsen T, Persson G. Image improvement of 4D CT using deformable registrations of the respiration phases (abstract 1181). Radiat Oncol 2011;99:S440.
- Wu J, Lei P, Shekhar R, Li H, Suntharalingam M, D’Souza WD. Do tumors in the lung deform during normal respiration?An image registration investigation. Int J Radiat Oncol Biol Phys 2009;75:268–75.
- Cervino LI, Chao AK, Sandhu A, Jiang SB. The diaphragm as an anatomic surrogate for lung tumor motion. Phys Med Biol 2009;54:3529–41.
- van der Weide L, van Sornsen de Koste JR, Lagerwaard FJ, Vincent A, van TB, Slotman BJ, et al. Analysis of carina position as surrogate marker for delivering phase-gated radiotherapy. Int J Radiat Oncol Biol Phys 2008;71:1111–7.
- Spoelstra FO, van Sornsen de Koste JR, Vincent A, Cuijpers JP, Slotman BJ, Senan S. An evaluation of two internal surrogates for determining the three-dimensional position of peripheral lung tumors. Int J Radiat Oncol Biol Phys 2009;74:623–9.