To the Editor,
The brainstem encompasses the midbrain, pons, and medulla. Brainstem gliomas arise at any age, but most frequently occur during childhood. In children, brainstem gliomas constitute approximately 10–20% of the malignancies affecting the central nervous system (CNS) [Citation1], and the average age at diagnosis is 7–9 years with no gender predilection [Citation2,Citation3]. There are approximately 150–300 new cases diagnosed in USA annually [Citation2]. The diagnosis of brainstem glioma includes a histopathologically diverse number of tumor types, which makes it difficult to assign an overall prognosis [Citation4]. Similar to tumors in other CNS sites, however, low-grade brainstem gliomas are curable with current treatment modalities, whereas high-grade gliomas are often fatal despite aggressive treatment [Citation5].
Of those children diagnosed with brainstem gliomas, approximately 20% are low-grade gliomas [Citation2,Citation3]. There is variability with regard to the resectability of these tumors; however, in general, complete resection is often impossible [Citation2,Citation3,Citation6–8]. External-beam radiation therapy is an accepted and effective treatment modality for patients with unresectable low-grade brainstem gliomas [Citation1,Citation5–8]. When determining the therapeutic ratio, the risks of radiation therapy must be considered along with the potential benefits [Citation9]. As the best 10-year survival rates mandate a relatively high radiation dose deposited in radiosensitive tissue, children treated for brainstem gliomas with radiation therapy are subject to a number of late sequelae, including hearing loss, neuroendocrine deficits, chronic otitis media, neurocognitive dysfunction, and the development of secondary malignancies [Citation9].
There are few contemporary studies in the literature directly addressing the treatment of low-grade pediatric brainstem gliomas. In general, to minimize the risk of late effects in pediatric patients treated with radiation, investigators have historically sought to attenuate the dose to non-target, healthy tissues. For treatment of low-grade brainstem gliomas, recent studies have sought to investigate the role of gamma knife surgery to reduce dose to non-target tissue [Citation10] and therapies of increased conformality, such as photon-based intensity-modulated radiation therapy (IMRT) and proton therapy [Citation11]. Technology facilitating the delivery of highly conformal radiation therapy, such as IMRT and proton therapy, may allow for better sparing of non-target tissues. In this study, we sought to investigate the relative dosimetric features of photon IMRT and proton therapy in the treatment of low-grade brainstem gliomas in order to assess their potential value in reducing late toxicity.
Material and methods
Following approval of our institutional review board, three pediatric patients with biopsy-proven low-grade brainstem gliomas were chosen from our institution's treatment planning database. The patients selected were diagnosed with gliomas located in varying positions within the brainstem, from the rostral to the caudal brainstem, to roughly correspond to the midbrain, pons, and medulla. Head and cervical spinal cord computed tomography (CT) and magnetic resonance imaging (MRI) images were imported into the EclipseTM Proton Planning (Varian Medical Systems, Palo Alto, CA, USA) treatment planning system (TPS). Image fusions between the CT and MRI image sets were performed using a mutual information-based technique. For patients 1 and 2, the CT and MRI slice thickness was 1.5 mm. For patient 3, the CT slice thickness was 3 mm and MRI slice thickness was 7.5 mm. The gross tumor volume (GTV), clinical target volume (CTV), and organs-at-risk (OARs) were contoured on each MRI dataset for each patient and then transferred onto the fused CT images. The GTV was the tumor volume defined on contrast-enhanced T1 and T2 MRI sequences. The CTV was an anatomically constrained 10-mm expansion of the GTV, customized to include suspicious edema. The planning target volume (PTV) was a 3-mm uniform expansion from the CTV, consistent with institutional protocol when daily image guidance is utilized. The proton therapy treatment plans were generated in the EclipseTM TPS. The CT datasets and original identical contours were then exported into the PinnacleTM TPS (Philips Electronics, Andover, MA, USA) for IMRT planning.
At our institution, both proton therapy and IMRT treatment plans for all pediatric CNS and base of skull tumors are evaluated using an institutional pediatric CNS dosimetry checklist. This checklist is the basis for an algorithm under which OAR tolerances are grouped into three levels. Level 1 (highest priority) includes the cervical spinal cord, optic chiasm, brainstem surface, and brainstem core. Level 2 (intermediate priority level) includes the bilateral retinae, optical nerves, cochleae, bilateral temporal lobes, hypothalamus, and pituitary gland. Level 3 (lower priority level) includes the hippocampi, bilateral lacrimal glands, posterior nasopharynx, bilateral mastoid air cells, scalp, supratentorial brain, and non-target whole brain. The sparing of higher-priority organs is emphasized through iterative treatment planning.
In proton treatment planning, for each field, target distal and proximal margins are based on the CTV and the aperture margin is based on the PTV. The actual size of the distal, proximal, and aperture margins for each beam depend on each field range and beam path heterogeneity. This is consistent with previous publications [Citation12] and ICRU guidelines [Citation13]. The target-smearing margin was set at 5 mm uniformly to account for setup uncertainty. The target coverage was normalized such that 99% of the CTV received 100% of the prescribed dose. The relative dose to 95% of the PTV was at least 96% in all three patients for both plans (Supplementary Tables I–III available online at http://www.informahealthcare.com/doi/abs/10.3109/0284186X.2013.767474), available online at http://www.informahealthcare.com). Three non-coplanar beams were used to optimize target coverage as well as spare OARs for each of the proton plans. For IMRT treatment planning, 6-MV photon beams were used for all of the patients. The plans were optimized to cover the PTV and spare the OARs according to the same institutional guidelines. For patients 1 and 2, 11 non-coplanar beams were used in the IMRT plans and for patient 3, with the caudal brainstem tumor, nine coplanar beams were used. The dose-volume histograms (DVH) were exported from both TPSs and analyzed to assess plan quality. Furthermore, integral dose (excluding dose to the CTV) for each treatment plan was also calculated to compare total energy deposition from treatment in each patient.
Statistical analyses were not appropriate due to the nature of the study and the limited utility of p-values when comparing dose levels. Instead, clinical significance was attributed based on organ site and dose range in accordance with QUANTEC data when available and supplemented by institutional dose-tolerance guidelines.
Results
Target coverage goals were met in all three cases. The brainstem glioma subsites of the patients included in our study are shown () for reference as well as to demonstrate spatial relationships with the surrounding non-target tissues. The color-wash dose distributions for both the IMRT and proton therapy treatment plans are shown in . Although the majority of healthy tissue received considerably less dose with proton therapy, doses to most of the OARs (including the cochlea) were well below acceptable institutional thresholds for both the IMRT and proton therapy plans. (Supplementary Tables I–III available online at http://www.informahealthcare.com/doi/abs/10.3109/0284186X.2013.767474) show the dosimetric data for organs with a clinically relevant difference in dose between the two plans. In all three patients, the IMRT plans were more heterogeneous, where larger areas received ≥ 105% of the dose. The IMRT plans also uniformly involved more than double the integral radiation dose to non-tumor normal tissue. Otherwise, the specific organs spared and the magnitude of dose difference was dependent on the general location of the tumor within the brainstem.
Figure 1. Coronal sections of patients 1–3 (from left to right) representing brainstem glioma location within brainstem with GTV outlined (red).
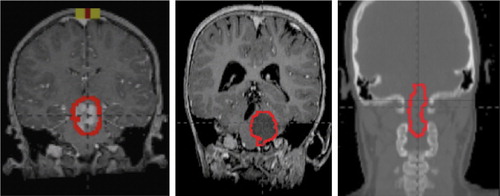
Figure 2. (A) Patient 1 axial (top) and coronal, sagittal (bottom) isodose distribution. (B) Patient 2 axial (top) and coronal, sagittal (bottom) isodose distribution. (C) Patient 3 axial (top) and coronal, sagittal (bottom) isodose distribution.
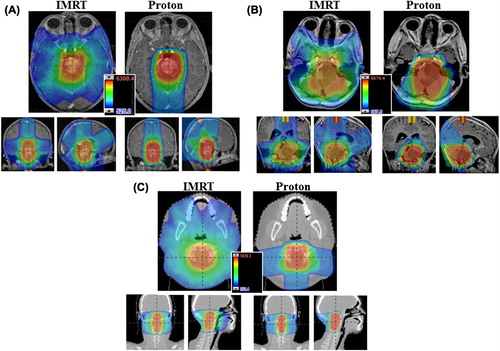
Patient 1 had a low-grade glioma of the rostral brainstem with a volume of 34 cm3. The heterogeneity of both plans was permissible. Supplementary Tables I (available online at http://www.informahealthcare.com/doi/abs/10.3109/0284186X.2013.767474) lists those organs with differences in normal-tissue doses between the two plans: notably the mean dose to the pituitary as well as right and left temporal lobes were observed to be significantly different between the proton and IMRT photon plans.
Patient 2 had a 44-cm3 low-grade glioma in a mid cranio-caudal location within the brainstem (Supplementary Tables II available online at http://www.informahealthcare.com/doi/abs/10.3109/0284186X.2013.767474). Clinically significant differences were observed in dose deposited to the pituitary, hypothalamus, supratentorial brain, left and right temporal lobes, right hippocampal head and tail, and posterior nasopharynx as well as the total integral dose (Supplementary Tables II available online at http://www.informahealthcare.com/doi/abs/10.3109/0284186X.2013.767474).
The dosimetric data from patient 3 with the 40-cm3 caudal brainstem tumor demonstrated less significant differences between plans (Supplementary Tables III available online at http://www.informahealthcare.com/doi/abs/10.3109/0284186X.2013.767474). The main tissue with a potentially significant dose difference was the posterior nasopharynx. The mean dose to the posterior nasopharynx was 29.5 Gy with the IMRT plan and 6.7 CGE with the proton plan. The mean dose to the parotids was > 15 Gy with the IMRT plan and < 1.5 CGE with the proton plan.
Discussion
Brainstem gliomas comprise a significant proportion (20%) of all brain tumors affecting pediatric patients and are classified according to grade [Citation14]. Although recent advances in imaging and neurophysiological monitoring have led to an increase in the use of surgical intervention, the location of many brainstem tumors limits the possibility of complete surgical resection [Citation15]. Radiation therapy thus remains the mainstay of treatment in this group [Citation5,Citation7,Citation8,Citation15]. Although patients with low-grade brainstem gliomas are frequently cured with definitive radiotherapy, the high radiation dose can cause permanent late side effects and complications [Citation2,Citation9]. The risks of chronic morbidity and the development of secondary malignancies are of primary concern in any pediatric patient where a long life expectancy is common [Citation9]. Most notably, pediatric patients may experience neuroendocrine dysfunction, sensorineural hearing loss, neurocognitive deficits, and chronic otitis media. In our study, we sought to investigate the role of highly conformal radiation therapy (IMRT and proton therapy) in the reduction of dose to non-target tissue as an indicator of risk for developing late effects. We found that for all patients included in our study, the high conformality of IMRT and proton therapy may be leveraged to spare the cochlea bilaterally without compromising target coverage. Depending on the brainstem region, proton therapy further demonstrated the potential to reduce dose and thereby possibly lower the risk of chronic otitis media, neurocognitive deficits, neuroendocrine dysfunction, and radiation-induced second malignancies in patients who will become long-term survivors.
The most common pituitary and hypothalamic abnormalities observed after irradiation are growth hormone deficits. It has been demonstrated that 1–3 days after a 3-week course of cranial radiation therapy to 24 Gy, growth hormone levels were lower both at hourly intervals and peak concentrations when compared to pretreatment levels [Citation3,Citation16]. Various subsequent studies confirm this relationship with similar findings, and indicate that the threshold dose for injury may be as low as 5 Gy to the hypothalamus [Citation17–19]. Growth hormone deficits caused by radiation are permanent [Citation3,Citation16] and exogenous growth hormone-replacement therapy is expensive. Our study indicates that patients with rostral or middle brainstem tumors would receive less non-target dose and possibly fewer late effects, thus avoiding the need for growth hormone therapy when treated with proton therapy instead of photon-based radiotherapy.
Another late effect of radiation therapy in pediatric patients is sensorineural hearing loss. The cochleae have a low threshold for irradiation and are often damaged in pediatric patients treated for brainstem gliomas [Citation20]. Our data suggest that both IMRT and proton therapy can spare sufficient volumes of the cochlea and, in this regard, proton therapy may not provide a clinically relevant superior dose profile. Otitis media with effusion and otomastoid opacification, however, have been shown in the literature to be common sequelae of radiation therapy to the posterior nasopharynx, negatively impacting hearing outcomes independent of cochlear dose [Citation21,Citation22]. In a multivariate analysis, Walker et al. demonstrated that mean doses of > 30 Gy to the posterior nasopharynx were associated with grade 2–3 effusions and otomastoid opacification [Citation21]. In our study, two of three patients received doses of approximately 30 Gy or greater with the IMRT plans. The proton plans consistently kept the posterior nasopharynx dose below this threshold, which would suggest a low likelihood of chronic otitis media and secondary hearing loss.
Irradiated children who become long-term survivors of brainstem gliomas may also experience a decline in neurocognitive function, resulting in an overall decreased intelligence quotient (IQ) with subsequent academic difficulties [Citation23]. Variables found to be particularly predictive of the degree of neurocognitive decline post-radiation were age at diagnosis and extent of radiation received [Citation23]. Patients younger than three years of age show the greatest degree of post-radiation impairment, as the immature brain is thought to be more sensitive to developing detriments from ionizing radiation [Citation23]. This finding is true even for moderate doses: A retrospective Pediatric Oncology Group study assessing cognitive function after radiation therapy for medulloblastoma found that patients randomly assigned to receive a reduced dose of craniospinal irradiation (CSI) (23.4 Gy), and those who were older at the time of diagnosis (> 8.8 years old), demonstrated higher cognitive functioning than patients randomly assigned to receive standard-dose CSI (36 Gy) and those who were younger [Citation23]. There is also evidence from the Childhood Cancer Survivorship Study (CCSS) data to suggest that there are lower rates of employment, education, and marriage associated with radiotherapy doses greater than 50 Gy to the temporal and frontal lobes [Citation24]. These deficits were independent of the dose received by the posterior fossa or parietal-occipital lobe regions. Our data would suggest that the differences in dose deposited to the temporal lobes in two of the three patients in this study might translate into fewer late neurocognitive deficits and possibly better social adjustment [Citation25,Citation26].
Late secondary malignancies are also of concern when treating pediatric patients with irradiation. Young children treated for CNS tumors are at a particularly high risk for developing secondary malignancies [Citation27]. Many of these risks are underreported and underappreciated because secondary malignancies primarily manifest decades following treatment. A recent study of 370 pediatric patients treated at the University of Florida Proton Institute demonstrated that actuarial incidences of secondary malignancies after photon-based radiotherapy to the CNS were 8% and 24% at 20 and 30 years of follow-up, respectively [Citation27]. Quantification of the integral doses in our study demonstrate a significant (> 50% lower) difference between the proton and IMRT plans in all three patients, these findings may suggests that the risk of a secondary malignancy would be reduced with proton therapy. Even patients with tumors in the cervico-medullary region, such as patient 3, where the parotid glands are the only sites where appreciable differences are demonstrated between the two planning approaches, receive a notably reduced integral dose with proton therapy.
In long-term survivors of pediatric CNS tumors, the frequency and severity of irreversible complications following radiation have prompted investigations into how to improve target conformality. There is a growing body of evidence to suggest that proton therapy can improve low- and moderate-dose conformation when compared to standard or 3D conformal therapy, and that patients might experience less late complications as a result. Our current study suggests that, specifically in patients with low-grade brainstem tumors, reducing the dose deposited to the surrounding uninvolved tissues may reduce late sequlae and that proton therapy represents an effective modality for reducing the dose deposition to non-target tissues. Moreover, the magnitude and type of benefit may be predicted based on the brainstem subsite.
This study presents dosimetric data necessary for the development of clinical studies and prospective trials to address the optimization of treatment for low-grade pediatric brainstem gliomas in order to reduce late effects. While limited by the inclusion of few patients and the dosimetric nature of the study, here we provide quantifiable evidence of the utility of technologies with increased conformality in the treatment of low-grade brainstem gliomas. These data may also be utilized to inform patient prioritization, a critical issue given the relatively few proton therapy allotments currently allocated to pediatric patients.
Supplementary Tables I–III
Download PDF (24.6 KB)Declaration of interest: The authors report no conflicts of interest. The authors alone are responsible for the content and writing of the paper.
References
- Combs SE, Steck I, Schulz-Ertner D, Welzel T, Kulozik AE, Behnisch W, . Long-term outcome of high-precision radiotherapy in patients with brain stem gliomas: Results from a difficult-to-treat patient population using fractionated stereotactic radiotherapy. Radiother Oncol 2009;91:60–6.
- Walker DA, Punt JA, Sokal M. Clinical management of brain stem glioma. Arch Dis Child 1999;80:558–64. [Review].
- Freeman CR, Farmer JP. Pediatric brain stem gliomas: A review. Int J Radiat Oncol Biol Phys 1998;40:265–71. [Review].
- Louis DN, Ohgaki H, Wiestler OD, Cavenee WK, Burger PC, Jouvet A, . The 2007 WHO classification of tumours of the central nervous system. Acta Neuropathol 2007;114: 97–109. [Review].
- Edwards MS, Prados M. Current management of brain stem gliomas. Pediatr Neurosci 1987;13:309–15. [Review].
- Vesper J, Bolke B, Wille C, Gerber PA, Matuschek C, Peiper M, . Current concepts in stereotactic radiosurgery – a neurosurgical and radiooncological point of view. Eur J Med Res 2009;14:93–101.
- Knab B, Connell PP. Radiotherapy for pediatric brain tumors: When and how. Expert review of anticancer therapy. 2007;7:S69–77.
- Kirsch DG, Tarbell NJ. Conformal radiation therapy for childhood CNS tumors. Oncologist 2004;9:442–50.
- Fuss M, Hug EB, Schaefer RA, Nevinny-Stickel M, Miller DW, Slater JM, . Proton radiation therapy (PRT) for pediatric optic pathway gliomas: Comparison with 3D planned conventional photons and a standard photon technique [In Process Citation]. Int J Radiat Oncol Biol Phys 1999;45:1117–26.
- Yen CP, Sheehan J, Steiner M, Patterson G, Steiner L. Gamma knife surgery for focal brainstem gliomas. J Neurosurg 2007;106:8–17.
- Hug EB, Muenter MW, Archambeau JO, DeVries A, Liwnicz B, Loredo LN, . Conformal proton radiation therapy for pediatric low-grade astrocytomas. Strahlenther Onkol 2002;178:10–7.
- Swanson EL, Smith A, Morris CG, Galloway TJ, Marcus Jr RB, Amdur R. Long-term outcomes following radiotherapy for ependymoma. Int J Radiat Oncol Biol Phys 2009;75(3):S231.
- International Commission on Radiation Units and M. Prescribing, recording, and reporting proton-beam therapy. J ICRU 2007;7:220.
- Siu IM, Tyler BM, Chen JX, Eberhart CG, Thomale UW, Olivi A, . Establishment of a human glioblastoma stemlike brainstem rodent tumor model. J Neurosurg Pediatr 2010;6:92–7.
- Hong S, Kim IH, Wang KC. Outcome and prognostic factors of childhood diffuse brainstem glioma. Cancer Res Treat 2005;37:109–13.
- Dacou-Voutetakis C, Xypolyta A, Haidas S, Constantinidis M, Papavasiliou C, Zannos-Mariolea L. Irradiation of the head. Immediate effect on growth hormone secretion in children. J Clin Endocrinol Metab 1977;44:791–4.
- Kaplan AM, Albright AL, Zimmerman RA, Rorke LB, Li H, Boyett JM, . Brainstem gliomas in children. A Children’s Cancer Group review of 119 cases. Pediatr Neurosurg 1996;24:185–92.
- Dunkel IJ, Garvin JH,Jr, Goldman S, Ettinger LJ, Kaplan AM, Cairo M, . High dose chemotherapy with autologous bone marrow rescue for children with diffuse pontine brain stem tumors. Children’s Cancer Group. J Neurooncol 1998;37:67–73.
- Jennings MT, Sposto R, Boyett JM, Vezina LG, Holmes E, Berger MS, . Preradiation chemotherapy in primary high-risk brainstem tumors: Phase II study CCG-9941 of the Children’s Cancer Group. J Clin Oncol 2002;20:3431–7.
- Hua C, Bass JK, Khan R, Kun LE, Merchant TE. Hearing loss after radiotherapy for pediatric brain tumors: Effect of cochlear dose. Int J Radiat Oncol Biol Phys 2008;72: 892–9.
- Walker GV, Ahmed S, Allen P, Gidley PW, Woo SY, Demonte F, . Radiation-induced middle ear and mastoid opacification in skull base tumors treated with radiotherapy. Int J Radiat Oncol Biol Phys 2011;81:e819–23.
- Young YH, Cheng PW, Ko JY. A 10-year longitudinal study of tubal function in patients with nasopharyngeal carcinoma after irradiation. Arch Otolaryngol Head Neck Surg 1997; 123:945–8.
- Mulhern RK, Kepner JL, Thomas PR, Armstrong FD, Friedman HS, Kun LE. Neuropsychologic functioning of survivors of childhood medulloblastoma randomized to receive conventional or reduced-dose craniospinal irradiation: A Pediatric Oncology Group study. J Clin Oncol 1998;16:1723–8.
- Schultz KA, Ness KK, Whitton J, Recklitis C, Zebrack B, Robison LL, . Behavioral and social outcomes in adolescent survivors of childhood cancer: A report from the childhood cancer survivor study. J Clin Oncol 2007;25:3649–56.
- Armstrong GT, Jain N, Liu W, Merchant TE, Stovall M, Srivastava DK, . Region-specific radiotherapy and neuropsychological outcomes in adult survivors of childhood CNS malignancies. Neuro-oncology 2010;12:1173–86.
- Blomstrand M, Brodin NP, Munck Af Rosenschold P, Vogelius IR, Sanchez Merino G, Kiil-Berthlesen A, . Estimated clinical benefit of protecting neurogenesis in the developing brain during radiation therapy for pediatric medulloblastoma. Neuro-oncology 2012;14:882–9.
- Galloway TJ, Indelicato DJ, Amdur RJ, Swanson EL, Smith AA, Marcus RB. Second tumors in pediatric patients treated with radiotherapy to the central nervous system. Am J Clin Oncol 2012;35:279–83.