Abstract
Background. Higher doses to NSCLC tumours are required to increase the low control rates obtained with conventional dose prescriptions. This study presents the concept of inhomogeneous dose distributions as a general way to increase local control probability, not only for isolated lung tumours but also for patients with involved lymph nodes. Material and methods. Highly modulated IMRT plans with homogeneous dose distributions with a prescribed dose of 66Gy/33F were created for 20 NSCLC patients, staged T1b-T4 N0-N3, using standard PTV dose coverage of 95–107%. For each patient, an inhomogeneous dose distribution was created with dose constraints of: PTV-coverage ≥ 95%, same mean lung dose as obtained in the homogeneous dose plan, maximum doses of 45 and 66 Gy to spinal canal and oesophagus, respectively, and V74Gy < 1 cm3 for each of: aorta, trachea + bronchi, the connective tissue in mediastinum, and the thorax wall. The dose was escalated using a TCP model implemented into the planning system. The difference in TCP values between the homogeneous and inhomogeneous plans were evaluated using two different TCP models. Results. Dose escalation was possible for all patients. TCP values based on assumed homogeneous distribution of clonogenic cells either in the GTV, CTV or PTV showed absolute TCP increases of approximately 15, 10 and 5 percentage points, respectively. This increase in local control was obtained without increasing the mean lung dose. However, small increases in maximum doses to the mediastinum were observed: 2.5 Gy for aorta, 4.4 Gy for the connective tissue, 1.6 Gy for the heart, and 2.6 Gy for trachea + bronchi. Conclusion. Increased target doses and TCP values using inhomogeneous dose distributions could be achieved for all patients, regardless of lymph node involvement, tumour stage, location, and size. These new treatment plans have the potential to increase the local tumour control by 10–15 percentage points without compromising the clinically acceptable lung toxicity level.
Survival rates for non-small cell lung cancer (NSCLC) patients treated with standard radiotherapy in 30–33 fractions are very poor. Local failure often occurs in the primary tumour suggesting higher doses than the standard 60–70 Gy are required in order to obtain a better local tumour control [Citation1,Citation2].
Planning studies have shown that it is possible to escalate the dose in order to increase the tumour dose while maintaining an acceptable toxicity level for the organs at risk [Citation3–5]. Clinical studies report an increase in overall survival for increasing radiation doses [Citation6,Citation7]. In accordance with the International Commission on Radiation Units and Measurements (ICRU) Report 50 [Citation8], the dose to the planning target volume (PTV) should be kept within 95–107% of the prescribed dose. However, when the upper dose limit of 107% is disregarded, a reduction in treatment volume combined with a dose escalation can be used to increase target dose with no increase in mean lung dose (MLD), i.e. no increased risk of pneumonitis [Citation9–11]. This method results in more inhomogeneous dose distributions although, in the above studies, plans were created from dose scaling of the underlying 95–107% homogeneous plan.
These promising results have paved the way for investigating whether truly inhomogeneous dose distributions created directly during plan optimisation could lead to even larger target doses and/or sparing of the healthy tissue. Schwarz et al. [Citation12] has used inhomogeneous dose distributions as a way to perform dose escalation. An inhomogeneous dose distribution based only on the mean dose to the planning volume was created if standard dose escalation was not feasible, which was the case for five of their intensity-modulated radiation therapy (IMRT) patients. Their patients were selected based on a tumour location in the superior half of the lung and the dose calculations were evaluated without accounting for geometrical uncertainties, such as setup errors and respiration.
In this current study inhomogeneous dose distributions are presented as a method to increase the expected tumour control probability (TCP) for all NSCLC patients regardless of tumour location. Geometrical uncertainties arising from setup errors and respiration are included in patient specific PTV margins.
Material and methods
This study is based on 20 NSCLC patients and the patient characteristics are listed in . Treatment planning was based on the mid ventilation phase of a four-dimensional computed tomography (4D-CT) scan with slice thickness of 2.5 mm [Citation13]. The gross tumour volume (GTV) was delineated based on the planning-CT and fluorodeoxyglucose positron emission tomography (FDG-PET) scans, and consists of both the primary tumour and involved lymph nodes (16 patients). The clinical target volume (CTV) was made as an expansion of the GTV by 10 mm in mediastinum and 5 mm in lung tissue excluding bony structures and major vessels in accordance with recommendations provided by the Danish Oncology Lung cancer Group (DOLG). The PTV was created as a patient specific expansion of the CTV with a range of 7–9 mm. This margin was based on an assumption of daily online imaging evaluated on soft tissue [Citation14].
Table I. Patient characteristics and planning results for the lungs.
Two IMRT treatment plans were created for each patient with 33 fractions: 1) a standard homogeneous dose distribution; and 2) an inhomogeneous dose distribution. The treatment plans were created within Pinnacle (Philips Healthcare, research version 8.1) for an Elekta Synergy accelerator (MLCi2 leaf bank, 2 × 40 leaves) using 6 MV. The dose calculation was performed using the Collapsed Cone (CC) algorithm with density correction and evaluated on a 2 mm dose grid. The plans were evaluated by a radiation oncologist in accordance with the standard clinical procedure.
Homogeneous dose distribution
Five to seven co-planar treatment fields were unevenly distributed for each patient such that unnecessary irradiation of the contra lateral lung and heart could be avoided. The dose criteria were: PTV coverage of 95–107% of the prescribed 66 Gy, maximum dose of 45 Gy and 66 Gy for spinal canal and oesophagus, respectively, and V20Gy for the lungs excluding GTV less than 40%. The optimisation was steered towards as low a value for V20Gy as possible, i.e. an initial value less than 40% was not accepted but was decreased even further whenever possible. The heart was not considered a dose- limiting organ [Citation15].
Inhomogeneous dose distribution
For each patient, the same beam and collimator angles as for the homogeneous treatment plans were used. The dose constraints were: PTV coverage of ≥ 95% of the prescribed dose, same MLD as in the homogeneous plan, and maximum dose of 45 Gy and 66 Gy to the spinal canal and oesophagus, respectively, and a maximum dose constraint of V74Gy < 1 cm3 for each of the following delineations: aorta, trachea + bronchi, heart, connective tissue in mediastinum, and thorax wall. The connective tissue in mediastinum is defined as tissue in the mediastinum which is not included as aorta, trachea, bronchi, heart or oesophagus.
For each patient, a “boost” optimisation region was constructed by expanding the GTV with the same expansion size as used to create the standard PTV from the CTV. The dose to this region was enhanced as much as possible in order to increase the dose to the central part of the tumour. This was accomplished by explicitly maximising the TCP to this region during optimisation using a TCP model that was implemented into the IMRT optimisation algorithm (Equation 2). While the dose to the boost optimisation region was increased, the standard PTV was covered with a minimum dose of 95% of the prescribed dose in order to ensure sufficient dose coverage. There were no limitations on the maximum dose to parts of the PTV that were outside the boost volume, i.e. only the surrounding normal tissues limited the dose to the PTV.
TCP models
Two different TCP models were used to evaluate the treatment plans:
The first model is based on the TCP model from Martel et al. [Citation16],
where D50 = 84.5 Gy and γ = 1.5, giving local progression-free survival at 30 months. This model was used to evaluate TCP values for all different dose voxels (Di). The combined TCP value was obtained by multiplication of these values
where Vi is the relative volume related to dose voxel Di. Since only the relative volumes are included in TCPM, the model is independent of the absolute tumour volume.
The second model is a volume-dependent TCP model based on the linear-quadratic equation which as function of the single-hit factor (α) is defined as
where Ni is the number of clonogenic cells in the dose bin i related to the dose Di, α/β = 10 Gy, and K = 33 fractions [Citation17]. The distribution of the clonogenic cells is assumed to be homogeneous thus Ni is proportional to Vi. The total number of clonogenic cells is defined by a cell density ρ times the patient specific volume of the GTV. In order to include α heterogeneity in the patient population, Equation 3 is averaged over a distribution of α values [Citation17]:
where and σα describes the average and standard deviation of the Gaussian-like distribution of α values. The values of ρ = 105 cm−3 and σα = 0.05 Gy−1 were obtained from Webb [Citation18] as best fit to clinical squamous cell carcinoma data of the upper respiratory and digestive tracts. The value of
was adjusted relative to the value quoted by Webb in order to produce clinically relevant TCP values for the patients in the current study. The value of
= 0.155 Gy−1 was chosen such that the TCP value for a uniform irradiated volume of 56.7 cm3 (median GTV volume in this study) corresponded to the TCP value predicted by the Martel equation (Equation 2).
The TCP value depends on the dose distribution and the distribution of clonogenic cells, which is assumed uniform in this study. However, it might be an overestimate of the TCP value to assume that all the clonogenic cells are located within the GTV. Therefore, a TCP calculation based on the same number of clonogenic cells but distributed uniformly within the CTV has also been performed in order to produce more conservative estimates of the TCP value. Similarly, TCP calculations were performed for the PTV with the same number of clonogenic cells in order to verify that the treatment plans with inhomogeneous dose distributions does not diminish the expected outcome compared to standard homogeneous dose distributions.
Results
A typical DVH is shown in . It is seen that not all parts of the tumour were escalated to the same dose level. It is also seen that the maximum lung dose was increased for the inhomogeneous plan but that this was compensated by less volume irradiated to lower doses (inhomogeneous lung curve is slightly below that for the homogeneous plan in the dose interval 5–50 Gy). Additional data is available in the online Supplementary Figures 1 and 2 at http://informahealthcare.com/doi/abs/10.3109/ 0284186X.2013.790560, where DVHs for all patients are displayed.
Figure 1. DVH for the homogeneous (black) and inhomogeneous (grey) dose distributions. Dashed lines: GTV, solid lines: CTV, dotted lines: PTV and dash-dotted lines: Lungs.
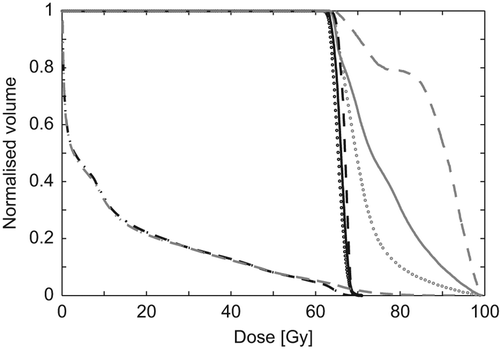
Tumour
Results from DVH evaluations and TCP calculations are listed in . For the inhomogeneous plans, minimum, mean, and maximum doses were larger compared to the homogeneous plans. The doses in the homogeneous plans were a priori situated within 95–107% of the prescribed dose of 66 Gy. Hence, for the homogeneous plans there were no major dose differences between the patients.
Table II. Planning results for the homogeneous and inhomogeneous plans.
The variation of the TCPM was less than the variation of the TCPLQ due to the lack of dependence of the absolute tumour volume in the Martel model. The absolute TCP values were almost constant in the homogeneous plans for the calculations based on the GTV, CTV, and PTV, which reflects the homogeneous dose distribution in these volumes. For the inhomogeneous plans, a variation in the calculated TCP values was seen. The cause of this was the, on average, larger dose escalation in the central parts of the tumour.
The increase in TCP values going from homogeneous to inhomogeneous plans for each patient is displayed in for both TCP models. The patients are ordered by study id which is sorted according to the gain in TCPM calculations for the PTV. Although differences exist between the TCP values calculated by the two models, the overall trend is similar, with approximately absolute TCP increases of 15, 10 and 5 percentage points for the GTV, CTV, and PTV calculations, respectively.
Figure 2. Histograms displays the difference in TCP for the inhomogeneous plan compared to the homogeneous plan for each patient. The left column shows the TCPM gain using the Martel model (Eq. 2), and in the righ column is shown the TCPLQ gain calculated using the LQ model (Eq. 4). The three rows represent calculations for clonogenic cells assumed to be homogeneous distributed over either PTV, CTV, or GTV.
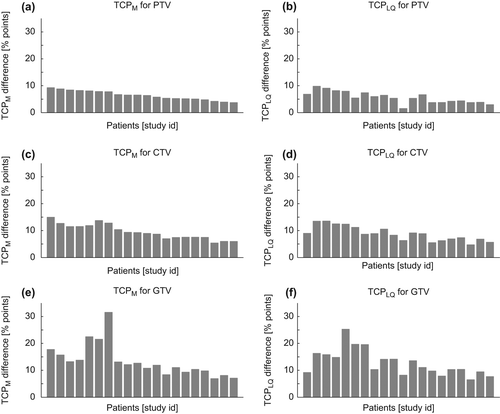
Normal tissue
The objective for the MLD was to maintain the same level in the inhomogeneous plan as obtained with the homogeneous plan and it was achieved within 0.03 Gy for each patient. The dose to the organs at risk in the mediastinum region are visualised in , where the maximum dose (D1cm3) in the homogeneous plan versus the inhomogeneous plan is plotted for all patients. On average, there was an increase in the maximum dose of 2.5 Gy for aorta, 4.4 Gy for the connective tissue, 1.6 Gy for the heart, and 2.6 Gy for trachea+ bronchi.
Figure 3. Maximum doses (D1cm3) to the organs at risk in the mediastinum area for the homogeneous versus inhomogeneous dose distributions. Each marker represents a patient. The diagonal lines are the identity lines (y = x).
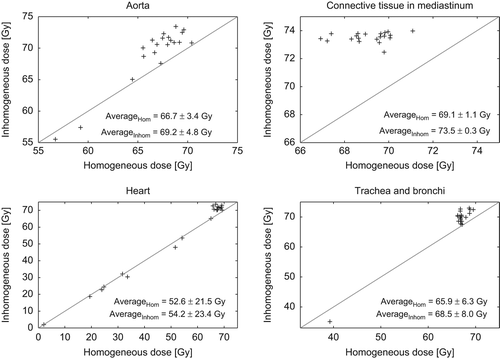
The change in mean heart dose was less than ± 1 Gy when changing from the homogeneous to the inhomogeneous plan, except for one patient, who had a decrease of 3 Gy.
Discussion
For all patients absolute TCP increased by use of inhomogeneous plans. There is no indication of tumours located in the upper part of the lung are better candidates for dose escalation than tumours located in the lower part of the lung. Most of the patients had lymph node involvement and for some of these it was possible to obtain even higher TCP gain than the patients which did not have involved lymph nodes. The two TCP models are somewhat different in nature (e.g. dependence of absolute tumour volume) and the values of the calculated TCP are therefore different. Nevertheless, the gain in TCP was quite similar indicating that changes in TCP are more robust than actual TCP values. The TCP gain was smallest for the PTV calculations and largest for the GTV calculations which simply reflect that the dose distribution was peaked in the centre of the tumour. Hence, the TCP gain in using inhomogeneous plans is obviously largest if the main portion of the clonogenic cells is situated centrally in the tumour. But even for the extreme case in which all the clonogenic cells are homogeneous distributed over the entire PTV, use of inhomogeneous plans results in an increase in TCP value. The distribution of the clonogenic cells within the PTV is not intuitive since the PTV is a volume used for geometrical uncertainties and not subclinical disease. Calculations related to a distribution of clonogenic cells within the PTV are only performed in order to measure the increase in TCP if the clonogenic cells are irradiated with a dose distribution represented by the one calculated for the PTV. This compensates for the effect that the dose distribution calculated for the CTV might not be indicative for the true dose distribution received by the clonogenic cells due to inter- and intra-fractional uncertainties. The clonogenic cells could theoretically be placed entirely on the surface of the CTV. If that is the case, the calculated increases in TCP by use of inhomogeneous plans would be less since the average increase in dose is higher for the entire CTV than for the rim of the CTV. The effect of such a strange distribution is to some degree reduced by applying the dose distribution related to the entire PTV and not just the dose distribution of the CTV.
Due to dose constraints in mediastinum the achievable dose level for the involved lymph node in the inhomogeneous plan depended on the node location. Dose escalation for patients with lymph node involvement based on homogeneous dose distributions would therefore be limited to a dose level of approximately 66–74 Gy, also for the part of the tumour located in the lung tissue. The idea of homogeneous dose distributions is based on the fact that for a homogeneous distribution of clonogenic cells and a given energy available for deposition, the highest TCP value is obtained for a homogeneous dose distribution [Citation17]. However, this result does not include normal tissue constraints, which for homogeneous dose distributions limits the dose to the entire tumour. In such a case, a homogeneous dose distribution will not produce the highest achievable TCP value.
The MLD is often used as a surrogate for lung toxicity [Citation9], so equal lung toxicity is expected for the homogeneous and inhomogeneous plans in this study. It might be tempting to treat all patients to a tolerance limit of MLD instead of a patient specific limit, which in the current study was the limit achievable for the standard homogeneous plans. This would increase the MLD for the majority of patients which in the current clinical practice has a MLD below such a tolerance limit. Lung toxicity is therefore expected to increase as part of such a dose escalation. The approach in this study demonstrates that dose escalation is possible without increasing MLD.
In the inhomogeneous plans it is needed to limit the dose to mediastinum in order to avoid hot-spots. Only few studies have focused on dose constraints on connective tissue and limited evidence for tolerance doses exists. Meijer et al. and van Elmpt et al. [Citation19,Citation20] used a tolerance dose of 76 Gy. In the current study this level was decreased to 74 Gy to ensure clinical applicability of the inhomogeneous plans. The main cost for the dose escalation in the current study was an increased maximum dose of approximately 4 Gy to the connective tissue in mediastinum.
This study shows that even for a homogeneous distribution of clonogenic cells it is beneficial to increase the dose to sub-volumes of the tumour. It is of course beneficial to have information of the actual distribution of the clonogenic cells, which to some extent is feasible by FDG-PET scans. There are therefore studies which boost PET active tumour volumes. However, even if the PET information is not truly indicative of the actual distribution of clonogenic cells, the current study shows that an increase in TCP is likely to occur anyway, due to the fact that more dose is deposited in the tumour.
For all 20 patients, both the homogeneous and inhomogeneous dose distributions have been re-calculated in 4D accounting for respiratory motion and simulated systematic and random uncertainties. Detailed discussion of the 4D study is outside the scope of this paper, but the results support a 10 percentage points TCP increase between homogeneous and inhomogeneous plans (data will be published in a future paper).
Conclusions
An inhomogeneous plan with higher mean tumour dose and predicted TCP than conventional homogeneous plans can be created for all NSCLC patients in this study, regardless of lymph node involvement, tumour stage, location, and size. With inhomogeneous dose distributions it is possible to increase the dose to the tumour without compromising the clinical toxicity levels. The conservative dose constraints used for the organs at risk ensures clinical applicable treatment plans that can be implemented today. Results from TCP estimates suggests a TCP gain in the range of 10–15 percentage points compared to standard homogeneous dose distributions without changing the expected lung toxicity.
Supplementary Figures 1 and 2
Download PDF (1.4 MB)Acknowledgements
This work is supported by the Region of Southern Denmark and by CIRRO – The Lundbeck Foundation Center for Interventional Research in Radiation Oncology and The Danish Council for Strategic Research.
Declaration of interest: The authors report no conflicts of interest. The authors alone are responsible for the content and writing of the paper.
References
- Aerts HJWL, van Baardwijk AAW, Petit SF , Offermann C, van Loon J, Houben R, et al. Identification of residual metabolic-active areas within individual NSCLC tumours using a pre-radiotherapy (18)Fluorodeoxyglucose-PET-CT scan. Radiother Oncol 2009;91:386–92.
- Abramyuk A, Tokalov S, Zöphel K, Koch A, Lazanyi KS, Gillham C, et al. Is pre-therapeutical FDG-PET/CT capable to detect high risk tumor subvolumes responsible for local failure in non-small cell lung cancer?. Radiother Oncol 2009;91:399–404.
- Lievens Y, Nulens A, Gaber MA, Defraene G, De Wever W, Stroobants S, et al. Intensity-modulated radiotherapy for locally advanced non-small-cell lung cancer: A dose-escalation planning study. Int J Radiat Oncol Biol Phys 2011;80:306–13.
- St-Hilaire J, Sévigny C, Beaulieu F, Germain F, Lavoie C, Dagnault A, et al. Dose escalation in the radiotherapy of non-small-cell lung cancer with aperture-based intensity modulation and photon beam energy optimization for non-preselected patients. Radiother Oncol 2009;91:342–8.
- Weiss E, Ramakrishnan V, Keall PJ. Is there a selection bias in radiotherapy dose-escalation protocols?. Int J Radiat Oncol Biol Phys 2007;68:1359–65.
- Belderbos JSA, Heemsbergen WD, De Jaeger K, Baas P, Lebesque JV. Final results of a Phase I/II dose escalation trial in non-small-cell lung cancer using three-dimensional conformal radiotherapy. Int J Radiat Oncol Biol Phys 2006;66:126–34.
- Kong FM, Ten Haken RK, Schipper MJ, Sullivan MA, Chen M, Lopez C, et al. High-dose radiation improved local tumor control and overall survival in patients with inoperable/unresectable non-small-cell lung cancer: Long-term results of a radiation dose escalation study. Int J Radiat Oncol Biol Phys 2005;63:324–33.
- ICRU Report 50. Prescribing, recording and reporting photon beam therapy. Technical report, Bethesda, MD: International Commission on Radiation Units and Measurements; 1993.
- Seppenwoolde Y, Lebesque JV, de Jaeger K, Belderbos JSA, Boersma LJ, Schilstra C, et al. Comparing different NTCP models that predict the incidence of radiation pneumonitis. Normal tissue complication probability. Int J Radiat Oncol Biol Phys 2003;55:724–35.
- Engelsman M, Remeijer P, van Herk M, Lebesque JV, Mijnheer BJ, Damen EM. Field size reduction enables iso-NTCP escalation of tumor control probability for irradiation of lung tumors. Int J Radiat Oncol Biol Phys 2001;51:1290–8.
- Baker M, Nielsen M, Hansen O, Jahn JW, Korreman S, Brink C. Isotoxic dose escalation in the treatment of lung cancer by means of heterogeneous dose distributions in the presence of respiratory motion. Int J Radiat Oncol Biol Phys 2011;81:849–55.
- Schwarz M, Alber M, Lebesque JV, Mijnheer BJ, Damen EMF. Dose heterogeneity in the target volume and intensity- modulated radiotherapy to escalate the dose in the treatment of non-small-cell lung cancer. Int J Radiat Oncol Biol Phys 2005;62:561–70.
- Gottlieb KL, Hansen CR, Hansen O, Westberg J, Brink C. Investigation of respiration induced intra- and inter- fractional tumour motion using a standard Cone Beam CT. Acta Oncol 2010;49:1192–8.
- Nielsen TB, Hansen VN, Westberg J, Hansen O, Brink C. A dual centre study of setup accuracy for thoracic patients based on Cone-Beam CT data. Radiother Oncol 2012;102:281–6.
- Schytte T, Hansen O, Stolberg-Rohr T, Brink C. Cardiac toxicity and radiation dose to the heart in definitive treated non-small cell lung cancer. Acta Oncol 2010;49:1058–60.
- Martel MK, Ten Haken RK, Hazuka MB, Kessler ML, Strawderman M, Turrisi AT, et al. Estimation of tumor control probability model parameters from 3-D dose distributions of non-small cell lung cancer patients. Lung Cancer 1999;24:31–7.
- Webb S, Nahum AE. A model for calculating tumour control probability in radiotherapy including the effects of inhomogeneous distributions of dose and clonogenic cell density. Phys Med Biol 1993;38:653–66.
- Webb S. Optimum parameters in a model for tumour control probability including interpatient heterogeneity. Phys Med Biol 1994;39:1895–914.
- Meijer G, Steenhuijsen J, Bal M, De Jaeger K, Schuring D, Theuws J. Dose painting by contours versus dose painting by numbers for stage II/III lung cancer: Practical implications of using a broad or sharp brush. Radiother Oncol 2011;100:396–401.
- van Elmpt W, De Ruysscher D, van der Salm A, Lakeman A, van der Stoep J, Emans D, et al. The PET-boost randomised phase II dose-escalation trial in non-small cell lung cancer. Radiother Oncol 2012;104:67–71.