Abstract
Backgound. Stereotactic radiotherapy for central lung tumors has a narrower therapeutic index than that for peripheral tumors. Tumor tracking strategies have been proposed to reduce treatment volumes and toxicity, however they need to consider uncertainties in tumor size and shape change throughout respiration to ensure optimal local control. We quantified these uncertainties and explored strategies to account for them. Material and methods. Ten patients with central tumors, PTV > 100 cm3, motion > 5 mm and a 10-phase 4DCT without significant artifact in the tumor region were evaluated. Uncertainties were quantified using GTV size in different phases, and the Hausdorff distance (HD) between the phase 50% GTV and other phases after soft-tissue rigid registration. An individualized internal target volume for tracking (ITVT) was generated from the union of the GTVs in all phases after rigid registration. This was compared to ITVs generated for tracking based on the phase 50% GTV alone or with isotropic margins of 3 or 5 mm for size and volume overlap. Results. Median free-breathing PTV size and motion were 162.1 cm3 (110–210) and 8.9 mm (6.1–14.1). Overall, median GTV size variation and HD were 4.7% (0.2–22.3) and 6.3 mm (3.9–17.6). Tracking using GTV 50% alone resulted in median volume overlap with ITVT of 71.7% (range 56.8–85.1). Isotropic margins of 3 or 5mm always resulted in a volume overlap less than 95% or a volume larger than the ITVT. Conclusions. Changes in size and shape of central lung tumors are substantial during respiration. These limit the ability to reduce treatment volumes with tracking, especially if isotropic margins are used. An individualized ITV for tracking, such as the ITVT is preferred.
Stereotactic ablative radiotherapy (SABR) is the preferred treatment for medically inoperable peripheral early-stage lung tumors [Citation1,Citation2]. In this setting, high rates of local control are achieved and toxicity is infrequent [Citation3,Citation4]. For central tumors there is an increased risk of high-grade toxicity [Citation5], and strategies to mitigate this, include reducing prescribed doses, accepting lower PTV coverage or reducing setup margins [Citation6–9], which may compromise local control.
SABR is widely planned using four-dimensional computed tomography (4DCT), from which it is common to delineate a free-breathing internal target volume (ITV) encompassing the gross tumor volume (GTV) in all breathing phases [Citation10,Citation11]. In addition to motion, this incorporates other uncertainties including variation in tumor size and shape during breathing [Citation12,Citation13]. Strategies that reduce treatment volumes such as tumor tracking [Citation14] may reduce ITV size and therefore the risk of toxicity [Citation15,Citation16]. However, reported experiences with tumor tracking typically generate plans using a GTV-defined from a single breathing phase, which is then considered the ITV for tumor tracking [Citation17–19]. This resultant ITV no longer accounts for changes in size and shape as a free-breathing ITV would. Instead, additional margins may or may not be added to the ITV for tumor tracking to account for these uncertainties. For large, central tumors, where tumor tracking may have the greatest therapeutic benefit, we quantified these variations in tumor size and shape and investigated different strategies to account for them.
Material and methods
Study population
The planning computed tomography (CT) scans from 10 patients with 1) central lung tumors, 2) a planning target volume (PTV) greater than 100 cm3, 3) tumor motion greater than 5 mm (3D displacement vector of the center of mass), and 4) 10-phase 4DCT visually free of gross artifact in the tumor region (as evaluated by two Radiation Oncologists), were assessed in this retrospective study. Four- dimensional CT images were acquired in uncoached free-breathing conditions without contrast, using a 2.5 mm slice thickness. The clinical free-breathing ITV (ITVFB) was defined by the union of the GTVs from all 10 breathing phases [Citation20]. The clinical PTV was generated using an isotropic 5 mm expansion of the ITVFB.
Quantifying variation in tumor size and shape during breathing
By consensus agreement, two Radiation Oncologists delineated the GTV in phase 50% of the planning 4DCT. Using deformable image registration (Velocity AI, version 2.7) this was propagated through the remaining phases and manually edited when required by the same observers [Citation21]. Subsequently, GTV size in each phase relative to phase 50% was determined. Next, after soft-tissue rigid registration of the GTV in each phase with the phase 50% GTV, the Hausdorff distance (HD) [Citation22] was determined. This assumed tracking could be achieved with the same accuracy as rigid registration of static images, thus more effectively enabling size and shape variation to be assessed independent of respiratory motion. The region of interest for rigid registration was the GTV with a margin of approximately 1 cm and included both translations and rotations.
Impact of variation in tumor size and shape on tracking volume
Soft-tissue rigid registration of the phase 50% GTV to other individual phases was used to assess coverage by the phase 50% and model the scenario of tracking the GTV 50% without accounting for changes in size and shape in other phases. The union of all GTVs after rigid registration with the phase 50% GTV was referred to as the individualized tracking ITV (ITVT). This tracking aperture was considered to account for variations in tumor size and shape during breathing. In addition, the following tracking strategies were also assessed, with respect to their size and volume overlap with ITVT. All analyses were carried out at the ITV level since we were interested in whether or not this structure adequately reflected variation in size and shape: 1) Phase 50% GTV alone [Citation17–19]; 2) Union of phase 50% and 0% GTVs after rigid registration (ITV0 + 50); 3) Phase 50% GTV + 3 mm isotropic margin [Citation12]; and 4) Phase 50% GTV + 5 mm isotropic margin.
Statistical considerations
Descriptive statistics were used for all baseline characteristics. Average size differences relative to phase 50% were based on absolute differences (i.e. all values were considered positive for this analysis). All statistical analyses were performed using Statistical Package for Social Sciences (version 18.0).
Results
Baseline tumor characteristics are summarized in . Representative axial slices of four cases illustrating the types of tumors studied are shown in . The median tumor diameter, PTV size and tumor motion were respectively, 52.5 mm (range 38–65), 162 cm3 (range 110–210) and 8.9 mm (range 6.1–14.1).
Figure 1. Representative axial slices (phase 50%) of four central tumors included in this study. Top left para-cardiac, top right hilar, bottom para-mediastinal (non-cardiac). The inner and outer outlines define the gross and planning tumor volumes, respectively.
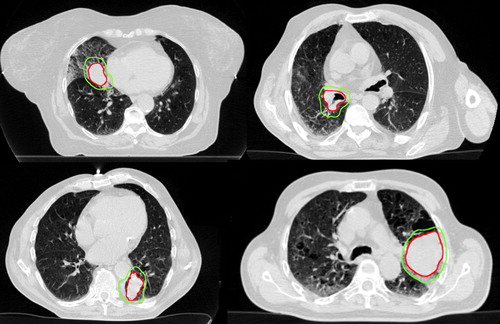
Table I. Baseline tumor characteristics.
Overall, GTV size varied a median of 4.7% (range 0.2–22.3) while the median maximal GTV size variation from phase 50% for individual patients was 12.5% (range 5.6–22.3). GTV size did not correlate with respiratory phase, and the volume of the phase 50% GTV could be the largest (patients 6 and 9) or the smallest (patients 3 and 5) (). Overall, the median HD relative to GTV 50% was 6.3 mm (range 3.9–17.6). The HD exceeded 5 mm for more than half the breathing cycle in all patients (). shows the phase 0% and 50% GTVs for patient 3, who had the greatest change in GTV size and shape with respiration.
Figure 2. Differences in gross tumor volume (GTV) size during breathing relative to phase 50% GTV (above), and the maximum Hausdorff distance between the GTV in each respiratory phase and the GTV 50% (below). Shown in color online.
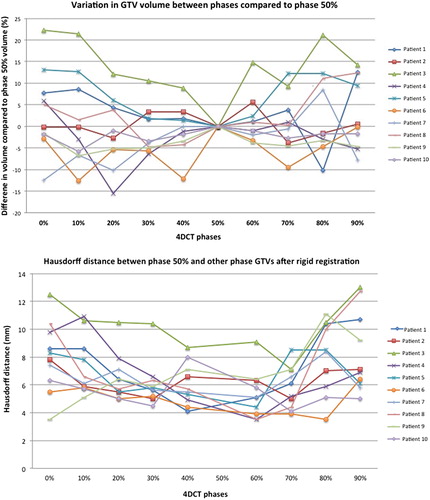
Figure 3. Illustration of significant variation in size and shape during breathing, with representative axial and coronal slices from patient 3 whose tumor showed the greatest change. CT images on left from phase 50% and on right from phase 0%. Contours from both phases are shown after rigid registration of the CT scans.
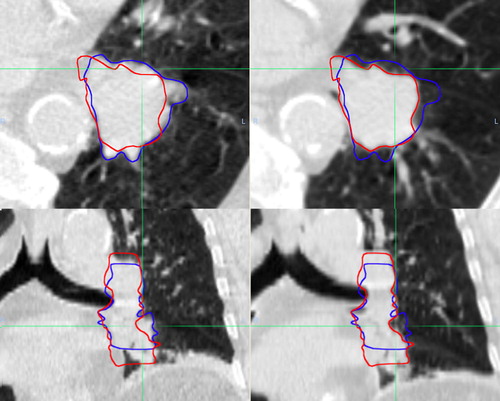
A tracking volume generated using the GTV 50% alone, resulted in a median volume overlap of ITVT of 71.7% (range 56.8–85.1). Using the union of GTV 50% and 0%, improved this to a median 82.1% (range 79.5–89.4). Tracking volumes generated using the phase 50% GTV with an isotropic margin of 3 or 5 mm, resulted in volume overlap of less than 95% or volume larger than ITVT (). ITVT was smaller than the ITVFB by a median 8% (range 2.1–21.2).
Table II. The impact of strategies to account for change in tumor size and shape during breathing.
Discussion
The importance of an adequate SABR dose for optimal local control has been confirmed in several studies [Citation5,Citation7,Citation8]. However, despite the use of respiratory tracking, concerns about toxicity when treating central tumors with SABR, have necessitated deliberate PTV under-dosing or dose reduction in a substantial proportion of patients, resulting in a measurable impact on local control [Citation6]. The findings in our study suggest that in some patients, marked variation in tumor size and shape may also influence local control and that trying to account fully for this would compromise the reduction in treatment volume that can be achieved with tracking. This suggests that tracking alone may not be an adequate solution for safer SABR delivery in very central tumors.
Our main finding was that overall, GTV size and shape varied by approximately 5% and more than 5 mm, respectively. We also observed that an ITV based on the GTV in a single respiratory phase or the extremes of respiration alone is generally inadequate to account for these variations. Applying an isotropic margin of 3 or 5 mm to the phase 50% GTV did not completely account for variations in size or shape, or it resulted in an ITV that was larger than ITVT. These results suggest that such variations limit the ability to reduce treatment volumes with tracking, particularly if isotropic margins are used to account for them. Despite this however, we found that the ITVT was smaller than the ITVFB by a median 8% confirming that accounting for size and shape variation with the ITVT when using a tracking strategy, may still offer gains over free breathing treatment.
Although there are methodological differences, our findings are consistent with previous reports. Wu et al. considered the extremes of breathing alone and found patients tumors > 80 cm3 (n = 3) all had a HD > 10 mm [Citation13]. Lu et al. found an additional 3 mm isotropic margin was required to account for variations in size and shape if coverage by the 95% prescribed isodoses was desired [Citation12]. However, this only considered the extremes of respiration and we observed that a 3 mm isotropic margin frequently generated an ITV larger than the ITVT. Using manual contours in all 4DCT phases, Persson et al. reported maximal GTV size variation was a median of 15.1% (range 2.3–90.8) [Citation23], while we observed a maximal variation of 12.5%.
We acknowledge that while tracking is the topic of interest in this study, gated delivery is more frequently employed in routine clinical practice as a strategy to minimize toxicity. Gating enables target volume reduction and accounts for changes in tumor size and shape with breathing, and the degree to which it might reduce toxicity depends on the relative location of the tumor and OAR during the gating phases.
A primary limitation of this study is the fact that our results are based on manual contours, which are subject to contouring uncertainties. We tried to limit these by having two clinicians contour by consensus and using deformable image registration to propagate contours over remaining phases, which has been shown to improve consistency [Citation21]. It is unclear how much of the total variation in size and shape during breathing we observed can be attributed to deformation or imaging artifact. However, we deliberately selected patients with no gross 4DCT artifact in the tumor region and variations in size and shape were not maximal in the mid-respiratory phases as would be expected if they were largely due to imaging artifacts [Citation11,Citation23]. We also acknowledge that changes in the size and shape of organs-at-risk, baseline shifts in tumor position and irregular breathing patterns impact tracking strategies, however a detailed analysis of the impact of these factors was beyond the scope of the current study for reasons including a lack of multiple 4DCT datasets. The HD is a very sensitive metric, as it uses pixel-level information representing the single largest of the shortest surface-to-surface distance measures between the GTV volumes being compared. Although it has been used by other authors [Citation13], the HD may overestimate the clinically relevant variation in size and shape, and the margin required to account for such changes. For this reason, size and overlap were also assessed. Additionally, the accuracy of rigid registration used to reflect the tracking may have influenced the HD results. Finally, a dosimetric analysis has not been performed to determine the clinical significance of these target volume differences.
The reduction in target volume using tumor tracking for large, central tumors appears to be limited by changes in tumor size and shape during breathing. In some patients, accounting for these with the ITVT, results in a smaller high-dose target volume than the ITVFB. Although using the ITVT, merits further investigation as one strategy to reduce the toxicity of central lung SABR without compromising local control, where underlying tumor-OAR geometry remains unfavorable its impact may be limited.
Declaration of interest statement: The VU University Medical Center has research collaborations with Varian Medical Systems, Brainlab AG and Velocity Medical Solutions. MD, BJS, and SUS have received honoraria and travel support from Varian Medical Systems. MD has received travel support from Brainlab AG. BJS has received honoraria and travel support from Brainlab AG. SAS declares no personal conflicts of interest.
References
- NCCN. Nccn clinical practice guildelines in oncology: Non-small cell lung cancer. Version 1.2013 . Available from: http://www.nccn.com. [cited 2012 Mar 1; 2013].
- Senan S. Surgery versus stereotactic radiotherapy for patients with early-stage non-small cell lung cancer: More data from observational studies and growing clinical equipoise. Cancer 2013;119:2668–70.
- Chi A, Liao Z, Nguyen NP, Xu J, Stea B, Komaki R. Systemic review of the patterns of failure following stereotactic body radiation therapy in early-stage non-small-cell lung cancer: Clinical implications. Radiother Oncol 2010; 94:1–11.
- Senthi S, Lagerwaard FJ, Haasbeek CJ, Slotman BJ, Senan S. Patterns of disease recurrence after stereotactic ablative radiotherapy for early stage non-small-cell lung cancer: A retrospective analysis. Lancet Oncol 2012;13:802–9.
- Senthi S, Haasbeek CJ, Slotman BJ, Senan S. Outcomes of stereotactic ablative radiotherapy for central lung tumours: A systematic review. Radiother Oncol 2013;106:276–82.
- Nuyttens JJ, van der Voort van Zyp NC, Praag J, Aluwini S, van Klaveren RJ, Verhoef C, et al. Outcome of four-dimensional stereotactic radiotherapy for centrally located lung tumors. Radiother Oncol 2012;102:383–7.
- Grills IS, Hope AJ, Guckenberger M, Kestin LL, Werner-Wasik M, Yan D, et al. A collaborative analysis of stereotactic lung radiotherapy outcomes for early-stage non-small-cell lung cancer using daily online cone-beam computed tomography image-guided radiotherapy. J Thorac Oncol 2012; 7:1382–93.
- Onishi H, Araki T, Shirato H, Nagata Y, Hiraoka M, Gomi K, et al. Stereotactic hypofractionated high-dose irradiation for stage I nonsmall cell lung carcinoma: Clinical outcomes in 245 subjects in a Japanese multiinstitutional study. Cancer 2004;101:1623–31.
- Waldeland E, Ramberg C, Arnesen MR, Helland A, Brustugun OT, Malinen E. Dosimetric impact of a frame-based strategy in stereotactic radiotherapy of lung tumors. Acta Oncol 2012;51:603–9.
- Hurkmans CW, Cuijpers JP, Lagerwaard FJ, Widder J, van der Heide UA, Schuring D, et al. Recommendations for implementing stereotactic radiotherapy in peripheral stage Ia non-small cell lung cancer: Report from the quality assurance working party of the randomised phase III Rosel study. Radiat Oncol 2009;4:1.
- Hurkmans CW, van Lieshout M, Schuring D, van Heumen MJ, Cuijpers JP, Lagerwaard FJ, et al. Quality assurance of 4D-CT scan techniques in multicenter phase III trial of surgery versus stereotactic radiotherapy (radiosurgery or surgery for operable early stage (stage 1a) non-small-cell lung cancer [Rosel] study). Int J Radiat Oncol Biol Phys 2011;80:918–27.
- Lu XQ, Shanmugham LN, Mahadevan A, Nedea E, Stevenson MA, Kaplan I, et al. Organ deformation and dose coverage in robotic respiratory-tracking radiotherapy. Int J Radiat Oncol Biol Phys 2008;71:281–9.
- Wu J, Lei P, Shekhar R, Li H, Suntharalingam M, D’Souza WD. Do tumors in the lung deform during normal respiration? An image registration investigation. Int J Radiat Oncol Biol Phys 2009;75:268–75.
- Keall PJ, Mageras GS, Balter JM, Emery RS, Forster KM, Jiang SB, et al. The management of respiratory motion in radiation oncology report of AAPM task group 76. Med Phys 2006;33:3874–900.
- Baker R, Han G, Sarangkasiri S, DeMarco M, Turke C, Stevens CW, et al. Clinical and dosimetric predictors of radiation pneumonitis in a large series of patients treated with stereotactic body radiation therapy to the lung. Int J Radiat Oncol Biol Phys 2013;85:190–5.
- Matsuo Y, Shibuya K, Nakamura M, Narabayashi M, Sakanaka K, Ueki N, et al. Dose-volume metrics associated with radiation pneumonitis after stereotactic body radiation therapy for lung cancer. Int J Radiat Oncol Biol Phys 2012;83:e545–9.
- Bibault JE, Prevost B, Dansin E, Mirabel X, Lacornerie T, Lartigau E. Image-guided robotic stereotactic radiation therapy with fiducial-free tumor tracking for lung cancer. Radiat Oncol 2012;7:102.
- Collins BT, Erickson K, Reichner CA, Collins SP, Gagnon GJ, Dieterich S, et al. Radical stereotactic radiosurgery with real-time tumor motion tracking in the treatment of small peripheral lung tumors. Radiat Oncol 2007;2:39.
- van der Voort van Zyp NC, Prevost JB, Hoogeman MS, Praag J, van der Holt B, Levendag PC, et al. Stereotactic radiotherapy with real-time tumor tracking for non-small cell lung cancer: Clinical outcome. Radiother Oncol 2009;91:296–300.
- Underberg RW, Lagerwaard FJ, Cuijpers JP, Slotman BJ, van Sornsen de Koste JR, Senan S. Four-dimensional CT scans for treatment planning in stereotactic radiotherapy for stage I lung cancer. Int J Radiat Oncol Biol Phys 2004;60:1283–90.
- van Dam IE, van Sornsen de Koste JR, Hanna GG, Muirhead R, Slotman BJ, Senan S. Improving target delineation on 4-dimensional CT scans in stage I NSCLC using a deformable registration tool. Radiother Oncol 2010;96:67–72.
- Sim DG, Kwon OK, Park RH. Object matching algorithms using robust hausdorff distance measures. IEEE Trans Image Process 1999;8:425–9.
- Persson GF, Nygaard DE, Brink C, Jahn JW, Munck AF Rosenschold P, Specht L, et al. Deviations in delineated GTV caused by artefacts in 4DCT. Radiother Oncol 2010;96:61–6.