Abstract
Background. Our aim was to define predictors of late radiation-induced lung injury (RILI) in Hodgkin's lymphoma (HL) survivors treated with bleomycin-containing chemotherapy and radiotherapy. Material and methods. Eighty consecutive patients treated with chemotherapy and subsequent supradiaphragmatic radiation therapy for HL were retrospectively reviewed for symptoms and/or radiological signs of RILI. Median patient age was 26 years (range 14–55). Left, right, and total lung dosimetric parameters along with clinical, disease, and treatment-related characteristics were analyzed. Multivariate logistic regression analyses were performed. A receiver operator characteristic (ROC) curve analysis was performed to find possible cutoff values dividing patients into high- and low-risk groups. Results. Seven of 80 (9%) patients had lung disease at baseline. Four of 80 (5%) had toxicity after chemotherapy and before the beginning of radiotherapy. These patients were excluded from further evaluation. At a median time of 10 months (range 9–18), 9/69 patients (13%) developed lung radiological changes on computed tomography (CT) after treatment. Four of nine patients were diagnosed RTOG grade ≥ 2. On multivariate analyses, left-lung V30 (p = 0.004, OR = 1.108 95% CI 1.033–1.189) and total-lung V30 (p = 0.009, OR = 1.146 95% CI 1.035–1.270) resulted to be predictors of lung CT changes with a cutoff value of 16% and 15%, respectively. When only symptomatic RILI was considered a left-lung V30 cutoff value of 32% was estimated. Conclusion. Bleomycin and RT may cause lung injury in a small, but significant fraction of HL patients. Left-lung V30 predicts the risk of developing asymptomatic or symptomatic RILI after sequential chemo-radiotherapy.
The gold standard treatment of early stage Hodgkin's lymphoma (HL) is sequential chemo-radiation therapy. A common side effect of both chemotherapy (CHT) and thoracic irradiation is lung toxicity. CHT regimens incorporate bleomycin, which may cause severe lung injuries ranging from hypersensitivity pneumonitis and bronchiolitis obliterans organizing pneumonia to acute interstitial pneumonia and progressive pulmonary fibrosis. Bleomycin-induced lung injury may occur at a rate in the range of 3–20% [Citation1]. Similarly, radiotherapy (RT) involving the thoracic region may determine radiation-induced lung injury (RILI). An extensive literature deals with factors capable of predicting early or late RILI, mainly severe radiation pneumonitis, for patients receiving RT for non-small cell lung cancer [Citation2–4]. Studies on the evaluation of dosimetric predictors for mild to moderate radiation-induced pulmonary toxicity in breast cancer are also available [Citation5,Citation6]. Compared with lung or breast cancer patients, HL patient population is generally characterized by lower age, better performance status, different smoking history in addition to different CHT regimens and lower radiation doses prescribed. All these factors lead to a low incidence (5–10%) of clinically significant symptomatic pneumonitis in HL cohorts [Citation2]. Limited data are available on dosimetric predictors for RILI in HL patients. Specific studies on RILI in HL patients are those by Koh et al. and by Fox et al. [Citation7,Citation8] focused on acute radiation pneumonitis, while Ng et al. [Citation9] evaluated pulmonary function decline in HL patients receiving combined modality therapy. However, bleomycin-containing CHT along with lower radiation doses inherent to HL radiation treatments may be responsible of late-phase subclinical RT-induced injury such as fibrosis with radiological lung density changes detectable by computed tomography (CT). Imaging appearance of radiation lung fibrosis may manifest itself as volume loss, consolidation and traction bronchiectasis and can be seen even without any prior radiation pneumonitis [Citation10]. Fibrosis, even if asymptomatic, may decrease lung compliance thus reducing the patient's reserve needed to deal with future cardiopulmonary stresses [Citation2]. Furthermore, it has been hypothesized that subclinical lung damage could be indirectly responsible of secondary cardiac damage [Citation11]. As a consequence, the knowledge of clinical and/or dosimetric factors predicting asymptomatic RILI is of great importance for HL patients characterized by high cure rates and prolonged survival. Recently, emerging radiation techniques for HL management [Citation12] considerably increase sparing of organs at risk and control over dose distribution, thus amplifying the clinical relevance of the determination of predictive factors to be used for an effective RT planning.
The aim of this study was to quantify the incidence of symptomatic and asymptomatic lung injuries and to identify clinical and dosimetric parameters that correlate with the risk of late RILI development in a cohort of 80 HL patients treated with bleomycin-containing CHT regimens and RT.
Material and methods
Patients and treatments
Clinical and dosimetric records of 80 consecutive patients with HL were retrospectively reviewed for late RILI events. All patients received CHT and subsequent supradiaphragmatic radiation therapy at our Radiation Oncology Department from November 2001 to October 2009. Median patient age was 26 years (range 14–55). Median follow-up was 80 months (range 53–135).
CHT consisted of 4–6 cycles either of VEBEP (vinblastine 6 mg/m2 i.v. day 1 and 15, etoposide 80 mg/m2 i.v. days 1–3 and 15–17, bleomycin 10 mg/m2 i.v. day 1 and 15, epidoxorubicin 40 mg/m2 i.v. day 1 and 15, and prednisone 40 mg/m2 p.o. days 1–5 and 15–19) or ABVD (doxorubicin 25 mg/m2, bleomycin 10 mg/m2, vinblastine 6 mg/m2, and dacarbazine 375 mg/m2, all drugs i.v. and administered day 1 and 15 every 28 days). Both CHT settings provide the same bleomycin dosage.
Clinical target volume (CTV) included the nodal sites involved at the time of diagnosis in patients with disease stage I–II [Citation13], and the residual disease and/or the bulky site in patients with disease stage III–IV. Planning target volume (PTV) included CTV plus a 1-cm margin. All patients were treated with full three-dimensional (3D) free-breathing CT-based radiation treatment planning as described in detail in previous publications [Citation13,Citation14]. Radiation therapy was administered using 6–20 MV photon beams from a linear accelerator with anteroposterior- posteroanterior fields. A total dose from 30 to 45 Gy was prescribed. Daily fraction size ranged between 1.5 Gy and 1.8 Gy. 3D conformal plans were generated using a commercial treatment planning system (XiO 4.64, Elekta CMS) with the multigrid superposition dose calculation algorithm (grid spacing 0.4 cm) for heterogeneous tissues.
For all patients, the left and right lung tissues (LL and RL) were retrospectively contoured by the same radiation oncologist following RTOG 1106 recommendations [Citation15]. The total lung (TL) taken as a single, paired organ was also considered.
Dosimetric parameters for lung structures were extracted from the cumulative dose-volume histograms (DVHs). Dosimetric parameters included: the dose to 2% and to 50% of the volume (D2%, D50%), and the percentage volume exceeding X Gy (Vx) in increment of 5 Gy. We corrected the dosimetric parameters for the different dose per fraction size using an α/β ratio of 4 for the lung [Citation16].
RILI evaluation
All patients have been monitored for lung disease/injury as part of clinical routine during CHT, after CHT before RT, and after RT. Baseline and follow-up evaluations consisted of history and physical examination along with periodic (every 6 months for the first 2 years, and yearly thereafter) total body CT scans. Follow-up visits were planned every 3 months for the first year, then every 6 months for the next 2 years, and once a year thereafter. The compliance of the patients to the follow-up schedule was good with all patients who had undergone at least five visits and two CT scans in the first 2 years.
Signs and symptoms of RILI were scored according to the RTOG late toxicity scoring system [Citation17] into the following groups:
Grade 1: Asymptomatic or mild symptoms (dry cough); slight radiographic appearances
Grade 2: Moderate symptomatic fibrosis or pneumonitis (severe cough); low grade fever; patchy radiographic appearances
Grade 3: Severe symptomatic fibrosis or pneumonitis; dense radiographic changes
Radiological lung density changes post-CHT-RT were evaluated on follow-up CT scans using the treatment planning CT as base-line comparison. All the CT scans were retrospectively reviewed by a radiologist. Time to RILI was computed from the beginning of treatment to the first symptom and/or radiological signs.
Any pretreatment lung disease (e.g. asthma), or any bleomycin-related lung toxicity along with comorbidities (preexisting cardiac abnormalities, hypertension, hypercholesterolemia and diabetes) as well as smoking history were recorded for each patient. Pretreatment elevated C-reactive protein (CRP) and lactate dehydrogenase (LDH) levels over normal limits were also recorded [Citation18,Citation19].
Statistical analysis
Statistical analysis was performed first for patients with symptoms, next for patients with radiological signs of RILI, thus including the former. Accordingly, the categories “symptomatic RILI” and “CT changes” were defined. Patient, disease and treatment-related characteristics were examined according to the development of “symptomatic RILI” or “CT changes” by univariate statistical methods: categorical variables were expressed as percentages and tested by Pearson's χ2-test or Fisher's exact test when appropriate; the median and the range were used to describe all continuous variables and Mann-Whitney U-test was employed for analyzing them.
Multivariate logistic regression analysis was performed on those parameters which resulted significant at the previous analyses. The logistic regression model is:
with
where m is the number of patients, x1, x2, …, xn represent different input variables and β0, β1, …, βn are the corresponding regression coefficients. For the selection of variables, a stepwise method (Wald-Enter method, p = 0.05 for entry into the model and p = 0.10 for removal from the model) was performed. A receiver operator characteristic (ROC) curve analysis was performed to find possible discrimination values dividing patients into high- and low-risk groups. The discrimination value on the ROC curve was determined by Youden’s J statistic. A non-parametric paired test (Wilcoxon signed rank test) was used to compare LL and RL dosimetric parameters. Statistical analysis was performed with SPSS 18.0 and MedCalc statistical software.
Results
Seven of 80 (9%) patients had some type of lung disease or CT appearance of radiological findings before CHT treatment while four of 73 (5%) had bleomycin-related lung toxicity after CHT and before beginning RT. These patients were excluded from further evaluations. Characteristics of the 69 patients eligible for analysis are shown in .
Table I. Patient, disease and treatment characteristics.
At a median time of 10 months, nine patients (13%) developed radiological changes on CT. The CT changes occurred in three patients at 9 months after treatment, in two patients at 10 months, in one at 11 months, in one at 15 months, and in two at 18 months. Four patients were symptomatic: one patient was diagnosed with severe symptomatic fibrosis showing dense radiographic changes (Grade 3), three cases developed grade 2 RILI (one slight radiological changes with severe cough and two moderate symptomatic fibrosis with patchy radiographic appearances). Five patients developed slight CT radiological changes without symptoms (Grade 1).
Complete descriptive statistics are reported in Supplementary and (available online at http//informahealthcare.com/doi/abs/10.3109/0284186X.2013.850739).
Table II. Results of the multivariate analyses for symptomatic radiation-induced lung injury (RILI) and CT changes.
Symptomatic RILI
Cases of grades 2 and 3 symptomatic RILI (four patients) were grouped together in the analyses and compared with asymptomatic patients. The univariate analysis didn't show significant differences in the distribution of clinical and disease factors between symptomatic and asymptomatic patients (Table SI). Results of univariate analysis for dosimetric parameters of LL, RL and TL are reported in Table SII. TL D2% and LL dosimetric parameters (D2%, V25 and V30) resulted significantly correlated to symptomatic RILI. Applying multivariate logistic regression to potential predictors, LL-V30 (p = 0.014, OR = 1.164 95% CI 1.031–1.312) resulted to be the only independent predictor of the risk of symptomatic RILI (). The result of ROC analyses () was a discrimination value of 32% (AUC = 0.87, 95% CI 0.76–0.94).
Figure 1. Receiver operating characteristic curve and histogram of outcome distribution for discrimination value definition: (a) LL-V30 for symptomatic RILI; (b) TL-V30 for radiological CT changes; (c) LL-V30 for radiological CT changes. The dotted line represents the discrimination value.
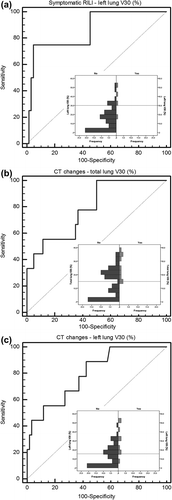
For LL-V30, shows the response curve calculated from Equation 1 and compared with the fraction of complications observed in the data grouped into quartiles.
Figure 2. Comparison of risk curves obtained by logistic regression model with the fraction of complications from the data grouped in quartiles for: (a) LL-V30 for symptomatic RILI (4 events over 69 patients), (b)–(c) TL-V30 and LL-V30 for radiological CT changes (nine events over 69 patients). The error bars are the standard deviation calculated from the data.
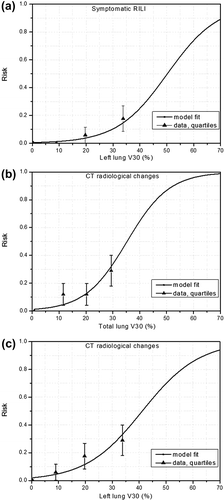
CT radiological density changes
When we considered all CT radiological density changes (i.e. any grade of RILI, nine patients) as outcome, the univariate analysis showed once again no significant difference in the distribution of clinical parameters between patients who developed CT changes and patients who did not (Table SI). Results of univariate analysis for dosimetric factors of LL, RL and TL are shown in Table SII. Dosimetric parameters for TL (D2%, V25 and V30) along with dosimetric parameters for LL (D50%, V05-V30) resulted significantly correlated to CT changes. Applying multivariate logistic regression to TL and LL potential predictors, TL-V30 (p = 0.009, OR = 1.146 95% CI 1.035–1.270) and LL-V30 (p = 0.004, OR = 1.108 95% CI 1.033–1.189) were the only independent predictors of CT changes, respectively. Results of multivariate logistic regression are reported in .
The results of ROC analyses ( and ) were a discrimination value of 15% (AUC = 0.79, 95% CI 0.68–0.88) for TL-V30 and of 16% (AUC = 0.80, 95% CI 0.69–0.89) for LL-V30.
For TL-V30 and LL-V30, and show the response curves calculated from Equation 1 and compared with the fraction of complications observed in the data grouped into quartiles.
In order to check if the obtained results on the LL were due to CTV localization and, consequently, to an asymmetric irradiation of the different lungs, we compared LL and RL dosimetric parameters. By analyzing either the entire cohort of patients or only the group of patients who developed CT changes no significant difference was found between LL versus RL dosimetric parameters. These results imply that both lungs were equally irradiated. Moreover, the spatial distribution of CT changes was uniformly distributed between left and right lungs.
Discussion
Bleomycin is an effective antineoplastic drug and it has been used successfully to treat a variety of malignancies, including HL. Nonetheless, bleomycin administration may result in lung inflammation that can progress to fibrosis. The mechanism of bleomycin-induced lung injury is not entirely clear, but it is likely to include components of oxidative damage, relative deficiency of the deactivating enzyme bleomycin hydrolase, and elaboration of inflammatory cytokines leading to endothelial damage of the lung vasculature [Citation20]. In early stage HL patients, bleomycin-containing polichemotherapy is sequentially administered before irradiation. RILI in HL is well documented [Citation7,Citation8]. Mediastinal irradiation can even further potentiate bleomycin-induced lung toxicity [Citation21]. However, a large fraction of patients experience sub-clinical RILI [Citation2].
Indeed, RILI generally manifests itself with two distinct clinical and pathologic phases: an early phase of transient inflammation and a later phase of asymptomatic chronic fibrosis. Pulmonary fibrosis is a consequence of the repair process by the injured tissue within the irradiated lung regions. It follows release of chemotactic factors for fibroblast including transforming growth factor-beta, fibronectin and platelet derived growth-factor. Patients who never experience symptomatic radiation pneumonitis may present with fibrosis some time later [Citation10]. Data on RILI have been mainly obtained from lung cancer settings and were often derived from patients with an in situ lung cancer [Citation2]. At the same time late effects such as pulmonary fibrosis have been less investigated despite the fact that the latter is important for long-term quality of life and could result in future respiratory insufficiency.
It has been suggested that it might be relevant to develop separate predictive models for RILI in patients with intact lungs and also explicitly analyze symptomatic, functional and radiographic endpoints separately [Citation2].
Volume of TL exceeding 20 and 30 Gy, along with mean lung dose (MLD), are well established predictors of RILI being V20 and MLD the most commonly reported predictors in the literature on lung and breast cancer. Dosimetric predictors in HL patients were less extensively studied. However, TL-V20 and MLD were also identified as predictive factors for acute radiation pneumonitis in HL patients in the studies by Koh et al. and by Fox et al. [Citation7,Citation8]. A V20 of 36% and a MLD of 14 Gy were found to be predictive of clinically significant pneumonitis (RTOG grade 2) by Koh et al. while slightly lower values (V20 of 33.5% and a MLD of 13.5 Gy) were obtained by Fox et al. for RTOG grade 1–3.
In our cohort, 5% of patients had lung toxicity after CHT and before the start of RT. This incidence is in agreement with that reported in literature [Citation1]. Furthermore, the incidence of lung toxicity in a series of HL patients treated at our institution with CHT alone was 5% (unpublished data).
At a median time of 10 months (range 9–18), we found an incidence of CT density changes of 13% (7% asymptomatic and 6% symptomatic). We separately analyzed symptomatic (grade ≥ 2) patients, and patients with radiological signs of RILI thus including the former patients. None of the clinical or CHT-related factors was associated with the considered outcomes. The LL-V30 emerged as the only independent dosimetric predictor of symptomatic RILI with a corresponding threshold value of 32% (AUC = 0.87). Interestingly, we found a trend (p = 0.08) for TL-V30 and a corresponding threshold value of 19% (AUC = 0.76, 95% CI 0.65–0.86) basically in agreement with the results of Hernando et al. [Citation2] who found a threshold of 18% for V30. Subsequently, we considered any CT radiological density changes as toxicity endpoint, thus taking into consideration symptomatic and asymptomatic patients. We found TL-V30 and LL-V30 to be both significant predictors with lower threshold values of 15% and of 16%, respectively.
These results suggest that the irradiation of LL might have a different influence on lung toxicity compared to the right lung. This statistical evidence requires additional studies to clarify the potential biological mechanism as well as its clinical meaning. However, we may hazard that the intrinsic difference between right and left lung (i.e. different numbers of lobes and size) could be a possible explanation for this finding. Remarkably, Wiegman et al. [Citation22] show in rats that LL irradiation has the most significant impact on lung toxicity evaluated using lung function and CT density as endpoints.
The lungs have been usually considered as a unique organ unit in dosimetric studies. Some authors, in lung or breast cancer patients’ studies, separately examined the effects of the radiation dose in relation to the ipsilateral and contralateral lung with respect to tumor location [Citation2,Citation5,Citation23]. In a cohort of breast cancer patients including mastectomized patients who received chest wall RT, Blom-Goldman et al. [Citation5] found a trend for the relation between ipsilateral lung V30 and density changes. Unfortunately, none of the above studies distinguished between left and right tumor position and consequently between right and left lung. The only study recording these data is that by Fay et al. [Citation24] who have found a trend between LL irradiation and the development of radiation pneumonitis. Moreover, it has been suggested that coirradiation of the heart may influence lung function due to the physiologic link between the lung and the cardiovascular system [Citation11,Citation25]. We can speculate that the significance for LL-V30, particularly stronger in symptomatic patients, may be in some way related to the irradiation of the heart.
It should be emphasized that our findings are the result of late pulmonary toxicity also evaluated by a high sensitive radiological method such as high- resolution CT which prevents the comparison with previous studies on lung toxicity in HL patients. In addition, we have taken into account the combined effect of bleomycin and radiation which prevents the comparison with data on RILI derived from patient settings other than HL. As with any retrospective analysis, our study had some limitations. For example, for most patients, we did not have data regarding pulmonary function. Furthermore, it is important to stress the relative small size of the analyzed cohort and a consequent low number of events. We need to continue to collect data in order to develop a more complete NTCP analysis.
In conclusion, sequential bleomycin-based CHT and radiation therapy may cause lung injury in a small, but significant fraction of HL patients. Through dosimetric analyses on lungs, also considered individually, we identified the LL as a critical volume and more precisely LL-V30 as a predictive factor of the risk of developing both asymptomatic and symptomatic late RILI. We defined threshold values for LL-V30 of 32% for symptomatic RILI and of 16% for CT radiological changes, respectively. The inclusion of these parameters in HL treatment plan optimization and evaluation may reduce the long-term effect of radiation to the lungs.
http://informahealthcare.com/doi/abs/10.3109/0284186X.2013.850739
Download PDF (615.3 KB)Declaration of interest: The authors report no conflicts of interest. The authors alone are responsible for the content and writing of the paper.
This work was partially supported by grants from the Italian Ministry for Education, University and Research (MIUR) in the framework of FIRB (RBFR10Q0PT_001 “DROPS” and RBNE08YFN3 “MERIT”).
References
- Froudarakis M, Hatzimichael E, Kyriazopoulou L, Lagos K, Pappas P, Tzakos AG, et al. Revisiting bleomycin from pathophysiology to safe clinical use. Crit Rev Oncol Hematol 2013;87:90–100.
- Marks LB, Bentzen SM, Deasy JO, Kong FM, Bradley JD, Vogelius IS, et al. Radiation dose-volume effects in the lung. Int J Radiat Oncol Biol Phys 2010;76: S70–6.
- Vogelius IR, Bentzen SM. A literature-based meta-analysis of clinical risk factors for development of radiation induced pneumonitis. Acta Oncol 2012;51:975–83.
- Dang J, Li G, Ma L, Diao R, Zang S, Han C, et al. Predictors of grade >/ = 2 and grade >/ = 3 radiation pneumonitis in patients with locally advanced non-small cell lung cancer treated with three-dimensional conformal radiotherapy. Acta Oncol 2013;52:1175–80.
- Blom-Goldman U, Svane G, Wennberg B, Lidestahl A, Lind PA. Quantitative assessment of lung density changes after 3-D radiotherapy for breast cancer. Acta Oncol 2007; 46:187–93.
- Kahan Z, Csenki M, Varga Z, Szil E, Cserhati A, Balogh A, et al. The risk of early and late lung sequelae after conformal radiotherapy in breast cancer patients. Int J Radiat Oncol Biol Phys 2007;68:673–81.
- Fox AM, Dosoretz AP, Mauch PM, Chen YH, Fisher DC, Lacasce AS, et al. Predictive factors for radiation pneumonitis in hodgkin lymphoma patients receiving combined-modality therapy. Int J Radiat Oncol Biol Phys 2012;83:277–83.
- Koh ES, Sun A, Tran TH, Tsang R, Pintilie M, Hodgson DC, et al. Clinical dose-volume histogram analysis in predicting radiation pneumonitis in Hodgkin’s lymphoma. Int J Radiat Oncol Biol Phys 2006;66:223–8.
- Ng AK, Li S, Neuberg D, Chi R, Fisher DC, Silver B, et al. A prospective study of pulmonary function in Hodgkin’s lymphoma patients. Ann Oncol 2008;19:1754–8.
- Wall RJ, Schnapp LM. Radiation pneumonitis. Respir Care 2006;51:1255–60.
- Ghobadi G, van der Veen S, Bartelds B, de Boer RA, Dickinson MG, de Jong JR, et al. Physiological interaction of heart and lung in thoracic irradiation. Int J Radiat Oncol Biol Phys 2012;84:e639–46.
- Maraldo MV, Brodin NP, Aznar MC, Vogelius IR, Munck Af Rosenschold P, Petersen PM, et al. Estimated risk of cardiovascular disease and secondary cancers with modern highly conformal radiotherapy for early-stage mediastinal Hodgkin lymphoma. Ann Oncol 2013;24: 2113–8.
- Cella L, Liuzzi R, Magliulo M, Conson M, Camera L, Salvatore M, et al. Radiotherapy of large target volumes in Hodgkin’s lymphoma: Normal tissue sparing capability of forward IMRT versus conventional techniques. Radiat Oncol 2010;5:33.
- Cella L, Conson M, Caterino M, De Rosa N, Liuzzi R, Picardi M, et al. Thyroid V30 predicts radiation-induced hypothyroidism in patients treated with sequential chemo-radiotherapy for Hodgkin’s lymphoma. Int J Radiat Oncol Biol Phys 2012;82:1802–8.
- Kong FM, Ritter T, Quint DJ, Senan S, Gaspar LE, Komaki RU, et al. Consideration of dose limits for organs at risk of thoracic radiotherapy: Atlas for lung, proximal bronchial tree, esophagus, spinal cord, ribs, and brachial plexus. Int J Radiat Oncol Biol Phys 2011;81:1442–57.
- Cella L, Liuzzi R, Conson M, Torre G, Caterino M, De Rosa N, et al. Dosimetric predictors of asymptomatic heart valvular dysfunction following mediastinal irradiation for Hodgkin’s lymphoma. Radiother Oncol 2011;101:316–21.
- Cox JD, Stetz J Pajak TF. Toxicity criteria of the Radiation Therapy Oncology Group (RTOG) and the European Organization for Research and Treatment of Cancer (EORTC). Int J Radiat Oncol Biol Phys 1995;31:1341–6.
- Matusiewicz SP, Williamson IJ, Sime PJ, Brown PH, Wenham PR, Crompton GK, et al. Plasma lactate dehydrogenase: A marker of disease activity in cryptogenic fibrosing alveolitis and extrinsic allergic alveolitis?Eur Respir J 1993; 6:1282–6.
- Rasmussen F, Mikkelsen D, Hancox RJ, Lambrechtsen J, Nybo M, Hansen HS, et al. High-sensitive C-reactive protein is associated with reduced lung function in young adults. Eur Respir J 2009;33:382–8.
- Sleijfer S. Bleomycin-induced pneumonitis. Chest 2001; 120:617–24.
- Hirsch A, Vander Els N, Straus DJ, Gomez EG, Leung D, Portlock CS, et al. Effect of ABVD chemotherapy with and without mantle or mediastinal irradiation on pulmonary function and symptoms in early-stage Hodgkin’s disease. J Clin Oncol 1996;14:1297–305.
- Wiegman EM, Meertens H, Konings AW, Kampinga HH Coppes RP. Loco-regional differences in pulmonary function and density after partial rat lung irradiation. Radiother Oncol 2003;69:11–9.
- Ramella S, Trodella L, Mineo TC, Pompeo E, Stimato G, Gaudino D, et al. Adding ipsilateral V20 and V30 to conventional dosimetric constraints predicts radiation pneumonitis in stage IIIA-B NSCLC treated with combined-modality therapy. Int J Radiat Oncol Biol Phys 2010;76:110–5.
- Fay M, Tan A, Fisher R, Mac Manus M, Wirth A Ball D. Dose-volume histogram analysis as predictor of radiation pneumonitis in primary lung cancer patients treated with radiotherapy. Int J Radiat Oncol Biol Phys 2005;61: 1355–63.
- Huang EX, Hope AJ, Lindsay PE, Trovo M, El Naqa I, Deasy JO, et al. Heart irradiation as a risk factor for radiation pneumonitis. Acta Oncol 2011;50:51–60.