Abstract
Background. Adjuvant chemoradiotherapy improves both overall- and relapse-free survival in patients with resected gastric cancer. However, this comes at the cost of increased treatment-related toxicity. Proton therapy (PT) has distinct dosimetric characteristics that may reduce dose to normal tissues, improving the therapeutic ratio. The purpose of this treatment planning study is to compare PT and intensity-modulated x-ray therapy (IMXT) in gastric cancer with regards to normal tissue sparing.
Material and methods. The patient population consisted of resected gastric cancer patients treated at a single institution between 2008 and 2013. Patients who had undergone 4D CT simulation were replanned to the originally delivered doses (45–54 Gy in 25–30 daily fractions) using six-field photon IMXT and 2–3-field PT (double scattering-uniform scanning techniques).
Results. Thirteen patients were eligible for the planning comparison. IMXT provided slightly higher homogeneity indices (median values 0.04 ± 0.01 vs. 0.07 ± 0.01, p = 0.03). PT resulted in significantly (p < 0.05) lower intermediate-low doses for all the normal tissues examined (small bowel V15 82 ml vs. 133 ml, liver mean doses Gy 11.9 vs. 14.4 Gy, left/right kidney mean doses 5/0.9 Gy vs. 7.8/3.1 Gy, heart mean doses 7.4 Gy vs. 9.5 Gy). The total energy deposited outside the target volume was significantly lower with PT (median integral dose 90.1 J vs. 129 J). Four patients were treated with PT: treatment was feasible and verifications scans showed that target coverage was robust.
Conclusion. PT can contribute to normal tissue sparing in the adjuvant treatment of gastric cancer, with a potential benefit in terms of compliance to treatment, acute and late toxicities.
Stomach cancer is the fourth most common neoplasm worldwide and the sixth in developed countries; as of 2008, it represents globally the second leading cause of death from cancer in both sexes [Citation1]. Surgery is the mainstay of cure for this disease; however, randomized phase III trials of adjuvant therapies have shown a significant impact on survival compared to surgery alone [Citation2,Citation3]. In the context of chemoradiation, the Intergroup 0116 study randomization to adjuvant chemoradiotherapy versus surgery alone was associated with improvements in median overall- and relapse-free survival [Citation2,Citation4].
Toxicity of chemoradiation is significant, as 17% of patients on the INT 0116 trial discontinued treatment, with 9% of patients receiving less than 40 Gy; 1% of patients died due to treatment-related complications. A non-significant increase in the occurrence of second malignancies was also reported in the chemoradiotherapy arm [Citation4]. Other studies have reported data on late toxicity, in particular progressive relative functional impairment of the left kidney, bowel obstruction/ perforation and anastomotic stricture [Citation5,Citation6].
The administration of radiotherapy for gastric cancer is complex due to: 1) large treatment volumes that include the postoperative bed and all draining lymph node regions at risk for tumor spread [Citation7]; and 2) numerous organs at risk (OAR), such as bowel, liver, kidneys, heart, and lungs with radiation tolerances well below the usual prescription dose (45–50 Gy).
Compared to the historical anteroposterior/ posteroanterior (AP/PA) field arrangement [Citation8], more advanced techniques such as three-dimensional conformal radiotherapy (3DCXT) and intensity-modulated radiation therapy (IMXT) have been shown to provide better target coverage and improved OAR sparing [Citation9,Citation10]. However, the volume of normal tissue which receives low doses is usually larger with IMXT, with possible concern for late effects and second malignancies [Citation11].
Proton therapy (PT) has unique dosimetric characteristics including a finite range in tissue, resulting in zero exit dose. The clinical literature using PT in gastric cancer is limited to case reports from University of Tsukuba, Japan [Citation12,Citation13]. No planning comparison studies have been published. However, it has been recently proposed that proton chemoradiation for upper GI cancers with comparable complexity, such as esophageal and pancreatic cancer, could help spare OARs, thereby decreasing acute toxicity, improving compliance, and lowering the rate of late complications in long-term survivors [Citation14,Citation15]. The aim of the present work was to evaluate the potential dosimetric benefit of PT in comparison with advanced photon radiotherapy in the multimodal treatment of gastric cancer.
Material and methods
Inclusion criteria
The internal electronic database at a single institution was reviewed for this planning comparison study with the authorization of the Institutional Review Board. Eligible patients included those who were treated with curative resection and adjuvant radiotherapy for gastric cancer, and had undergone a 4D simulation computed tomography (CT) for target motion evaluation. Patients with gastroesophageal junction tumors were excluded, as were patients treated with preoperative or palliative radiotherapy.
Patient population
Fourteen patients treated between August 2008 and February 2013 were considered eligible for the study. One patient was excluded after a preliminary planning evaluation revealed an extent of target volume exceeding the maximum allowed size for the compensator (15.2 cm thickness); therefore, a total of 13 patients were analyzed.
Supplementary Table I (available online at http://informahealthcare.com/doi/abs/10.3109/0284186X.2014.912351) summarizes the patient characteristics. Eleven patients (85%) had node positive disease. The majority of patients underwent partial gastrectomy and D2 lymphadenectomy. The actual prescription dose was 45 Gy in 25 fractions for 10 patients; three patients received an additional boost of 9 Gy in 5 fractions (total dose 54 Gy) to high-risk volumes. The median volume of PTV prescribed 45 Gy was 752 ml (range 198–963, interquartile range 242 ml).
Volume contouring review
Target volume and OARs were contoured according to the prospective protocol for treatment of upper gastrointestinal malignancies with proton chemoradiation. Internationally renowned guidelines for target delineation were followed [Citation7]. For the purpose of this study, the small bowel was contoured as tight loops rather than a peritoneal structure, 2 cm below the inferior extent of the clinical target volume (CTV) as per RTOG 0848 recommendations. The internal target volume (ITV) was the volume encompassing the CTV in addition to internal margin, as determined by the boolean function “OR” of the CTVs. at maximum inspiration and expiration. The maximum allowable target motion was 1 cm in order to qualify for proton treatment.
Treatment planning
Regardless of the actual (PT, 3D conformal or IMXT) treatment technique clinically delivered, all eligible cases were replanned to the originally delivered doses using PT and IMXT as detailed below.
A 2–3-field arrangement was chosen for PT plans. Posterior-lateral beam directions were preferred; anterior beam entrances were used when required by target coverage and/or OAR sparing, especially when the target was located anteriorly and extended superficially. The same approach was followed for the three cone down plans covering postoperative residual disease volumes: a posterior-lateral beam arrangement was adopted in two cases, while a right anterior/posterior arrangement was chosen for a target located close to the patient's anterior surface.
Plans were calculated using the Eclipse Proton Convolution Superposition algorithm with the double scattered or uniform scanning beam system from Ion Beam Application (IBA) proton therapy system. Choice of treatment modality depended on location and size of the target. The gantry is equipped with a multi-leaf collimator (MLC) which determines the allowable treatment size. Double scattering is capable of delivering protons with a 28 cm range and a maximum radius of 12 cm. For targets exceeding this limit we used uniform scanning, which has a 30 cm range and 18 × 25 cm field size.
Compensators and MLCs were used to conform the dose to the target distally and laterally. The MLC margin for the passive scattering proton plans was set from 1.2 cm to 1.6 cm beyond the ITV on a case-by-case basis in order to achieve the target coverage laterally, accounting for internal margin, setup margin, and the 90–50% penumbra. Target and compensator margins were made following a formalism common in modern proton therapy centers [Citation16]. Proximal margins (PM) and distal margins (DM) were set based on the uncertainty in proton range to the distal and proximal side of the CTV according to the following:
The smearing margin (SM) (or Bolus expansion in the Moyers formalism) was set according to:
In order to eliminate range uncertainties due to bowel gas movement, bowel gas was contoured on the original average CT set and Hounsfield Units were overridden.
IMXT plans were generated using 6 MV beam energies with six coplanar and non-coplanar fields using the PTV, a 5 mm uniform margin from the ITV, as the target volume. Beam angles were optimized for each patient. When feasible, the posterior direction in the beam arrangement was avoided for the purpose of minimizing kidney dose. Photon doses were calculated using the Eclipse Analytical Anisotropic Algorithm (AAA). OAR constraints for plan optimization are described in Supplementary Table II (available online at http://informahealthcare.com/doi/abs/10.3109/0284186X.2014.912351).
Data analysis
Dose volume histograms (DVH) were generated for target coverage and OAR sparing comparison between IMXT and PT. The photon PTV was used for both IMXT and PT evaluation.
In order to perform a fair comparison in OAR sparing between IMXT and PT plans, all the plans were normalized to administer 95% prescription dose to 98% of the PTV.
Homogeneity index was defined as follows: (D2-D98)PTV/D50PTV, where D2, D98 and D50 represent the dose delivered to the 2%, 98% and 50% of the target volume, respectively.
The OARs evaluated and the parameters collected and compared between PT and IMXT are described in Supplementary Table III (available online at http://informahealthcare.com/doi/abs/10.3109/0284186X.2014.912351). The integral dose (ID) was calculated as the product of the mean dose in Gray and the mass in kilograms of the “Whole Body minus PTV” structure. A uniform density of 1 g/cm3 was assumed [Citation17].
Mean, median, and standard deviation values were collected for all data points.
Statistical analysis was performed using a Wilcoxon pairwise signed-rank test. A p ≤ 0.05 was considered statistically significant.
Results
Plan quality
IMXT plans for initial volumes to 45 Gy were slightly more homogenous than PT plans (median values 0.04 ± 0.01 vs. 0.07 ± 0.01, p < 0.05). Cone down plans were almost identically homogeneous (Supplementary Table IV, available online at http://informahealthcare.com/doi/abs/10.3109/0284186X.2014.912351). An example of dose distributions of both techniques for an anterior target is shown in . The typical proton beam arrangements are described in Supplementary (available online at http://informahealthcare.com/doi/abs/10.3109/0284186X.2014.912351).
OAR sparing
Supplementary Table V (available online at http://informahealthcare.com/doi/abs/10.3109/0284186X.2014.912351) summarizes the comparison between IMXT and PT with regard to small bowel dose. A slight but significant decrease in maximum dose was achieved with IMXT. PT significantly reduced the volume of small bowel receiving doses in the medium-to-low range. For example, the median V15 with IMXT was 133 ml versus 82 ml with proton therapy, p = 0.002 (). Small bowel V15 was below 120 ml in 8/13 patients with PT and in 5/13 with IMXT.
Figure 2. Evaluation of differences in a) small bowel V15-25, b) mean dose to various organs (liver, kidneys, heart), and c) integral dose with IMXT and PT.
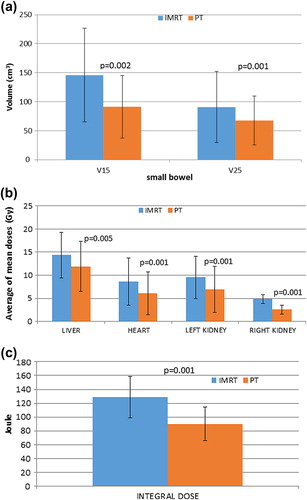
Large bowel maximum doses were slightly higher with PT (median D1 45.5 Gy vs. 46.1 Gy, p < 0.05). Large bowel D50 was significantly lower with PT (median values 10.7 Gy vs. 22.1 Gy, p < 0.05).
Liver D50 and mean doses () were significantly lower with PT compared with IMXT (median values 7.3 Gy vs. 0 Gy, p = 0.001 and 14.4 Gy vs. 11.9 Gy, p = 0.005, respectively). Values of V30 did not differ significantly between the two modalities (median values 17% IMXT vs. 20% PT, p = NS).
Supplementary Table VI (available online at http://informahealthcare.com/doi/abs/10.3109/0284186X.2014.912351) summarizes the median dose values received by the kidneys. Mean doses and D50 for both kidneys were significantly lower with PT; IMXT provided lower maximum doses for both kidneys, with a significant gain for the left kidney.
The heart mean doses () were significantly lower with PT compared with IMXT (median values 7.4 vs. 9.5, p = 0.001). Individual mean doses were < 2 Gy and > 10 Gy in one and six IMXT patients and in four and two PT patients, respectively.
Maximum doses to the spinal cord were slightly higher with IMXT (median values 26.2 Gy vs. 23.1 Gy, p = 0.02).
The total dose deposited in tissues outside the PTV (calculated as mentioned above) was significantly lower with PT compared with IMXT (, median values 129 J vs. 90.1 J, p = 0.001).
OAR sparing for the three cases receiving 54 Gy showed a similar trend for all OARs examined (Supplementary Table VI available online at http://informahealthcare.com/doi/abs/10.3109/0284186X.2014.912351).
Robustness analysis
Four patients (31% of the patient population) were actually treated with PT (Supplementary Table I available online at http://informahealthcare.com/doi/abs/10.3109/0284186X.2014.912351). In order to evaluate robustness of target coverage during treatment, three patients underwent two verification CT scans, while one patient underwent one verification CT scan (). All verification scans reported target coverage within ± 2% of the simulation CT (Supplementary Table VII, available online at http://informahealthcare.com/doi/abs/10.3109/0284186X.2014.912351), showing consistency between planned and delivered doses and a robust proton beam arrangement.
Discussion
The effectiveness of chemoradiation in gastric cancer has been demonstrated in phase III randomized trials [Citation2,Citation18], meta-analyses [Citation19,Citation20], and also from population-based studies evaluating large databases (> 4000 patients) [Citation21]. Its relative benefit over chemotherapy alone is probably greatest in node-positive patients [Citation18,Citation21].
Adjuvant chemoradiation is associated with significant treatment-related complications, and there are reasons to investigate PT as a means of reducing this considerable toxicity. Moreover, the International Commission on Radiation Units and Measurements report (ICRU 78) suggests that “the dose-sparing possible with protons is likely to be most valuable for large target volumes for which sparing the remaining volume is likely to be particularly valuable” [Citation22]. In this context, gastric cancer represents a promising disease setting for PT. Indeed, a Swedish expert opinion-based analysis on the need of PT across tumor sites [Citation23] concluded that all radically resected gastric patients below 75 years of age could enter a controlled study involving PT [Citation24]. The need to undertake model studies was also suggested. In light of this, we conducted the present work to compare dose distributions achieved with PT with those attained with advanced x-ray techniques, such as IMXT.
Our data demonstrate the superiority of PT over IMXT in the medium-low dose range for all OARs analyzed. The potential clinical benefit of these findings is yet to be determined. Gastrointestinal toxicity is the most common non-hematological toxicity experienced during adjuvant chemoradiation for gastric cancer, with 10–34% of patients experiencing ≥ G3 acute toxicity [Citation2,Citation25,Citation26]. Furthermore, the most common clinically apparent late complications following adjuvant chemoradiation for gastric cancer are bowel obstruction and perforation, and anastomotic stricture [Citation6,Citation27]. The small bowel is the main dose-limiting organ in the abdomen. The Quantitative Analyses of Normal Tissue effect in the Clinic (QUANTEC) recommended the absolute volume of intestinal loops receiving ≥ 15 Gy should be held under 120 ml [Citation28]. In the present analysis, in the group of patients planned up to 45 Gy, small bowel V15 was below 120 ml in 8/13 patients with PT and in 5/13 with IMXT. Moreover, for the three patients planned to a total dose = 54 Gy, small bowel mean V15 was 107 ml with PT, while it exceeded threshold dose with IMXT (Supplementary Table IV available online at http://informahealthcare.com/doi/abs/10.3109/0284186X.2014.912351). A clinical advantage for PT in reducing both acute and late small bowel toxicity could potentially be expected.
Radiation-induced kidney injury is typically divided in subclinical alterations, which can occur in the subacute period after treatment (3–18 months), and clinical symptoms in which latency can be as long as 19 years [Citation29]. The work of Jansen et al. [Citation5], which analyzed pre- and post-chemoradiation renograms and creatinine levels in a series of 44 gastric cancer patients, showed a significant correlation between dose-volume parameters (mean dose and V20) and progressive impairment of left kidney function with clinical manifestation (renovascular hypertension) in one patient followed for more than 18 months. A threshold value of 64% and 66% was found for left kidney V20 and mean dose, respectively. Previous reports [Citation30] demonstrated IMXT could reduce left kidney dose by > 50% compared with conventional 3D planning, while both techniques achieved the same sparing for the right kidney.
In our analysis, both IMXT and PT were able to deliver very low doses to the left kidney, with values well below the thresholds reported in the literature (Supplementary Table VI available online at http://informahealthcare.com/doi/abs/10.3109/0284186X.2014.912351). Similarly, the right kidney was adequately spared by both techniques, with PT achieving an almost complete avoidance of the organ (median doses < 1 Gy).
In our series, PT showed a slight but statistically significant advantage over IMXT in liver and heart mean doses. The mean liver doses were adequately below the limits commonly reported in literature for both techniques [Citation31]; consequently, the likelihood of realizing a clinical gain from the dosimetric superiority of PT is low.
Conversely, the dosimetric advantage of PT over IMXT in heart dose could have a clinical impact. Darby et al. [Citation32] have shown that the rate of coronary events in breast cancer patients increases linearly with the mean heart dose by 7.4% per Gy, starting within the first five years after exposure, and with no apparent threshold. Moreover, the absolute radiation related risk was found to be greater in patients with preexisting cardiac risk factors than for other women. In our analysis, the average mean heart dose for IMXT was 9.5 Gy and 7.4 Gy for PT, both within the range where one may expect to see clinically significant coronary events with adequate follow-up. The 10 year follow-up update analysis of the INT 0116 study did not mention any relevant late cardiotoxicity; however, details of non-lethal toxicities, such as hypertension and coronary atherosclerosis were not available for analysis.
Second malignancies are always a concern with radiotherapy; and there was a suggestion of increased second malignancies in the chemoradiation arm of INT 0116 [Citation4]. As expected, PT resulted in a lower total integral dose, which may reduce the likelihood of second malignancies. Of note, all PT plans analyzed in this study employed passive scattering or uniform scanning techniques, whose associated neutron production may or may not increase the risk of secondary cancer [Citation33–35]. In a recently published work by Chung et al. [Citation36], which matched data of 558 proton patients treated with passive techniques at the Harvard Cyclotron in Cambridge between 1973 and 2001 with 558 photon patients retrieved from the Surveillance, Epidemiology, and End Results (SEER) Program Cancer Registry, a lower incidence of second cancer was registered in the proton patient group compared with patients treated with x-ray therapy.
The present analysis has several limitations. Firstly, the planning robustness of the two techniques, intended as the offset between the planned and the delivered dose distribution, was not exhaustively evaluated. In the context of upper abdominal targets, the complex interaction between set-up errors, organ motion and variations in bowel filling along the beam path could result in a degradation of quality between planned dose and delivered dose. The potential impact of such variations is greater in PT than in photon therapy (IMXT), due to the finite range of protons [Citation37]. However, it is noteworthy that four of the 13 analyzed patients were actually treated with PT in a prospective study. To our knowledge, no other experiences of adjuvant PT for gastric cancer have been reported. In these patients, verification CT scans during treatment allowed verification that plans were robust between the planned and delivered dose distributions.
Adequate beam selection is crucial in proton therapy; in the present work, the beam arrangement (two-field with posterior-anterior and lateral oblique directions) was chosen to allow the most homogenous beam path in most patients. In some cases, however, a direct anterior beam was needed due to the limitation of compensator thickness to cover the targets laterally; the possible dosimetric and clinical disadvantages [Citation15] of this arrangement should be carefully evaluated and robustness must be evaluated with at least weekly verification CT scans.
Passive scattering PT resulted in a slight (less than 2%) increase in maximum doses to small bowel compared with IMXT; plan acceptability was not compromised and no clinical implications resulted for the four patients treated with protons. However, physicians should be aware of this limitation of passive scattering technique and a careful plan evaluation should be performed, especially when higher doses (≥ 54 Gy) in the range of normal tissue maximum dose limits are prescribed. The use of active beam scanning delivery technique could theoretically further improve dose distributions compared with passive techniques (especially conformality in the high dose regions). It was not employed in the present study because it represents a relatively new technique which is much more sensitive to motion than passive methods. The clinical implementation of active scanning technique in upper abdominal targets needs the validation of special tools currently under development, such as: 1) robustness optimization and evaluation software [Citation38]; and 2) respiratory motion management [Citation39].
Finally, our analysis was limited to the postoperative setting following the INT 0116 approach. However, an effective preoperative approach could enhance the rates of RO resections, reduce local recurrences and achieve a substantial rate of pathological complete response [Citation40]. The value of preoperative chemoradiation in resectable gastric cancer is currently being tested in an ongoing international randomized phase III trial (TOPGEAR, NCT01924819). As of PT, it could be hypothesized that its administration in the preoperative setting could reduce both treatment-related and postoperative complications, as already shown in the neoadjuvant approach for esophageal cancer [Citation41]. A strict protocol to reduce organ filling variations along with respiratory management would be mandatory to ensure adequate reproducibility of target position.
In conclusion, a dosimetric advantage over advanced x-ray therapy in the range of medium and low doses was demonstrated with the use of PT in resected gastric cancer. A patient-specific, head-to-head planning comparison could help in the optimal selection of patients to be considered for PT. The potential clinical advantages of PT with regards to treatment compliance and minimization of acute and late toxicity, justifies further study. The development of robustness tools (i.e. protocols for reimaging and plan adaptation during treatment) to ensure the delivery of high quality plans is essential.
Supplementary Figure 1 and Supplementary Tables I–VII
Download PDF (1.2 MB)Acknowledgments
We thank the reviewers’ work, which contributed to improve the quality of the manuscript.
Declaration of interest: The authors report no conflicts of interest. The authors alone are responsible for the content and writing of the paper.
References
- Ferlay J, Shin H-R, Bray F, Forman D, Mathers C, Parkin DM. Estimates of worldwide burden of cancer in 2008: GLOBOCAN 2008. Int J Cancer 2010;127: 2893–917.
- Macdonald JS, Smalley SR, Benedetti J, Hundahl SA, Estes NC, Stemmermann GN, et al. Chemoradiotherapy after surgery compared with surgery alone for adenocarcinoma of the stomach or gastroesophageal junction. N Engl J Med 2001;345:725–30.
- Cunningham D, Allum WH, Stenning SP, Thompson JN, Van de Velde CJH, Nicolson M, et al. Perioperative chemotherapy versus surgery alone for resectable gastroesophageal cancer. N Engl J Med 2006;355:11–20.
- Smalley SR, Benedetti JK, Haller DG, Hundahl SA, Estes NC, Ajani JA, et al. Updated analysis of SWOG- directed intergroup study 0116: a phase III trial of adjuvant radiochemotherapy versus observation after curative gastric cancer resection. J Clin Oncol 2012;30:2327–33.
- Jansen EPM, Saunders MP, Boot H, Oppedijk V, Dubbelman R, Porritt B, et al. Prospective study on late renal toxicity following postoperative chemoradiotherapy in gastric cancer. Int J Radiat Oncol Biol Phys 2007;67:781–5.
- Wysocka B, Kassam Z, Lockwood G, Brierley J, Dawson L, Ringash J. Late toxicity after adjuvant radiochemotherapy for gastric adenocarcinoma. J Clin Oncol 2006;24:s14042.
- Tepper JE, Gunderson LL. Radiation treatment parameters in the adjuvant postoperative therapy of gastric cancer. Semin Radiat Oncol 2002;12:187–95.
- Smalley SR, Gunderson L, Tepper J, Martenson JA Jr, Minsky B, Willett C, et al. Gastric surgical adjuvant radiotherapy consensus report: Rationale and treatment implementation. Int J Radiat Oncol Biol Phys 2002;52:283–93.
- Leong T, Willis D, Joon DL, Condron S, Hui A, Ngan SYK. 3D conformal radiotherapy for gastric cancer – results of a comparative planning study. Radiother Oncol 2005;74: 301–6.
- Milano MT, Garofalo MC, Chmura SJ, Farrey K, Rash C, Heimann R, et al. Intensity-modulated radiation therapy in the treatment of gastric cancer: Early clinical outcome and dosimetric comparison with conventional techniques. Br J Radiol 2006;79:497–503.
- Dahele M, Skinner M, Schultz B, Cardoso M, Bell C, Ung YC. Adjuvant radiotherapy for gastric cancer: A dosimetric comparison of 3-dimensional conformal radiotherapy, tomotherapy and conventional intensity modulated radiotherapy treatment plans. Med Dosim 2010;35:115–21.
- Shibuya S, Takase Y, Aoyagi H, Orii K, Sharma N, Tsujii H, et al. Definitive proton beam radiation therapy for inoperable gastric cancer: A report of two cases. Radiat Med 1991;9: 35–40.
- Koyama S, Kawanishi N, Fukutomi H, Osuga T, Iijima T, Tsujii H, et al. Advanced carcinoma of the stomach treated with definitive proton therapy. Am J Gastroenterol 1990; 85:443–7.
- Lin SH, Komaki R, Liao Z, Wei C, Myles B, Guo X, et al. Proton beam therapy and concurrent chemotherapy for esophageal cancer. Int J Radiat Oncol Biol Phys 2012;83: e345–51.
- Nichols RC Jr, George TJ, Zaiden RA Jr, Awad ZT, Asbun HJ, Huh S, et al. Proton therapy with concomitant capecitabine for pancreatic and ampullary cancers is associated with a low incidence of gastrointestinal toxicity. Acta Oncol 2013;52: 498–505.
- Moyers MF, Miller DW, Bush DA, Slater JD. Methodologies and tools for proton beam design for lung tumors. Int J Radiat Oncol Biol Phys 2001;49:1429–38.
- Eldebawy E, Parker W, Abdel Rahman W, Freeman CR. Dosimetric study of current treatment options for radiotherapy in retinoblastoma. Int J Radiat Oncol Biol Phys 2012; 82:e501–5.
- Lee J, Lim DH, Kim S, Park SH, Park JO, Park YS, et al. Phase III trial comparing capecitabine plus cisplatin versus capecitabine plus cisplatin with concurrent capecitabine radiotherapy in completely resected gastric cancer with D2 lymph node dissection: The ARTIST trial. J Clin Oncol 2012;30:268–73.
- Valentini V, Cellini F, Minsky BD, Mattiucci GC, Balducci M, D’Agostino G, et al. Survival after radiotherapy in gastric cancer: Systematic review and meta-analysis. Radiother Oncol 2009;92:176–83.
- Ohri N, Garg MK, Aparo S, Kaubisch A, Tome W, Kennedy TJ, et al. Who benefits from adjuvant radiation therapy for gastric cancer?A meta-analysis. Int J Radiat Oncol Biol Phys 2013;86:330–5.
- Coburn NG, Govindarajan A, Law CHL, Guller U, Kiss A, Ringash J, et al. Stage-specific effect of adjuvant therapy following gastric cancer resection: A population-based analysis of 4,041 patients. Ann Surg Oncol 2008;15:500–7.
- ICRU Report No. 78. Treatment planning. J ICRU 2007;7:95–122.
- Glimelius B, Ask A, Bjelkengren G, Björk-Eriksson T, Blomquist E, Johansson B, et al. Number of patients potentially eligible for proton therapy. Acta Oncol 2005; 44:836–49.
- Ask A, Johansson B, Glimelius B. The potential of proton beam radiation therapy in gastrointestinal cancer. Acta Oncol 2005;44:896–903.
- Kassam Z, Lockwood G, O’Brien C, Brierley J, Swallow C, Oza A, et al. Conformal radiotherapy in the adjuvant treatment of gastric cancer: Review of 82 cases. Int J Radiat Oncol Biol Phys 2006;65:713–9.
- Kundel Y, Purim O, Idelevich E, Lavrenkov K, Man S, Kovel S, et al. Postoperative chemoradiation for resected gastric cancer – is the Macdonald Regimen Tolerable? A retrospective multi-institutional study.Radiat Oncol 2011; 6:127.
- Boda-Heggemann J, Weiss C, Schneider V, Hofheinz R-D, Haneder S, Michaely H, et al. Adjuvant IMRT/XELOX radiochemotherapy improves long-term overall- and disease-free survival in advanced gastric cancer. Strahlenther Onkol 2013;189:417–23.
- Kavanagh BD, Pan CC, Dawson LA, Das SK, Li XA, Ten Haken RK, et al. Radiation dose-volume effects in the stomach and small bowel. Int J Radiat Oncol Biol Phys 2010;76:S101–7.
- Dawson LA, Kavanagh BD, Paulino AC, Das SK, Miften M, Li XA, et al. Radiation-associated kidney injury. Int J Radiat Oncol Biol Phys 2010;76:S108–15.
- Wieland P, Dobler B, Mai S, Hermann B, Tiefenbacher U, Steil V, et al. IMRT for postoperative treatment of gastric cancer: Covering large target volumes in the upper abdomen: A comparison of a step-and-shoot and an arc therapy approach. Int J Radiat Oncol Biol Phys 2004;59:1236–44.
- Pan CC, Kavanagh BD, Dawson LA, Li XA, Das SK, Miften M, et al. Radiation-associated liver injury. Int J Radiat Oncol Biol Phys 2010;76:S94–100.
- Darby SC, Ewertz M, McGale P, Bennet AM, Blom- Goldman U, Brønnum D, et al. Risk of ischemic heart disease in women after radiotherapy for breast cancer. N Engl J Med 2013;368:987–98.
- Hall EJ. Intensity-modulated radiation therapy, protons, and the risk of second cancers. Int J Radiat Oncol Biol Phys 2006;65:1–7.
- Paganetti H, Bortfeld T, Delaney TF. Neutron dose in proton radiation therapy: In regard to Eric J. Hall (Int J Radiat Oncol Biol Phys 2006;65:1–7). Int J Radiat Oncol Biol Phys 2006;66:1594–5.
- Gottschalk B. Neutron dose in scattered and scanned proton beams: In regard to Eric J. Hall (Int J Radiat Oncol Biol Phys 2006;65:1–7). Int J Radiat Oncol Biol Phys 2006;66:1594.
- Chung CS, Yock TI, Nelson K, Xu Y, Keating NL, Tarbell NJ. Incidence of second malignancies among patients treated with proton versus photon radiation. Int J Radiat Oncol Biol Phys 2013;87:46–52.
- Engelsman M, Schwarz M, Dong L. Physics controversies in proton therapy. Semin Radiat Oncol 2013;23:88–96.
- Unkelbach J, Chan TCY, Bortfeld T. Accounting for range uncertainties in the optimization of intensity modulated proton therapy. Phys Med Biol 2007;52:2755–73.
- Knopf A-C, Hong TS, Lomax A. Scanned proton radiotherapy for mobile targets – the effectiveness of re-scanning in the context of different treatment planning approaches and for different motion characteristics. Phys Med Biol 2011;56:7257–71.
- Ajani JA, Mansfield PF, Janjan N, Morris J, Pisters PW, Lynch PM, et al. Multi-institutional trial of preoperative chemoradiotherapy in patients with potentially resectable gastric carcinoma. J Clin Oncol 2004;22:2774–80.
- Wang J, Wei C, Tucker SL, Myles B, Palmer M, Hofstetter WL, et al. Predictors of postoperative complications after trimodality therapy for esophageal cancer. Int J Radiat Oncol Biol Phys 2013;86:885–91.