To the Editor,
There are many indications that future radiotherapy treatment planning will be automated and protocol based [Citation1–3]. Today's clinics have multi-modality treatment equipment and are moving towards more individually optimised treatments. This coincides with a busy clinic's need to optimise its resources as they are often limited. Thus, it is important to identify patients in need of an advanced and resource demanding treatment modality, e.g. helical tomotherapy or intensity-modulated proton therapy (IMPT), as well as those patients that can receive an equivalent treatment with conventional and less resource demanding modalities, e.g. three-dimensional conformal radiation therapy (3DCRT) [Citation4]. Hence, to ensure that every patient receives the optimal radiotherapy treatment it is necessary to introduce multi-modality optimisation in the treatment planning process. Multi-modality optimisation can be achieved by creating an ideal (clinically optimal) treatment plan or a treatment plan made for the most advanced treatment modality available in the clinic. Then for every clinically optimal plan, plans for alternative treatment modalities are automatically created via ‘dose-mimicking’ and compared with the original plan with minimal extra effort required. Thus, for each patient, this procedure will make it possible to identify a clinically optimised (and potentially resource optimised) treatment plan.
In order to minimise the need for radiation oncologists (ROs) to spend a lot of their time examining and comparing a selection of treatment plans, there is a need for alternative ways of comparing the automatically produced plans. If a clinically ideal treatment plan is used as the goal for the ‘dose-mimicking’, our hypothesis is that ROs would grade the automatically produced plans in a similar way as if they were graded solely based on their composite objective values. The composite objective value (the value of the composite objective function) is a measure of how well the optimiser manages to fulfil predefined objectives and constraints in the optimisation process of an inversely planned radiotherapy treatment plan [Citation5,Citation6]. The value is lowered for each objective/constraint that the plan fulfils. It is a very attractive idea to use these values for plan quality comparisons. A purely objective single value is produced per plan as a direct result of the optimisation, which makes it fast and easy to use. However, the value is seldom relevant for plan comparison studies as the meaning of the value changes as soon as the objectives/ constraints or weight factors (included in the optimisation) are altered in any way. Additionally, the value has a different meaning in different treatment planning systems (TPS) (or optimisers). However, if optimisations are performed using the same objectives/constraints (and weighting factors) but for different treatment delivery techniques/modalities, the minimum objective values reached during the optimisations will be measures of the plan quality achievable for the different treatment delivery techniques/modalities [Citation3,Citation7,Citation8]. In this study, we investigate if the composite objective value could be useful for concluding the optimal treatment alternative in multi-modality radiotherapy treatment planning.
Material and methods
In order to test our hypothesis, we use the fallback planning module in RayStation® (RaySearch Laboratories AB, Stockholm, Sweden) which allows for automated creation and optimisation (via ‘dose-mimicking’) of coplanar/non-coplanar 3DCRT (with gantry, collimator, couch, and wedge angle optimisation as well as segment shape optimisation), step-and-shoot intensity-modulated radiation therapy (IMRT), and volumetric modulated arc therapy (VMAT) fallback plans from reference radiotherapy treatment plans of any modality [Citation6], e.g. TomoTherapy®, CyberKnife®, Vero®, electron, proton, or ion plans.
Details about the ‘dose-mimicking’ algorithm [Citation9]: The composite objective function during generation of a fallback plan is a weighted sum of reference dose-volume histogram (DVH) functions that impose a one-sided quadratic penalty on DVH curve error. Functions associated with organs at risk (OARs) are given unit weight while functions associated with targets are given a weight equal to a user-defined target priority. Reference DVH functions associated with OARs penalise overdosage with respect to the fallback DVH over the entire volume interval, whereas reference DVH functions associated with targets penalise overdosage for relative volumes in the interval [0, 0.5] and underdosage in the interval [0.5, 1.0]. All reference DVH functions are based on creating sets of DVH points for the reference dose and the present dose, dividing the volume interval into subintervals in which the dose levels of both curves are constant, e.g. for the subinterval [Vlow,Vhigh]with corresponding dose levels d and dref the contribution to the objective function value from the given subinterval will be:
where H is the Heaviside function.
Twelve patient cases with tumours in the head & neck (H 1-3), brain (B 1-3), abdominal (A 1-3) and pelvic regions (P 1-3) were used for the study. A ‘clinically ideal’ reference plan (a deliverable treatment plan of high quality, i.e. that fulfilled all ‘reasonable’ demands of our ROs for the specific case) was created in the TomoTherapy® TPS (Accuray® Incorporated, CA, USA) for each of the 12 cases. Plan parameters were chosen to achieve clinical treatment plans of the highest quality, i.e. a field width of 1.05 cm, a pitch of 0.172, and a modulation factor of 5. Such plans would not normally be used clinically (except perhaps for very small targets) since they would take too long to deliver. The tomotherapy plans were imported into RayStation® and fallback plans were produced. For each tomotherapy plan; one dual-arc VMAT, one seven-beam non-coplanar 3DCRT, and one seven-beam IMRT fallback plan were produced (a target weight priority of 100 was used for all of these plans). The composite objective value was registered and analysed for each plan. Wilcoxon signed-rank tests were applied on these values to determine if there was a significant difference between the treatment modalities.
A CGA method [Citation4] was used to further compare fallback plans generated for different treatment modalities. The method is inspired by visual grading analysis (VGA), a well-established method for determining image quality in radiology [Citation10]. We have previously shown that CGA is a useful method for comparing radiotherapy treatment plans [Citation4]. We used the same procedure [Citation4] in this study, i.e. the treatment plans were demonstrated for 10 experienced ROs individually. They were shown DVHs, region of interest (ROI) data, and dose distributions in every CT slice. To facilitate the comparison between different treatment modalities, the plans were shown side-by-side in the plan evaluation module. A grading scale was constructed and the ROs were asked to grade the IMRT/3DCRT plan on how it compared to the VMAT plan. Grade ‘A’ was given if the IMRT/3DCRT plan was judged as considerably better than the VMAT plan, ‘B’ as somewhat better, ‘C’ as equivalent, ‘D’ as somewhat worse, and ‘E’ as considerably worse. The ROs were also asked to motivate their judgment. None of the plans were compared to any of the reference tomotherapy plans. One-sided sign tests were performed in order to test the statistical significance of the clinical grading results from the plan comparisons, i.e. if there was a significant difference in how often D/E grades were given compared to A/B grades (is VMAT or IMRT/3DCRT better?) and also E grades compared to A/B/C grades (is VMAT considerably better?). The tests were performed on the results for all cases separately, for all ROs separately, as well as for all results combined. The significance level chosen was 5% (α = 0.05) for all statistical tests included in this study.
In order to further determine if the composite objective value is a useful parameter for determining the preferred (optimal) treatment modality/plan we evaluated the correlation between CGA results and the composite objective values using the Pearson's product-moment correlation method.
Results
The composite objective values for the VMAT plans were significantly lower than for the 3DCRT plans (p < 0.01), and IMRT plans (p = 0.01). There was no significant difference between the composite objective values for the 3DCRT and the IMRT plans. All 10 ROs agreed that overall, VMAT was the better fallback delivery technique for the 12 cases included in this study ( and Supplementary Table I, available online at: http://informahealthcare.com/doi/abs/10.3109/0284186X.2014.953255). For all cases combined the CGA gave a significant difference between the different delivery techniques in favour of VMAT, i.e. VMAT was graded as considerably better than 3DCRT and also better than IMRT (total p-values in and Supplementary Table I available online at: http://informahealthcare.com/doi/abs/10.3109/0284186X.2014.953255).
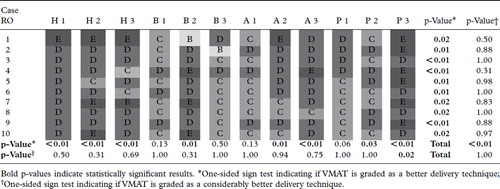
CGA results were in good agreement with the differences seen in composite objective values between the different treatment modalities, i.e. VMAT was graded as superior to IMRT for cases H 1-3, B 2, A 2-3, as well as P 2-3, i.e. cases with large differences in composite objective values between the treatment modalities (see and ). Furthermore, the cases for which 3DCRT was not graded as inferior to VMAT (B 1-3) also had the smallest differences in composite objective values between the treatment modalities and the cases for which 3DCRT was graded as considerably inferior to VMAT (H 1-3 and P 1-3) also had some of the largest differences in composite objective values (see Supplementary Table I available online at: http://informahealthcare.com/doi/abs/10.3109/0284186X.2014.953255 and ). There was a strong correlation (r = 0.76, p ≤ 0.01) between the difference in the logarithm of the composite objective values and the number of ROs that graded the VMAT plan as superior to the corresponding 3DCRT plan, for the different patient cases (Supplementary Figure 1 available online at: http://informahealthcare.com/doi/abs/10.3109/0284186X.2014.953255). A similar strong correlation (r = 0.74, p < 0.01) was found for the VMAT plans compared to the IMRT plans ().
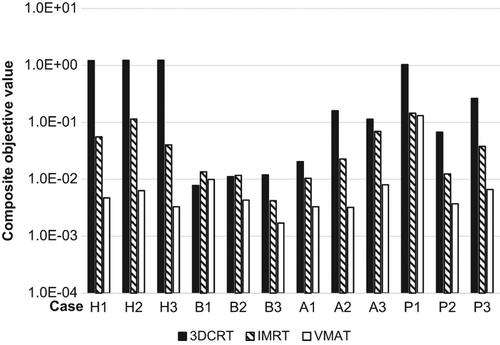
![Figure 2. The number of D and E grades for each case, from the clinical grading analysis (CGA) results and their correlation with the difference in the logarithm of the composite objective values [diff. log (OV)]; between volumetric modulated arc therapy (VMAT) and step-and-shoot intensity-modulated radiation therapy (IMRT).](/cms/asset/d9e82dce-670d-4469-bdae-161fa3b27505/ionc_a_953255_f0002_b.gif)
During the grading of the treatment plans all ROs mentioned that hot-spots of clinical significance occurred for the two most complex cases (P 1, and P 3), which rendered the treatment plans clinically unacceptable. This was most pronounced and occurred most frequently (for both P 1 and P 3) for IMRT but it also occurred for the other treatment modalities (only for case P 1). These hot-spots were the main reason why the CGA results did not always agree with the results from the objective value evaluation, as they were considered to be of clinical significance by the ROs but they barely influenced the composite objective values.
Discussion
Results from the CGA show that the composite objective value is useful as an evaluation parameter for choosing between fallback treatment modalities/plans, since cases with significant superiority for VMAT compared to 3DCRT and IMRT also have large differences in composite objective values. There was also a strong (and significant) correlation between the CGA results and results from the composite objective value evaluation, i.e. the number of ROs that preferred one treatment modality/plan and the difference in composite objective values. The study was performed with the fallback module in RayStation® but our results should be also be valid for other TPS, if the same predefined conditions exists, i.e. to produce treatment plans for different delivery techniques using the same objectives/constraints and weight factors.
The evaluation of composite objective values showed that a lower value was achievable with dual arc VMAT delivery technique compared to IMRT or 3DCRT. The ROs graded VMAT as a considerably superior treatment modality compared to 3DCRT, and also superior to IMRT for fallback planning. However, VMAT was only graded as considerably superior to IMRT for one (P 3) of the 12 patient cases. The CGA revealed that plans with hot-spots of clinical significance can be produced in the fallback planning of complex cases, especially for the IMRT delivery technique. They can occur since the ‘dose-mimicking’ optimisation is based solely on DVH data, which means that hot-spots barely influence the objective value. Furthermore, the CGA showed that the ROs were in fairly good agreement regarding which treatment modality they preferred. The few differences seen in the grading scores between the different ROs could be due to subjective preferences, differences in educational background, or due to the lack of specific treatment objectives in the clinic [Citation4,Citation11].
The reference plans used for the composite objective value and CGA evaluation were produced for a rotational delivery technique, i.e. helical tomotherapy. Hence, a rotational treatment modality might have an advantage in the evaluation. However, the reference plans were generated in a way that assures the highest plan quality achievable with photon delivery technique [Citation12,Citation13]. Consequently, the plan quality should depend on the similarity between the fallback plan and the reference plan. This is also supported by the results from the CGA. The composite objective value itself is of course also a measure of the quality of the fallback plan in comparison with the reference plan. We have not investigated in detail how automatic plan generation would work if an ‘idealised’ dose distribution was used as a reference. However, preliminary results indicate that when the quality of the reference plan is too far from what is achievable, then the optimiser prioritise differently than what a RO would when it tries to fulfil the objectives/constraints. Hence, the resulting treatment plan is perhaps theoretically (DVH-wise) of high quality but clinically of low quality, i.e. far from what a RO would consider as clinically acceptable. Similarly to results seen in this study for the complex case (P1), for which the optimiser struggled to fulfil the objectives and as a result hot-spots of clinical significance occurred.
In our study, VMAT was the technique generally preferred by the ROs. However, for our brain tumour cases the less resource demanding 3DCRT technique could produce treatment plans which were judged as equivalent to the VMAT plans. It is important to be able to identify such cases in order to optimise the use of a clinic's advanced radiotherapy treatment resources [Citation4]. This identification can be performed effortless with automatic planning and plan evaluation of the generated plans using their composite objective values. This method should also be useful for comparing other delivery techniques, e.g. for comparing proton/ion treatment plans to photon-based plans (VMAT), which are deliverable at your ‘home’ clinic. This could be used to optimise your treatment resources or to investigate different fallback alternatives in case of downtime at the proton/ion treatment facility.
This study presents the composite objective value as a useful parameter for choosing the optimal fallback treatment modality/plan. Another useful method for concluding the optimal treatment modality is the Pareto front evaluation method which has some advantages compared to conventional methods based on DVHs [Citation4,Citation11,Citation14,Citation15]. However, unlike our standard use of the Pareto front evaluation, all dimensions of the optimisation are taken into account in the objective value evaluation, i.e. the evaluation is not limited to two quantitative parameters or objective functions (target coverage and sparing of an OAR). Instead, all objectives and constraints included in the optimisation are also part of the evaluation. So, where Pareto front evaluation describes the sharpness of a dose gradient in the overlapping region of the target and an OAR, the composite objective value evaluation can account for the entire dose distribution.
The results from the CGA confirmed that the composite objective value was useful for concluding the optimal treatment alternative for a variety of tumour sites and patient cases, in multi-modality optimisation. In case automated multi-modality optimisation is the future for radiotherapy treatment planning, the method presented in this study can facilitate the production of clinically as well as resource optimised treatment plans.
Supplementary material available online
Supplementary Figure 1 and Table I available online at: http://informahealthcare.com/doi/abs/10.3109/0284186X.2014.953255.
ionc_a_953255_sm2216.pdf
Download PDF (127.7 KB)Acknowledgements
The authors would like to acknowledge the following ROs; Jacob Engellau, Elisabeth Kjellén, Anders Ask, Adalsteinn Gunnlaugsson, Michael Garkavij, Karin Belfrage, Sven-Börje Ewers, Alexander Verbitski, Maria Gebre-Medhin, and Silke Engelholm who with their time spent grading plans helped to accomplish this project.
Declaration of interest: The authors report no conflicts of interest. The authors alone are responsible for the content and writing of the paper.
References
- Breedveld S, Storchi PR, Voet PW, Heijmen BJ. iCycle: Integrated, multicriterial beam angle, and profile optimization for generation of coplanar and noncoplanar IMRT plans. Med Phys 2012;39:951–63.
- Craft DL, Hong TS, Shih HA, Bortfeld TR. Improved planning time and plan quality through multicriteria optimization for intensity-modulated radiotherapy. Int J Radiat Oncol Biol Phys 2012;82:e83–90.
- Petersson K, Ceberg C, Engström P, Benedek H, Nilsson P, Knöös T. Conversion of helical tomotherapy plans to step-and-shoot IMRT plans – Pareto front evaluation of plans from a new treatment planning system. Med Phys 2011;38:3130–8.
- Petersson K, Engellau J, Nilsson P, Engstrom P, Knoos T, Ceberg C. Treatment plan comparison using grading analysis based on clinical judgment. Acta Oncol 2013;52:645–51.
- Haas O. Radiotherapy treatment planning: New system approaches. (Advances in industrial control). Grimble MJ, Johnson MA, editors. London: Springer-Verlag London Ltd.; 1999.
- RayStation 3.5 User Manual. RaySearch Laboratories AB, 2013.
- Weiss E, Siebers JV, Keall PJ. An analysis of 6-MV versus 18-MV photon energy plans for intensity-modulated radiation therapy (IMRT) of lung cancer. Radiother Oncol 2007;82:55–62.
- Suh Y, Weiss E, Zhong H, Fatyga M, Siebers JV, Keall PJ. A deliverable four-dimensional intensity-modulated radiation therapy-planning method for dynamic multileaf collimator tumor tracking delivery. Int J Radiat Oncol Biol Phys 2008;71:1526–36.
- Multi criteria optimization in RayStation. Stockholm: RaySearch Laboratories AB; 2012.
- Båth M, Månsson LG. Visual grading characteristics (VGC) analysis: A non-parametric rank-invariant statistical method for image quality evaluation. Br J Radiol 2007;80:169–76.
- Knöös T, Benedek H, Ceberg C, Nilsson P, Petersson K. Uncertainties in the evaluation of treatment plans. In: Palta JR, Mackie TR, editors. Uncertainties in external beam radiation therapy: AAPM Medical Physics Monograph No 35 Madison, WI: Medical Physics Publishing; 2011. p. 117–27.
- Oliver M, Ansbacher W, Beckham WA. Comparing planning time, delivery time and plan quality for IMRT, RapidArc and Tomotherapy. J Appl Clin Med Phys 2009;10:3068.
- Pasquier D, Cavillon F, Lacornerie T, Touzeau C, Tresch E, Lartigau E. A dosimetric comparison of tomotherapy and volumetric modulated arc therapy in the treatment of high-risk prostate cancer with pelvic nodal radiation therapy. Int J Radiat Oncol Biol Phys 2013;85: 549–54.
- Ottosson RO, Engstrom PE, Sjostrom D, Behrens CF, Karlsson A, Knoos T, et al. The feasibility of using Pareto fronts for comparison of treatment planning systems and delivery techniques. Acta Oncol 2009;48:233–7.
- Thor M, Benedek H, Knoos T, Engstrom P, Behrens CF, Hauer AK, et al. Introducing multiple treatment plan-based comparison to investigate the performance of gantry angle optimisation (GAO) in IMRT for head and neck cancer. Acta Oncol 2012;51:743–51.