Abstract
Background. Proton therapy offers superior low and intermediate radiation dose distribution compared with photon-based radiation for brain and skull base tumors; yet tissue within and adjacent to the target volume may receive a comparable radiation dose. We investigated the tolerance of the pediatric brainstem to proton therapy and identified prognostic variables.
Material and methods. All patients < 18 years old with tumors of the brain or skull base treated from 2007 to 2013 were reviewed; 313 who received > 50.4 CGE to the brainstem were included in this study. Brainstem toxicity was graded according to the NCI Common Terminology Criteria for Adverse Events v4.0.
Results. The three most common histologies were ependymoma, craniopharyngioma, and low-grade glioma. Median patient age was 5.9 years (range 0.5–17.9 years) and median prescribed dose was 54 CGE (range 48.6–75.6 CGE). The two-year cumulative incidence of toxicity was 3.8% ± 1.1%. The two-year cumulative incidence of grade 3 + toxicity was 2.1% ± 0.9%. Univariate analysis identified age < 5 years, posterior fossa tumor location and specific dosimetric parameters as factors associated with an increased risk of toxicity.
Conclusion. Utilization of current national brainstem dose guidelines is associated with a low risk of brainstem toxicity in pediatric patients. For young patients with posterior fossa tumors, particularly those who undergo aggressive surgery, our data suggest more conservative dosimetric guidelines should be considered.
Although first described in 1930 [Citation1], radiation- related central nervous system (CNS) toxicity is still poorly understood. It is largely classified by its temporal relationship to radiation [Citation2] and thought to originate following microvascular damage [Citation3]. The incidence of radiation injury to the pediatric brainstem and its associated risk factors are particularly ill-described, and unique disease processes and host factors can confound attempts to extrapolate pediatric guidelines from adult data on brainstem radiation tolerance. Given the high relative proportion of pediatric tumors requiring radiation to the posterior fossa and skull base, more accurate data on brainstem toxicity is needed for designing safe radiation plans, comparing the risks of alternate therapeutic modalities (such as chemotherapy and surgery), and estimating side effects so that caregivers can be informed of treatment risks.
In treating pediatric tumors of the brain and skull base, proton therapy results in superior low and intermediate radiation dose distribution compared to photon-based radiation, a property which can be especially advantageous around the brainstem. This translates into a reduction in acute [Citation4–6] and late side effects [Citation7–10] across a range of organ systems. Nevertheless, tissue within and immediately adjacent to the target volume receives a comparable high dose of radiation. Potential differences in the radiobiologic effect between photon and proton therapy on white matter or the vascular endothelium may make the existing brainstem dose-effect models, which are predominantly based on photon therapy in adults [Citation11], even less reliable. Thus the purpose of this study was to investigate the tolerance of the pediatric brainstem to proton therapy based on clinical data.
Material and methods
Under an institutional review board-approved study, the medical records of all patients ≤ 18 years old with tumors of the brain or skull base treated at the University of Florida Proton Therapy Institute (UFPTI) were reviewed. Patients with primary brainstem gliomas were excluded because in these patients the brainstem integrity is compromised prior to radiotherapy by the tumor itself. Patients who received < 50.4 Cobalt Gray Equivalent (CGE) to the brainstem were excluded. Patients who received prior radiation to any area were also excluded.
From August 2006 to August 2013, 563 patients ≤ 18 years old were treated with proton therapy at UFPTI. Of these, 313 patients met the inclusion criteria. and Supplementary Table I (to be found online at http://informahealthcare.com/doi/abs/10.3109/0284186X.2014.957414) summarize patient, tumor, and treatment characteristics.
Table I. Patient, tumor, and preradiotherapy characteristics (N = 313).
Patient follow-up testing was standard and consistent with Children's Oncology Group (COG) disease protocols and late effect guidelines where applicable. None of the 313 patients were lost to follow-up. Imaging evaluations were primarily magnetic resonance imaging (MRI)-based and focused on T1, T2, and fluid attenuated inversion recovery (FLAIR) sequences with and without contrast. All patients had a gross tumor volume (GTV) or tumor bed, clinical target volume (CTV), and planning target volume (PTV) defined. Daily image guidance was used in all cases to achieve a PTV margin of 3 mm. Standard institutional planning goals dictate that the PTV is encompassed by the 95% isodose line and the CTV is encompassed by the 99% isodose line. The brainstem was defined to encompass the midbrain, pons, and medulla. The cranial border was defined at the inferior edge of the third ventricle and optic tracts. The caudal extent was defined at the foramen magnum. For analysis, the brainstem surface was also defined as the most peripheral 3-mm edge and the brainstem core was defined as the brainstem minus the brainstem surface. Consistent with the largest previous publication [Citation12], brainstem toxicity following radiation was defined by new or progressive symptoms involving cranial nerves V-VII or IX-XII, motor weakness, or dysmetria with a corresponding radiographic abnormality within the brainstem in the absence of disease progression. Magnetic resonance (MR) spectrography was not routinely performed. Per the recommendations set forth in the Quantitative Analyses of Normal Tissue Effects in the Clinic (QUANTEC) report [Citation13], brainstem toxicity was graded using the National Cancer Institute (NCI) Common Terminology Criteria for Adverse Events (CTCAE v4.0) for ‘central nervous system necrosis’ to harmonize the findings with National Cancer Institute (NCI) Cancer Therapy Evaluation Program (CTEP) protocols. Brainstem toxicity of grades 2–5 (i.e. symptomatic toxicity) was recorded. In accordance with NCI sponsored guidelines for the use of protons, proton therapy dose is reported in CGE (1 CGE = 1 proton Gy × 1.1). The passive scattered beam delivery at UFPTI has been credentialed by the United States Radiologic Physics Center and treatment was delivered according to the Advanced Technology Consortium proton therapy guidelines (available through www.qarc.org). Our standard proton treatment technique included a number of precautions to minimize the risk of theoretical end of beam relative biological effectiveness (RBE) uncertainty. This includes multi-field plans and permitting no more than 1/3 of the beams to end in brainstem tissue outside the PTV. All 313 patients in this study were treated with multiple fields daily and > 99% of treatments were delivered with ≥ 3 beams (range 2–6).
To achieve normal-tissue tolerance goals, 129 patients had a planned field reduction/boost as part of their treatment plan. Thirty-nine patients (12.4%) were treated with CSI prior to a tumor bed boost. Thirty-one (9.9%) patients were treated with a component of photon therapy, either planned (n = 4; median, 25 days; range 25–28 days) due to field size limitations or skin dose reduction or unplanned (n = 27; median, 2 days; range 1–4 days) due to cyclotron downtime.
SAS and JMP software was used for all statistical analyses (SAS Institute, Cary, NC, USA). The Kaplan-Meier product limit method was used to estimate time to brainstem toxicity; the log-rank test statistic was used to detect statistically significant differences between strata of selected prognostic factors predictive of brainstem toxicity (). These factors included sex, race, age, tumor location, presence of hydrocephalus, need for a cerebrospinal fluid shunt, number of operations prior to RT, extent of resection, use of any chemotherapy, specific use of intrathecal or high-dose intravenous methotrexate, use of a craniospinal radiotherapy component, and use of a mixed modality (proton+ photon) treatment plan.
Table II. Clinical and dosimetric variables potentially associated with brainstem toxicity.
Results
In all 313 patients, the brainstem dose was within QUANTEC constraints and, when applicable, COG disease protocol guidelines, which predict a < 5% rate of brainstem necrosis. The mean brainstem V54 CGE was 26% (range 0–100%). The mean (and range) brainstem D10%, D50%, and D90% were 51.3 CGE (5.0–61.6 CGE), 37.4 CGE (0.6–59.2 CGE), and 18.2 CGE (0.1–58.1 CGE), respectively.
With a median follow-up of two years, the two-year overall survival rate for the 313 patients was 90.5%. Overall, 11 of 313 patients have experienced brainstem toxicity attributable at least in part to their radiation treatment: seven (2.2%) with grade 2, one (0.3%) with grade 3, two with grade 4 (0.6%), and one (0.3%) with grade 5. The median time to symptom onset was three months (range 2–12 months). Overall, the two-year cumulative incidence of any brainstem toxicity was 3.8% ± 1.1%. The two-year cumulative incidence of serious (grade 3+) brainstem toxicity was 2.1% ± 0.9%. The clinical manifestation of brainstem injury included primarily ataxia and lower cranial nerve signs. For the affected patients, clinical data are summarized in . At the time of analysis, symptoms have stabilized or resolved in 9 of 10 living patients.
is a comprehensive dose-volume histogram (DVH) including each patient's DVH curve with the colored curves indicating the 11 patients who experienced toxicities as shown in . Consistent with the overall series, all patients with brainstem toxicity had 99% of their daily treatment delivered through three or more fields. In each case with toxicity, the PTV overlapped with part of the brainstem, which was taken into consideration in field design and orientation. illustrates the clinical variables statistically associated with brainstem toxicity. Of note, patients < 5 years old had a high rate of brainstem toxicity (6.9% vs. 1.1%, p < 0.01) and all of the patients with brainstem toxicity had primary tumors located in the posterior fossa. Restricting the exploratory analysis to just patients with posterior fossa tumors, the two-year cumulative rate in children < 5 years old was slightly higher (12.5%) than the rate in children 5 years and older (7.2%). Although age may be an independent predictor of toxicity when controlling for tumor location, the exact interplay between these factors is uncertain as a more comprehensive multivariate Cox regression analysis is not feasible given the small number of events.
Figure 1. A comprehensive dose-volume histogram (DVH) including each patient's DVH curve. The colored curves correspond with the 11 patients who experienced toxicities outlined in as follows:










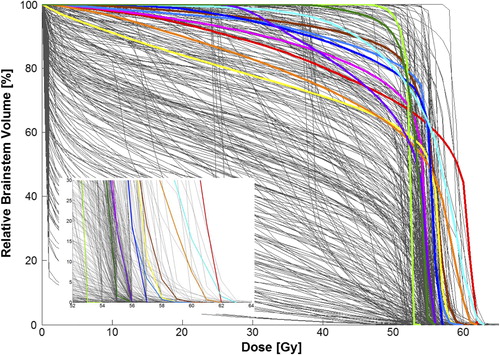
Table III. Patient, tumor, and treatment characteristics for patients who experienced brainstem toxicity.
Discussion
To our knowledge, this study presents the largest and most comprehensive analysis of pediatric brainstem radiation tolerance to date, independent of radiation modality. It also provides a unique perspective on the use of proton radiation for pediatric patients with CNS and skull base tumors. We found that when competing risks are considered, the cumulative incidence of serious brainstem toxicity is 2.1% but may be higher for certain patient groups, such as young patients with posterior fossa tumors. In these patients, particularly those who undergone aggressive surgery, more conservative brainstem dose guidelines should be considered. Our data suggests that the parameters of V55 Gy and maximum brainstem dose may be the most useful modifiable factors to consider in treatment planning.
Assessing rates of radiation-related brainstem toxicity across the academic literature can be challenging. Imaging techniques are largely non-specific and few studies involve histopathologic analysis; therefore, differences in clinical terminology lead to varying estimates. For example, some authors have labeled ‘radiation necrosis’ as any changes noted on imaging that are thought to represent a treatment effect rather than a recurrent tumor [Citation14]. Others reserve the term ‘necrosis’ for patients with symptomatic and irreversible radiation damage, intending to distinguish it from less severe and reversible ‘radiation injury’ [Citation15]. Certain authors introduce temporal criteria, where necrosis specifically connotes ‘late injury’ [Citation14]. Further complicating these estimates is the addition of chemotherapy to radiation for treatment of tumors in the brain or skull base. Although many chemotherapy agents sensitize tumor cells to radiation, the contribution of chemotherapy to radiation brainstem toxicity is poorly understood. Some authors have described changes similar to radiation necrosis in patients who received chemotherapy alone for extracranial malignancies [Citation16]. A third explanation for the broad differences in the incidence of radiation necrosis may be related to the evolution of diagnostic imaging. The application of MRI and functional imaging in defining radiation brainstem toxicity widely varies across series and eras, with most later series reporting ‘higher’ incidences [Citation14]. In the current series, the radiographic findings in patients with toxicity usually included both focal T2 prolongation and contrast enhancement within the brainstem. Some patients demonstrated additional focal radiographic findings in the cerebellar parenchyma, similar but separate from the brainstem abnormalities. The imaging changes within the brainstem were often multifocal and occurred within various isodose lines ranging from 50 CGE to > 60 CGE. Across all patients, there was no clear spatial dose-effect relationship observed on the imaging studies beyond 50 CGE nor were the imaging findings consistent with a clear vascular distribution. In no cases were the radiographic findings exclusively limited to the end range of the proton beams. Finally, due to the overall low incidence of pediatric brain and skull base tumors, many estimates of pediatric brainstem toxicity encompass non-brainstem CNS tissue and extrapolate data from patients of all ages [Citation11,Citation13]. This may be a mischaracterization if the pediatric brainstem is in fact more radiosensitive. Acknowledging these limitations, the rate of reported symptomatic brainstem toxicity in pediatric patients who receive photon-based radiotherapy at standard dose and fractionation is somewhere between 2% and 18%, and the risk of fatal (grade 5) necrosis ranges from 0.4% to 2% (Supplementary Table I to be found online at http://informahealthcare.com/doi/abs/10.3109/0284186X.2014.957414).
Obtaining an accurate dose-effect estimate for brainstem tolerance is even more challenging because the actual brainstem dose is rarely reported or analyzed [Citation17–23]. Instead, the nominal prescription dose is reported, which may bear little relation to the actual brainstem dose accounting for the injury and often does not include important information on the tumor location and treatment technique. As a result, many of the patients included in the studies listed in Supplementary Table II (to be found online at http://informahealthcare.com/doi/abs/10.3109/0284186X.2014.957414) actually could have received < 50 Gy to the brainstem. As the risk of brainstem injury approximates 0% at doses of < 50 Gy [Citation13,Citation18], including these patients in such analyses might exert a downward bias on the risk estimate of brainstem toxicity. Therefore, the current study is valuable and unique as it is the only study that: 1) specifically reports brainstem toxicity by brainstem dose across a variety of tumors; 2) correlates radiographic and clinical findings; and 3) excludes patients who received < 50.4 Gy to the brainstem and thus are not at measurable risk. Together, these factors allow for an accurate and generalizable dose-effect analysis.
The data provided herein may help guide treatment and radiation plan design. outlines various dosimetric parameters that can be used to shape the volume of brainstem receiving high doses in young patients. It is important to also consider, however, the clinical factors that might identify patients at higher risk. It was not surprising that younger patients were more susceptible to radiation damage and that the average latency time observed in our series was only three months. These findings are consistent with long-standing radiobiologic principles and animal studies [Citation24,Citation25]. We observed that patients who have undergone a gross total resection might be at higher risk. Other authors have suggested that morbidity associated with aggressive surgery may play a role in increased brainstem sensitivity to radiation [Citation12,Citation26]. These operations might involve subclinical devascularization or neurological injury, making the brainstem less resilient to higher radiation doses. Adjuvant systemic therapy, particularly involving agents with known neurotoxic or vascular effects, may play a role [Citation18]. The patient who experienced grade 5 toxicity was a 7-year-old girl with a posterior fossa ependymoma enrolled on COG's study ACNS0831 who received a prescription dose of 59.4 CGE in accordance with protocol guidelines. Approximately four months after completing treatment, she developed ataxia and lower cranial nerve palsy, which stabilized on steroids and hyperbaric oxygen therapy. She was transitioned to bevacizumab and shortly thereafter experienced an acute brainstem stroke causing respiratory failure. It is not clear what role the VEGF inhibitor played in her cerebrovascular event. Although promising in the treatment of radiation toxicity, bevacizumab has also been associated with thrombotic microangiopathy [Citation27,Citation28].
This study is also unique in that it reports the outcomes of patients treated with proton therapy. The most widely accepted current hypothesis is that CNS radiation necrosis is caused by vascular endothelial damage [Citation3] leading to oligodendrocyte damage and demyelination. The pons is particularly susceptible to demyelination [Citation29]. In patients treated with therapeutic radiation, ionization density within sensitive cellular structures (e.g. DNA) increases with linear energy transfer (LET) and apoptosis increases with ionization density. In the common clinical setting, double-scattered proton therapy is regarded to have approximately the same LET as photon radiation when utilizing a spread-out Bragg peak (SOBP) to cover the tumor volume. However, when examining differences in the LET along the SOBP, the LET increases slightly throughout the SOBP and then significantly at the terminal end of the SOBP [Citation30]. In theory, endothelial cells at the edge of the SOBP could experience an enhanced rate of apoptosis causing a cascade of events leading to demyelination and brainstem injury. For this reason, we continue to recommend that no more than one third of proton beams end in brainstem tissue outside the PTV. When this approach is employed, our data does not suggest an increased rate of brainstem toxicity with proton therapy compared to modern series involving adult and children treated with photon-based radiation (Supplementary Table II to be found online at http://informahealthcare.com/doi/abs/10.3109/0284186X.2014.957414). However, further follow-up of this cohort will be necessary to determine long-term effects.
In conclusion, brainstem radiation injury is a tragic yet rarely fatal toxicity encountered when delivering curative radiotherapy to children with CNS or skull base tumors. Across all radiotherapy modalities, we strive for better predictive models to further reduce the rate of brainstem damage. Our data provide a necessary foundation for brainstem radiation dose toxicity estimates and contribute additional information to the body of literature on risk factors. Our findings suggest that current QUANTEC and COG guidelines should generally be associated with a low risk of brainstem toxicity in pediatric patients and are equally applicable to proton- and photon-based therapy when appropriate precautions are utilized regarding proton beam orientation. For young patients with posterior fossa tumors, particularly those who undergo aggressive surgery, we recommend more conservative guidelines outside the clinical trial setting. As a precautionary measure based on the theoretical concerns of enhanced LET, pediatric radiation oncologists utilizing proton therapy should continue to closely monitor and actively report toxicity outcomes.
http://informahealthcare.com/doi/abs/10.3109/0284186X.2014.957414
Download PDF (26.9 KB)Acknowledgments
We would like to thank Jessica Kirwan and the editorial staff of the University of Florida, Department of Radiation Oncology for editing and preparing this manuscript for publication.
Declaration of interest: The authors report no conflicts of interest. The authors alone are responsible for the content and writing of the paper.
References
- Fischer AW, Holfelder. Lokales Amyloid im Gehirn. Deut Z Chir 1930;227:475–83.
- Sheline GE, Wara WM, Smith V. Therapeutic irradiation and brain injury. Int J Radiat Oncol Biol Phys 1980;6:215–28.
- Coderre JA, Morris GM, Micca PL, Hopewell JW, Verhagen I, Kleiboer BJ, et al. Late effects of radiation on the central nervous system: Role of vascular endothelial damage and glial stem cell survival. Radiat Res 2006;166:495–503.
- Suneja G, Poorvu PD, Hill-Kayser C, Lustig RA. Acute toxicity of proton beam radiation for pediatric central nervous system malignancies. Pediatr Blood Cancer 2013;60:1431–6.
- Huh WW, Fitzgerald N, Mahajan A, Sturgis EM, Beverly Raney R, Anderson PM. Pediatric sarcomas and related tumors of the head and neck. Cancer Treat Rev 2011;37:431–9.
- Habrand JL, Schneider R, Alapetite C, Feuvret L, Petras S, Datchary J, et al. Proton therapy in pediatric skull base and cervical canal low-grade bone malignancies. Int J Radiat Oncol Biol Phys 2008;71:672–5.
- Beltran C, Roca M, Merchant TE. On the benefits and risks of proton therapy in pediatric craniopharyngioma. Int J Radiat Oncol Biol Phys 2012;82:e281–7.
- Perez-Andujar A, Newhauser WD, Taddei PJ, Mahajan A, Howell RM. The predicted relative risk of premature ovarian failure for three radiotherapy modalities in a girl receiving craniospinal irradiation. Phys Med Biol 2013;58:3107–23.
- Zhang R, Howell RM, Homann K, Giebeler A, Taddei PJ, Mahajan A, et al. Predicted risks of radiogenic cardiac toxicity in two pediatric patients undergoing photon or proton radiotherapy. Radiat Oncol 2013;8:184.
- Zhang R, Howell RM, Giebeler A, Taddei PJ, Mahajan A, Newhauser WD. Comparison of risk of radiogenic second cancer following photon and proton craniospinal irradiation for a pediatric medulloblastoma patient. Phys Med Biol 2013; 58:807–23.
- Marks LB, Yorke ED, Jackson A, Ten Haken RK, Constine LS, Eisbruch A, et al. Use of normal tissue complication probability models in the clinic. Int J Radiat Oncol Biol Phys 2010;76:S10–9.
- Merchant TE, Chitti RM, Li C, Xiong X, Sanford RA, Khan RB. Factors associated with neurological recovery of brainstem function following postoperative conformal radiation therapy for infratentorial ependymoma. Int J Radiat Oncol Biol Phys 2010;76:496–503.
- Mayo C, Yorke E, Merchant TE. Radiation associated brainstem injury. Int J Radiat Oncol Biol Phys 2010;76:S36–41.
- Giglio P, Gilbert MR. Cerebral radiation necrosis. Neurologist 2003;9:180–8.
- Chao ST, Ahluwalia MS, Barnett GH, Stevens GH, Murphy ES, Stockham AL, et al. Challenges with the diagnosis and treatment of cerebral radiation necrosis. Int J Radiat Oncol Biol Phys 2013;87:449–57.
- Burger PC, Kamenar E, Schold SC, Fay JW, Phillips GL, Herzig GP. Encephalomyelopathy following high-dose BCNU therapy. Cancer 2006;48:1318–27.
- Shaw E, Arusell R, Scheithauer B, O’Fallon J, O’Neill B, Dinapoli R, et al. Prospective randomized trial of low- versus high-dose radiation therapy in adults with supratentorial low-grade glioma: Initial report of a North Central Cancer Treatment Group/Radiation Therapy Oncology Group/Eastern Cooperative Oncology Group study. J Clin Oncol 2002; 20:2267–76.
- Ruben JD, Dally M, Bailey M, Smith R, McLean CA, Fedele P. Cerebral radiation necrosis: Incidence, outcomes, and risk factors with emphasis on radiation parameters and chemotherapy. Int J Radiat Oncol Biol Phys 2006;65:499–508.
- Fouladi M, Chintagumpala M, Laningham FH, Ashley D, Kellie SJ, Langston JW, et al. White matter lesions detected by magnetic resonance imaging after radiotherapy and high-dose chemotherapy in children with medulloblastoma or primitive neuroectodermal tumor. J Clin Oncol 2004;22:4551–60.
- Merchant TE, Li C, Xiong X, Kun LE, Boop FA, Sanford RA. Conformal radiotherapy after surgery for paediatric ependymoma: A prospective study. Lancet Oncol 2009;10:258–66.
- Spreafico F, Gandola L, Marchiano A, Simonetti F, Poggi G, Adduci A, et al. Brain magnetic resonance imaging after high-dose chemotherapy and radiotherapy for childhood brain tumors. Int J Radiat Oncol Biol Phys 2008;70:1011–9.
- Bishop MW, Hummel TR, Leach J. Radiation injury in pediatric patients with cns tumors treated with proton beam radiation therapy: A case series. Neuro Oncol 2012;14:148–56.
- Murphy ES, Merchant TE, Wu S, Xiong X, Lukose R, Wright KD, et al. Necrosis after craniospinal irradiation: Results from a prospective series of children with central nervous system embryonal tumors. Int J Radiat Oncol Biol Phys 2012;83:e655–60.
- Friedman DL, Constine LS. Late effects of cancer treatment. In: Halperin EC, Constine LS, Tarbell NJ, Kun LE, editors. Pediatric radiation oncology. Philadelphia: Lippincott, Williams & Wilkins; 2005.
- Ruifrok AC, Kleiboer BJ, van der Kogel AJ. Reirradiation tolerance of the immature rat spinal cord. Radiother Oncol 1992;23:249–56.
- Debus J, Hug EB, Liebsch NJ, O’Farrel D, Finkelstein D, Efird J, et al. Brainstem tolerance to conformal radiotherapy of skull base tumors. Int J Radiat Oncol Biol Phys 1997;39:967–75.
- Eremina V, Jefferson JA, Kowalewska J, Hochster H, Haas M, Weisstuch J, et al. VEGF inhibition and renal thrombotic microangiopathy. N Engl J Med 2008;358:1129–36.
- Schraermeyer U, Julien S. Formation of immune complexes and thrombotic microangiopathy after intravitreal injection of bevacizumab in the primate eye. Graefes Arch Clin Exp Ophthalmol 2012;250:1303–13.
- Riggs JE, Schochet SS, Jr. Osmotic stress, osmotic myelinolysis, and oligodendrocyte topography. Arch Pathol Lab Med 1989;113:1386–8.
- Paganetti H, Niemierko A, Ancukiewicz M, Gerweck LE, Goitein M, Loeffler JS, et al. Relative biological effectiveness (RBE) values for proton beam therapy. Int J Radiat Oncol Biol Phys 2002;53:407–21.