Abstract
Background. Cardiac morbidity is an important late effect in long-term childhood cancer survivors (CCS) treated with cardiotoxic agents or radiotherapy (RT) on the chest. However, there is limited data on the long-term cardiac sequelae in CCS who only received cranial RT. We hypothesized that cranial RT might negatively influence cardiac structure and function.
Methods and results. We studied 13 CCS [mean age 30.8 (18.1–39.3) years, 7 males] who received RT only on the head for a cranial tumor and 36 age- and sex-matched healthy sibling controls. Echocardiographic follow-up was performed at median 21.7 (12.6–30.8) years after diagnosis. CCS had lower indexed diastolic LV volumes [56.0 (31.4–68.3) vs. 60.5 (41.9–94.3) mL/m2, p = 0.024]. CCS also had reduced LV systolic and diastolic function, reflected by lower systolic LV myocardial velocities (5.3 ± 0.9 vs. 7.1 ± 1.7 cm/s, p = 0.001) and longitudinal deformation (− 17.3 ± 3.1 vs. − 20.7 ± 2.0%, p < 0.001), as well as lower diastolic LV myocardial velocities (− 10.7 ± 1.7 vs. − 12.2 ± 1.5 cm/s, p = 0.006) and deformation speed (1.1 ± 0.3 vs. 1.5 ± 0.2 1/s, p = 0.005). Additionally, in CCS insulin-like growth factor levels [15.4 (9.2–34.6) vs. 24.4 (14.8–55.5) nmol/L, p = 0.007] were lower.
Conclusion. Cranial RT in CCS is associated with smaller cardiac volumes and reduced systolic and diastolic LV function. This off target effect of RT might be related to lower insulin-like growth factor levels.
Cardiac damage is an important late effect in childhood cancer survivors (CCS) [Citation1]. It can be caused by several cytotoxic drugs, but also by radiotherapy (RT) [Citation2]. Dependent on the target region, RT increases the risk of cardiac morbidity. Several studies have shown an association between mediastinal/thoracic RT and subsequent coronary artery disease, pericardial disease and myocardial fibrosis [Citation3–5]. In addition, myocardial perfusion defects are described in patients who are treated with RT for breast cancer [Citation6,Citation7] which can result in cardiac wall motion abnormalities. However, cardiac effects of RT outside the thoracic region have not been fully investigated. In this study we evaluated whether RT on the head, as a single treatment without chemotherapy, has long-term effects on cardiac function. Vascular status was assessed since recent observations in CCS suggest an off-target effect of RT on the vascular system [Citation8], which might in turn impact cardiac structure and function [Citation9–12]. In addition, we evaluated the growth hormone axis because hypopituitarism is a common complication after cranial RT and growth hormone deficiency frequently is the first manifestation of hypopituitarism [Citation13]. Growth hormone plays an important role in body composition and muscle development, including the myocardium, and growth hormone deficiency has a negative effect on the cardiac function [Citation14]. We hypothesized that cranial RT might negatively influence cardiac structure and function, and that these effects (if demonstrable) might be related to growth hormone deficiency and/or deranged vascular function.
Material and methods
This study used data obtained in a recent study on late cardiovascular sequelae in a large group of CCS [Citation1]. For the present analysis we selected the subgroup of CCS who were diagnosed with a cranial tumor or a tumor in the high neck region who received local (non-thoracic) RT as single therapy without any additional systemic treatment with anthracyclines or other cytostatics. Age-matched healthy subjects (2 controls per CCS), drawn from the control group of the main study, served as controls. All subjects gave written informed consent and the study was approved by the local medical ethics committee.
Echocardiography was performed by one single operator (Y.M.H.), who was blinded for the status of the subjects. Echocardiographic examinations were performed on a VIVID-7 (General Electric, Horten, Norway) utilizing a 2.5–3.5 MhZ probe, and the measurements were performed in accordance with ASE and EAE recommendations [Citation15]. Left ventricular (LV) dimensions were acquired on two-dimensional (2D) images. LV and left atrial volumes were measurements and indexed for body surface area where appropriate. Evaluation of LV diastolic function consisted of transmitral inflow measurements (early mitral valve inflow, late mitral valve inflow, deceleration time and isovolumetric relaxation time) and early diastolic tissue velocities (e’) and early diastolic strain rate. Evaluation of LV systolic function consisted of LV ejection fraction (LVEF), stroke volume, systolic tissue velocity (s’) and longitudinal strain (Ɛ). All tissue velocities (e’ and s’) were measured at the septal and lateral side of the mitral annulus, and averaged. LV systolic and diastolic longitudinal deformation measurements were performed using 2D speckle tracking technique on offline images using an ECHOPAC system (General Electric, Horten, Norway). LV longitudinal deformation, i.e. longitudinal strain (Ɛ), represents a change in the dimension of an object normalized to its original dimension and is expressed in a percentage, a negative value representing tissue shortening/contraction in the longitudinal direction of the LV. Early diastolic deformation speed (1/s) represents the velocity of 1 unit of deformation in the early phase of the diastole, and is considered a measure of diastolic tissue function (relaxation). Finally, we evaluated post-systolic tissue shortening, which is considered present in case tissue shortening occurs after aortic valve closure [Citation16]. For this purpose, we evaluated 12 LV segments (septal, lateral, anterior and inferior; at the basal, mid and apical level). In case > 2 segments were not analyzable by the 2D speckle tracking software the analysis was discarded. Vascular structure and function were evaluated through measurement of carotid intima media thickness, carotid compliance and mean pulse pressure. All measurements were performed on a vascular ultrasound system (Acuson Corp. 128 XP ultrasound system, Mountain View, CA, USA), using a 7 MHz linear array transducer. Autonomic function was evaluated through non-invasive measurement of baroreceptor reflex sensitivity (BRS) as described by Brouwer et al. and others [Citation1,Citation17,Citation18].
Biomarkers were evaluated from overnight fasting blood samples according to standard laboratory routine. We evaluated blood glucose, insulin and insulin-like growth factor (IGF-1). We used IGF-1 z score < − 2 SD and IGF-1 < 15 nmol/l, for referral to an endocrinologist on suspicion of growth hormone deficiency (GHD). GHD was determined by a growth hormone stimulation test by the endocrinologist. Seven of eight patients were known with GHD at the study visit, and one patient was discovered at the study visit. Homeostatic model assessment insulin resistance (HOMA-IR) was performed. Renal function was measured by creatinin clearance.
Statistical analysis was performed in SPSS version 18.0 (IBM SPSS, Inc., Chicago, IL, USA). Differences between CCS and controls were evaluated through χ2-tests for categorical variables. Normality of distribution was tested through probability plots. Normally distributed variables were tested through t-tests and expressed in mean ± standard deviation, and non-normally distributed variables were tested through Mann-Whitney U-tests and expressed in median and range. Differences between groups were tested through Fisher's exact test in case > 20% of the cells showed an expected count of less than 5. Correlations were tested using Pearson's test. Two-sided p-values < 0.05 were considered significant.
Results
The initial study group consisted of 18 CCS (10 males and 8 females) who had previously received RT on the head or the neck without any other systemic anti-cancer treatment. They were matched with 19 males and 17 females in the control group (healthy siblings). Fifteen CCS had a brain tumor, one had a malignant lymphoma, one sarcoma, and one neuroblastoma. Sixteen CCS (88.9%) received RT to the head, one (5.6%) received RT to the head and neck and one (5.6%) RT to the neck. The latter subject was excluded from analysis based on RT region (only neck). Eight CCS had growth hormone deficiency. Two CCS of them who received growth hormone substitution at the time of the study echo visit were excluded from the analysis. Two more CCS had an inadequate echocardiographic window precluding echocardiographic analysis and were also excluded from analysis. After the exclusion of the five above mentioned subjects 13 CCS remained and they constituted the final study group (). Median age at evaluation was 30.8 (18.1–39.3) versus 29.2 (19.7–43.3) years (p = NS) for the sibling controls. Median follow-up time for the CCS since the RT was 21.7 (12.6–30.8) years (). The dose of irradiation varied between 40 and 68.4 Gy. Heart rate, blood pressure and body mass index were comparable in CCS and controls, but body surface area was lower in CCS ().
Table I. General characteristics, vascular structure and function and biomarkers in CCS who receive radiotherapy on the head as sole treatment and controls.
Echocardiographic results are presented in . LV mass index was comparable in CCS and controls. LV end-diastolic volume index was lower in CCS compared to the controls [56.0 (31.4–68.3) vs. 60.5 (41.9–94.3) mL/m2, p = 0.024], as was the LV stroke volume index [26.5 (19.2.–36.2) vs. 33.2 (22.0–58.8) mL/m2, p = 0.008].
Table II. Echocardiographic measurements.
Indeed, although no difference was found in LVEF, systolic LV function was decreased in CCS as compared to controls, shown by a lower s’ (5.3 ± 0.9 vs.7.1 ± 1.7 cm/s, p = 0.001) and less longitudinal deformation Ɛ (−17.3 ± 3.1 vs. −20.7 ± 2.0%, p < 0.001). Diastolic LV function was also decreased in CCS, shown by a lower e’ (−10.7 ± 1.7 vs. −12.2 ± 1.5 cm/s, p = 0.006) and early diastolic strain rate (1.1 ± 0.3 vs. 1.5 ± 0.2 1/s, p = 0.005). Furthermore, CCS showed more segments which contract after the systole has ended (post-systolic shortening) (4.9 ± 1.7 vs. 3.6 ± 1.3, p = 0.019).
Results on vascular structure and function are presented in . Both carotid compliance and baroreflex sensitivity were lower in CCS as compared to the controls but this difference was not significant.
Results on biochemical parameters are presented in . IGF-1 was lower in CCS [15.4 (9.2–34.6) vs. 24.4 (14.8–55.5) mmol/L, p = 0.007]. Moreover, in CCS a positive correlation was found between IGF-1 levels and LV end-diastolic volume index (r = 0.801, p = 0.003) as well as LV stroke volume index (r = 0.782, p = 0.04). Furthermore, IGF-1 levels in CCS correlated negatively with e’ (r = −0.752, p = 0.005) and positively with s’(r = 0.650, p = 0.022) ().
Figure 2. Left upper panel; Scatterplot representation of the positive correlation between IGF-1 (nmol/L) and left ventricular end-diastolic volume index (Ml/m2) (r = 0.801, p = 0.003). Right upper panel; Scatterplot representation of the positive correlation between IGF-1 (nmol/L) and left ventricular stroke volume index (Ml/m2) (r = 0.782, p = 0.004). Left lower panel; Scatterplot representation of the positive correlation between IGF-1 (nmol/L) and left ventricular systolic myocardial velocity (s’) (cm/s) (r = 0.650, p = 0.022). Right lower panel; Scatterplot representation of the positive correlation between IGF-1 (nmol/L) and left ventricular early diastolic myocardial velocity (e’) (cm/s) (r = -0.752, p = 0.005).
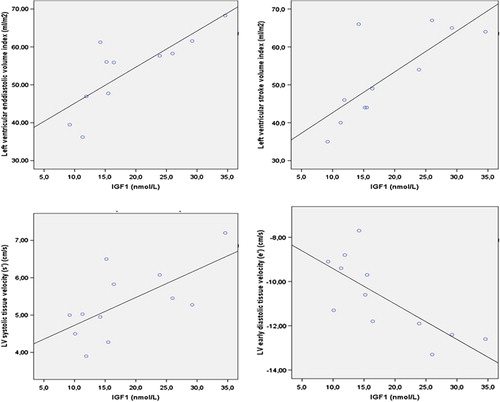
Discussion
The main and novel finding of the present study is that CCS who received RT on the head had on average smaller cardiac volumes and decreased LV systolic and diastolic function at long-term follow-up.
RT on the chest is known to have negative effects on vascular and cardiac function. Several studies provided evidence of increased myocardial fibrosis as a consequence of RT on the chest, which in turn leads to increased stiffness of the LV [Citation3,Citation4,Citation19,Citation20]. In addition, a higher incidence of coronary artery and pericardial disease [Citation3–5], as well as myocardial perfusion defects [Citation6,Citation7] was observed in CCS after RT. However, in most cases a difference in LVEF was not demonstrated [Citation2,Citation21–23], but LVEF is known to be a relatively insensitive measure of systolic myocardial LV function when subtle cardiac damage is suspected [Citation2,Citation24]. Using more subtle contemporary techniques, a decrease in systolic LV function after RT was found by Tsai et al, reporting decreased global longitudinal strain in cancer survivors who were treated with mediastinal RT (with and without additional chemotherapy) [Citation2]. Importantly however, to our knowledge there is only one study about possible cardiac effects of non-chest RT. Landy and co-workers thus recently reported in a group of CCS that cranial RT was associated with an additional decrease in LV mass and dimension compared to CCS who only received anthracyclines on top of the cranial RT [Citation25]. In addition, IGF-1 levels were lower in the CCS who had received RT but the authors did not report a correlation between the structural remodeling and IGF-1 levels. Results from our study are in line with this important paper but with more detail and additional data. First, in addition to cardiac structure, we also assessed subtle derangements of cardiac function. Second, we performed additional analyses to investigate the possible role of decreased IGF-1 levels. Third, we also considered the possible role of impaired vascular function. Moreover, as opposed to the CCS in the study by Landy and co-workers, the CCS we investigated only received RT thus circumventing possible confounding effects of anthracyclines or other systemic treatment. Like Landy and co-workers, we also observed lower end-diastolic volumes in CCS who received cranial RT compared to healthy controls. In addition, although LVEF was not lower, systolic myocardial velocities as well as systolic longitudinal myocardial deformation were reduced indicating subtle derangement of systolic function. Moreover, our study showed more post-systolic shortening in CCS. To some extent post-systolic shortening, i.e. shortening of individual segments of the myocardium beyond closure of the aortic valve is also found in healthy subjects as described by Voight et al. [Citation16]. However, increased post-systolic shortening is considered indicative of a diseased myocardium [Citation16]. Finally, we found significant differences in diastolic LV function between CCS and controls, with lower values of tissue velocities and strain rate. Taken together, it appears that RT on the head region is associated with smaller cardiac dimensions and slightly reduced systolic and diastolic LV function.
The observed cardiac effects in our analysis cannot be explained by factors like age, sex, body mass index, or differences in cardiovascular risk factors as the CCS group and the control group were well matched and no significant differences were present in general characteristics. The only difference was a lower body surface area in CCS, but the cardiac volumes were indexed for body surface area. However, we did observe differences in vascular function (albeit non-significant), shown by carotid compliance and baroreflex sensitivity which were both lower in CCS. These alterations can contribute to the difference in cardiac structure and function between the CCS and controls as was shown in preclinical study in subjects with cardiovascular risk factors [Citation10]. In that study, carotid arterial stiffness showed a strong correlation with e’ and early diastolic LV strain rate, increased stiffness being associated with reduced diastolic function. A more likely explanation for the observed cardiac abnormalities relates to the observed difference in IGF-1, as already alluded to in the study by Landy and co-workers. Hypothalamic pituitary dysfunction is a well-known side effect of cranial RT and growth hormone deficiency is frequently the only manifestation of hypothalamic pituitary dysfunction. Growth hormone and IGF-1 play a modulating role in the myocardial structure and function [Citation14]. Lower IGF-1 levels are known to be associated with cardiac dysfunction and growth, and several studies have shown that administration of IGF leads to cardiac hypertrophy [Citation26,Citation27] and increased myocardial function/performance [Citation26]. Growth hormone deficiency and its influence on cardiac structure was described by Salerno et al. [Citation27]. They described a decreased LV mass index in patients with growth hormone deficiency, which normalized after growth hormone replacement. The CCS in our study had lower IGF-1 levels compared to the controls and within the CCS group lower IGF-1 levels were associated with lower LV volumes and more reduced LV function. The data thus suggest that not only the observed structural but also functional cardiac abnormalities in the CCS might be due to impaired pituitary gland function. Supplementing growth hormone after treatment for pediatric brain tumors is not uncommon, and in our cohort 2 CCS received growth hormone substitution. They were however excluded from our analysis in order to prevent confounding.
As we studied a rather small cohort of CCS our results should be interpreted with caution. This also applies to the findings on possible underlying mechanisms, which should be seen as hypothesis generating. The results of our study need to be validated in larger cohorts to be able to reach firm conclusions. Finally, we did not measure growth hormone itself, and a low level of IGF-1 is only indirect evidence of pituitary gland dysfunction.
Conclusion
In conclusion RT to the head in CCS is associated with significant long-term structural and functional cardiac changes. A mechanism likely involved in the pathogenesis is hypothalamic pituitary dysfunction leading to growth hormone deficiency. In terms of clinical implications, decreased LV dimension is generally not considered to be problematic and diastolic function was only slightly reduced, but the combination of the two may be relevant by limiting LV filling and hence cardiac output and exercise tolerance. If anything, a strict cardiac follow-up utilizing echocardiography seems to be warranted for CCS who received RT to the head, even when the heart was not in the field of irradiation.
Declaration of interest: The authors report no conflicts of interest. The authors alone are responsible for the content and writing of the paper.
References
- Brouwer CA, Postma A, Vonk JM, Zwart N, van den Berg MP, Bink-Boelkens MT, et al. Systolic and diastolic dysfunction in long-term adult survivors of childhood cancer. Eur J Cancer 2011;47:2453–62.
- Tsai HR, Gjesdal O, Wethal T, Haugaa KH, Fossa A, Fossa SD, et al. Left ventricular function assessed by two-dimensional speckle tracking echocardiography in long-term survivors of Hodgkin's lymphoma treated by mediastinal radiotherapy with or without anthracycline therapy. Am J Cardiol 2011;107:472–7.
- Glanzmann C, Kaufmann P, Jenni R, Hess OM, Huguenin P. Cardiac risk after mediastinal irradiation for Hodgkin's disease. Radiother Oncol 1998;46:51–62.
- Adams MJ, Hardenbergh PH, Constine LS, Lipshultz SE. Radiation-associated cardiovascular disease. Crit Rev Oncol Hematol 2003;45:55–75.
- Darby SC, Ewertz M, McGale P, Bennet AM, Blom-Goldman U, Bronnum D, et al. Risk of ischemic heart disease in women after radiotherapy for breast cancer. 2013;368:987–98.
- Hardenbergh PH, Munley MT, Bentel GC, Kedem R, Borges-Neto S, Hollis D, et al. Cardiac perfusion changes in patients treated for breast cancer with radiation therapy and doxorubicin: Preliminary results. Int J Radiat Oncol Biol Phys 2001;49:1023–8.
- Prosnitz RG, Hubbs JL, Evans ES, Zhou SM, Yu X, Blazing MA, et al. Prospective assessment of radiotherapy-associated cardiac toxicity in breast cancer patients: analysis of data 3 to 6 years after treatment. Cancer 2007;110: 1840–50.
- Brouwer CA, Postma A, Hooimeijer HL, Smit AJ, Vonk JM, van Roon AM, et al. Endothelial damage in long-term survivors of childhood cancer. J Clin Oncol 2013;31:3906–13.
- Yambe M, Tomiyama H, Hirayama Y, Gulniza Z, Takata Y, Koji Y, et al. Arterial stiffening as a possible risk factor for both atherosclerosis and diastolic heart failure. Hypertens Res 2004;27:625–31.
- Miyoshi H, Mizuguchi Y, Oishi Y, Iuchi A, Nagase N, Ara N, et al. Early detection of abnormal left atrial-left ventricular-arterial coupling in preclinical patients with cardiovascular risk factors: Evaluation by two-dimensional speckle-tracking echocardiography. Eur J Echocardiogr 2011;12:431–9.
- London GM, Cohn JN. Prognostic application of arterial stiffness: Task forces. Am J Hypertens 2002;15:754–8.
- Safar ME, Levy BI, Struijker-Boudier H. Current perspectives on arterial stiffness and pulse pressure in hypertension and cardiovascular diseases. Circulation 2003;107:2864–9.
- Merchant TE, Rose SR, Bosley C, Wu S, Xiong X, Lustig RH. Growth hormone secretion after conformal radiation therapy in pediatric patients with localized brain tumors. J Clin Oncol 2011;29:4776–80.
- Colao A, Marzullo P, Di Somma C, Lombardi G. Growth hormone and the heart. Clin Endocrinol (Oxf) 2001;54: 137–54.
- Nagueh SF, Appleton CP, Gillebert TC, Marino PN, Oh JK, Smiseth OA, et al. Recommendations for the evaluation of left ventricular diastolic function by echocardiography. J Am Soc Echocardiogr 2009;22:107–33.
- Voigt JU, Lindenmeier G, Exner B, Regenfus M, Werner D, Reulbach U, et al. Incidence and characteristics of segmental postsystolic longitudinal shortening in normal, acutely ischemic, and scarred myocardium. J Am Soc Echocardiogr 2003;16:415–23.
- Nuver J, Smit AJ, Sleijfer DT, van Gessel AI, van Roon AM, van der Meer J, et al. Left ventricular and cardiac autonomic function in survivors of testicular cancer. Eur J Clin Invest 2005;35:99–103.
- Robbe HW, Mulder LJ, Ruddel H, Langewitz WA, Veldman JB, Mulder G. Assessment of baroreceptor reflex sensitivity by means of spectral analysis. Hypertension 1987;10:538–43.
- Corn BW, Trock BJ, Goodman RL. Irradiation-related ischemic heart disease. J Clin Oncol 1990;8:741–50.
- Patt DA, Goodwin JS, Kuo YF, Freeman JL, Zhang DD, Buchholz TA, et al. Cardiac morbidity of adjuvant radiotherapy for breast cancer. J Clin Oncol 2005;23: 7475–82.
- Green DM, Gingell RL, Pearce J, Panahon AM, Ghoorah J. The effect of mediastinal irradiation on cardiac function of patients treated during childhood and adolescence for Hodgkin's disease. J Clin Oncol 1987;5:239–45.
- Constine LS, Schwartz RG, Savage DE, King V, Muhs A. Cardiac function, perfusion, and morbidity in irradiated long-term survivors of Hodgkin's disease. Int J Radiat Oncol Biol Phys 1997;39:897–906.
- Morgan GW, Freeman AP, McLean RG, Jarvie BH, Giles RW. Late cardiac, thyroid, and pulmonary sequelae of mantle radiotherapy for Hodgkin's disease. Int J Radiat Oncol Biol Phys 1985;11:1925–31.
- Neilan TG, Jassal DS, Perez-Sanz TM, Raher MJ, Pradhan AD, Buys ES, et al. Tissue Doppler imaging predicts left ventricular dysfunction and mortality in a murine model of cardiac injury. Eur Heart J 2006;27:1868–75.
- Landy DC, Miller TL, Lipsitz SR, Lopez-Mitnik G, Hinkle AS, Constine LS, et al. Cranial irradiation as an additional risk factor for anthracycline cardiotoxicity in childhood cancer survivors: An analysis from the cardiac risk factors in childhood cancer survivors study. Pediatr Cardiol 2013;34:826–34.
- Duerr RL, Huang S, Miraliakbar HR, Clark R, Chien KR, Ross J, Jr. Insulin-like growth factor-1 enhances ventricular hypertrophy and function during the onset of experimental cardiac failure. J Clin Invest 1995;95:619–27.
- Salerno M, Esposito V, Spinelli L, Di Somma C, Farina V, Muzzica S, et al. Left ventricular mass and function in children with GH deficiency before and during 12 months GH replacement therapy. Clin Endocrinol (Oxf) 2004;60: 630–6.