Abstract
Purpose. The purpose of this study was to establish a feasible and convenient method for selection of the subset of patients with left-sided breast cancer for whom respiratory-gated radiotherapy (RT) would be necessary to meet the national recommendation regarding radiation dose to the heart.
Material and methods. The volume of heart receiving a dose equal to or higher than 25 Gy (V25Gy), the mean heart dose (Dmean) and total lung volume (TLV-CT) were obtained from treatment plans based on computer tomography (CT) series recorded during free breathing (FB), and the correlation between dose to the heart and TLV-CT was studied. Second, the correlation between TLV-CT and TLV defined from three pulmonary function tests (PFTs); spirometry, gas diffusion and plethysmograhy, was evaluated.
Results. Dose to the heart (V25Gy and Dmean) decreased with increasing TLV-CT. Pearson's correlation coefficient (r) for TLV-CT versus V25Gy and Dmean was equal (r = −0.809, p < 0.01) for patients planned for tangential breast RT only, and r = −0.853 and −0.861 (p < 0.01) for patients planned for loco-regional RT. Regression analysis showed good correlation between TLV-CT and TLV calculated from pulmonary function tests (R2 ≥ 0.717, p < 0.01).
Conclusion. TLV defined by routine pulmonary function tests can be used to identify the subset of left-sided breast cancer patients who require respiratory-gated RT.
Respiratory-gated radiotherapy (RT) during deep inspiration breath-hold (DIBH) significantly decreases the radiation dose to the heart in left-sided breast cancer patients [Citation1–8], without compromising the target dose. Although respiratory-gated RT is beneficial for all patients [Citation3,Citation4], the method is not always required to meet national guidelines regarding dose constraints to the heart. The guidelines vary from country to country and are changed over time according to new knowledge. The 50% isodose with cut-off at 5% heart volume inside this was used in Norway and many other countries until recently. However, after the publication of the Darby overview [Citation9], the mean heart dose has been the evaluation criterion.
Several institutions do not have the equipment to perform respiratory-gated RT and have to refer the patients to other clinics, and others cannot offer the method to all left-sided breast cancer patients due to limited resources. Therefore, there is great demand for methods that can identify the patients who would otherwise not fulfil the dose constraints for the heart.
In two earlier treatment planning studies we were able to show that respiratory-gated RT with DIBH enables achievement of national guidelines for heart dose in patients who would not meet the dose constraints in free breathing (FB) [Citation3,Citation4]. In this work we present new results based on data from these studies showing fairly good correlation between total lung volume (TLV) in FB and radiation dose to the heart, implying TLV as a selection parameter for respiratory-gated RT. The main subject of this study was to find a method that could easily estimate TLV in FB. The method should be as early as possible in the planning process, preferably before the computed tomography (CT) scan for treatment planning, to save time and staff resources by avoiding duplication, and in the view of the ALARP (as-low-as-reasonably-practicable) principle, save the patient from unnecessary radiation from a second CT scan. Such a method will also prevent delay in start-up of treatment due to repeated CT scanning and re-planning.
To our knowledge this is the first study that describes a simple and quick pulmonary function test (PFT) for estimation of TLV, which can be utilized for the selection of patients for respiratory-gated RT.
Patients and methods
This study consists of two parts; in the first part we reanalyzed data from a previous treatment planning study to identify a parameter that correlated with dose to the heart, and in the second part we describe a method, other than CT, to measure this parameter.
Total lung volume and dose to the heart
TLV-CT and dose to the heart (V25Gy and Dmean) were obtained from treatment plans based on CT series in FB from a previous study. Patients and methods are described in detail elsewhere [Citation3,Citation4]. Briefly, 16 patients with early breast cancer, referred for adjuvant RT after breast conserving surgery, were included in the study. The patients’ characteristics, referred to as group I, are summarized in . Delineation of clinical target volumes (CTV) and the heart was performed by the same oncologist for all patients, whereas the TLV was automatically generated using the auto-contouring segmentation tool of the treatment planning system (Eclipse™, Varian Medical Systems, Palo Alto, CA, USA). For study purposes two CTVs, CTV1 and CTV2, were delineated for each patient. CTV1 consisted of left mammary gland only, whereas CTV2 in addition included the following left-sided regional lymph node areas: axillary level 1–3, internal mammary glands in the intercostal spaces 1–3 and the periclavicular region. Planning target volumes, PTV1 and PTV2, were generated by adding a margin of 5 mm to the CTV's; in superficial areas the CTV and PTV were cropped when closer than 5 mm to the skin.
Table I. Patient characteristics for the two groups, group I (n = 16) and group II (n = 18). Mean ± SD and range (in brackets) are shown.
Treatment plans were made for both PTVs focusing on good PTV coverage (V95% > 98%). The Eclipse pencil beam (PB) algorithm was used for dose calculation. The prescribed dose to PTV was 50 Gy in fractions of 2 Gy. TLV-CT and dose to the heart (Dmean and V25Gy) were obtained from the dose-volume histogram (DVH) statistics and graphs.
Lung volume estimated from CT series and pulmonary function tests
Twenty consecutive patients with left-sided breast cancer referred for respiratory-gated RT between April and June 2012 were included in this study. Of these, two were excluded from the study due to unsatisfactory performance of the PFTs, leading to wrong output data from the tests. However, the two patients were treated with respiratory-gated RT, as we offer this type of treatment to all patients with left-sided breast cancer who manage to perform the DIBH technique. Unsatisfactory performance of the PFTs is not an exclusion criterion for respiratory-gated RT. Of the remaining 18 patients, 16 had undergone a breast conserving surgery (BCS) and two had a mastectomy. The characteristics of the patients, referred to as group II, are shown in . The study was approved by the regional ethics committee, and informed consent was obtained before the CT scanning and PFTs were performed.
Two CT series were acquired for each patient, one during FB and the other during DIBH. The CT series acquired during DIBH were used for clinical treatment planning; the others were used for study purposes to compare TLV delineated in CT series with TLV defined from PFTs parameters. The CT scanning was carried out in the same manner as described in our previous work [Citation3,Citation4]. The median interval between CT and PFTs was 28.5 hours (SD 132.1 hours, range 0.5–528 hours). The lungs were automatically contoured as described above and the TLV-CT was obtained by measuring the volume of the total lung delineated.
In this study we used three standard, routine PFTs: spirometry, diffusion capacity for carbon monoxide (DLCO) and whole body plethysmography. The tests were performed with the patient in a sitting position under guidance of an experienced technician, according to routine protocols. All measurements were carried out in accordance with American Thoracic Society (ATS) and European Respiratory Society (ERS) guidelines [Citation10–12]. The tests were carried out at the Section for Pulmonary Medicine and each test took about 10 minutes. The volumes and capacities we chose to extract from the PFTs and the relation between them are shown in Supplementary Figure 1 (available online at http://informahealthcare.com/doi/abs/10.3109/0284186X.2014.990107).
CT acquired during an end-expiration pause enables highly accurate estimations of the functional residual capacity, FRC [Citation13]. In our study the CT series were recorded during FB, thus including parts of the tidal volume (TV) in addition to FRC. TV is the volume of air moved into or out of the lungs during FB. To estimate the volume of TV depicted in the CT series we assume that the relative changes in the dorsoventral chest wall position during breathing as detected by the gating system, reflect the relative changes in TV. Upon reviewing the patients’ breathing curve, i.e. the dorsoventral chest wall movement recorded during CT scanning, we found that approximately 40% of the TV was depicted. This seems reasonable, as most people have a longer expiration than inspiration phase. This leads to the following relation for estimation of TLV from PFT parameters (TLV-PFT):
TLV-PFT can thus be estimated from a combination of spirometry and DLCO parameters
where IC is the inspiratory capacity, IRV is the inspiratory residual volume, VC is the vital capacity and RV is the residual volume; or by plethysmography parameters
The subscripts at the end of the PFT parameters refer to the test type; 1: spirometry, 2: DLCO and 3: plethysmography.
Statistical analysis
The correlation between TLV-CT and dose to the heart (V25Gy and Dmean) was tested using Pearson's correlation coefficient; r. p-Values less than 0.05 were considered statistically significant.
Receiver operating characteristic (ROC) curve analysis, which is a plot of the fraction of true positives out of the total actual positives [true positive rate (TPR)] versus the fraction of false positives out of the total actual negatives [false positive rate (FPR)], was used to determine cut-off values for TLV with the wanted sensitivity (equivalent to TPR) and specificity (equivalent to 1-FPR) to meet recommendations for dose to the heart (V25Gy < 5%), given different institutional limitations in staff or equipment.
Concordance between the two techniques, CT and PFTs (Equations 1–3), to estimate TLV was evaluated by linear regression analysis and Bland-Altman plots [Citation14].
The data were processed and analyzed using the statistical programs SPSS v.20 and SigmaPlot v.12.3.
Results
Patient characteristics () are equal in the two groups (p > 0.51 using independent Student's t-test).
shows V25Gy versus TLV-CT for each patient, for both PTV1 (panel A) and PTV2 (panel B), respectively, whereas the curves in panel C and D show Dmean versus TLV-CT for the same patients. All figures show decreasing dose to the heart with increasing TLV. Dose to the heart, V25Gy and Dmean, correlates fairly good with TLV-CT. Pearson's correlation coefficient, r, for TLV-CT versus V25 and Dmean, respectively, was −0.809 (p < 0.01) in both cases for PTV1, and −0.853 (p < 0.01) and −0.861 (p < 0.01) for PTV2.
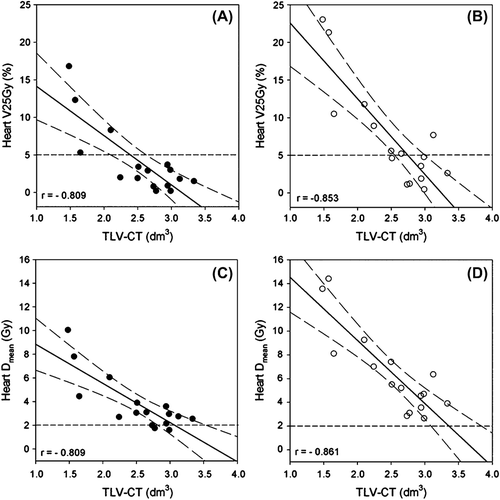
shows ROC curves for PTV1 and PTV2, with TLV-CT selected as a predictor for a heart dose exceeding the recommendations in the previous national guidelines, V25Gy > 5%. The TLV-CT value with the highest summarized value of sensitivity and specificity is 2.2 and 2.7 dm3 for PTV1 and PTV2, respectively. The sensitivity and specificity at this TLV-CT value were both equal to 1.0 for PTV1, and 0.88 for PTV2.
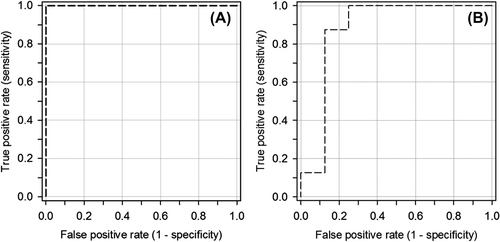
show scatterplots of TLV-CT versus TLV-PFT1 + 2 (Equation 2) and TLV-PFT3 (Equation 3), respectively. Regression analysis shows good correlation between CT and PFTs to estimate TLV, R2 was 0.717 for CT versus PFT1 + 2 and 0.753 for CT versus PFT3, respectively. Concordance between the techniques is displayed in standard Bland-Altman plots (). The mean difference (bias) between TLV-CT and TLV-PFT1 + 2 is 0.30 dm3, whereas TLV-CT versus TLV-PFT3 has a bias of 0.55 dm3. The precision (standard deviation of the difference) of TLV-CT versus TLV-PFT1 + 2 and TLV-PFT3 was 0.3 dm3 in both cases.
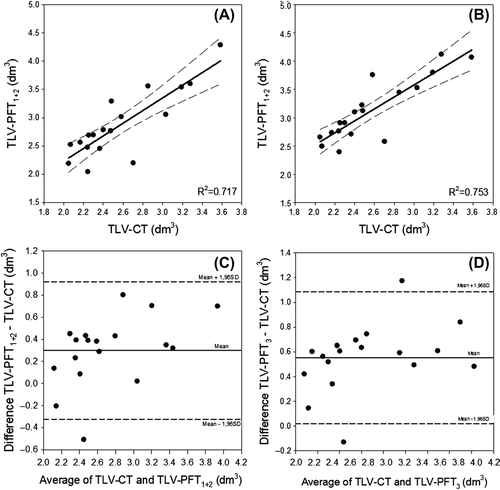
Discussion
In this study we were able to demonstrate good correlation between radiation dose to the heart (V25Gy and Dmean) and TLV, as measured in CT series acquired during FB. We were also able to demonstrate that the TLV estimated from the CT series corresponded well with the same volume calculated from parameters obtained by PFTs. The results strongly indicate that PFTs can be used for selection of patients for respiratory-gated RT, prior to the start of the treatment planning process. The PFTs are quick and easy to perform, and one single plethysmography test is enough to make a decision (Equation 3). TLV can also be calculated from parameters obtained by spirometry and DLCO (Equation 2) with nearly the same correlation. Spirometry and DLCO might be preferable in daily practice, since the equipment is far cheaper than a body plethysmograph.
As the PFTs can be done prior to the planning process, the workload on the CT scanner as well as the contouring and treatment planning task is not increased, when using the right cut-off value. The radiation dose to the patient is not increased, and the start-up of treatment is not delayed. In addition, the PFTs may also be of great value in the surveillance of patients at risk of developing radiation-induced lung injuries.
On the basis of the ROC curve analysis we would, if we were to limit the number of patients for respiratory-gated RT, choose a TLV-CT of 2.2 (breast only) and 2.7 dm3 (loco-regional treatment) as cut-off values for respiratory-gated RT, as they give the highest summarized sensitivity and specificity, thus minimizing the number of false negatives and false positives. These cut-off values may, however, be chosen differently at other institutions according to needs or limitations regarding machine- and staff resources. Setting a lower value would initially select fewer patients for respiratory-gated RT which increases the possibility of having to perform an additional CT scan in DIBH in order to meet the criteria for dose to the heart and PTV coverage. This may be the choice if the institution does not offer gated RT or the number of machines that can perform gated RT is limited and the number of false positives must be kept to a minimum. With higher cut-off values more patients would be selected for respiratory-gated RT, some of whom would also have acceptable heart doses with conventional treatment, this being an option if staff resources for CT scanning and target contouring are critical or the access to a CT scanner is limited.
A weakness of this study is the two separate patient cohorts included, which means that we are not able to show a direct correlation between the TLV-PFT and heart dose. However, similar patient characteristics within the two patient cohorts, combined with a fairly good correlation between TLV and heart dose on the one hand, and between TLV as measured with CT and the same volume measured with PFT on the other hand, strongly indicate a correlation between TLV-PFT and heart dose. Further studies, including more patients with treatment plans and PFTs for all, are necessary to quantify the correlation between TLV-PFT and heart dose, and to determine whether the results can be generalized to the whole population of patients with left-sided breast cancer. Institutions planning to use TLV as a selection criterion are recommended to establish their own data sets for heart dose versus TLV, due to institutional variations in delineation and treatment planning.
The median interval between CT and PFTs in this study was 28.5 hours. For technical reasons, two patients had a gap of 14 and 22 days, respectively, and did not complete the PFT before start of RT. However, we do not expect any changes in the TLV after only a few fractions of radiations, as such changes would occur much later (months). In addition, the difference in TLV estimated from CT and PFTs for these two patients did not differ from patients with a smaller gap.
The dose to the heart should be kept as low as possible. New guidelines in Norway take into account the work of Darby et al. [Citation9], and recommend the mean dose to the heart to be kept lower than 2 Gy [Citation15]. At the time when this study was conducted the national guidelines recommended to keep the relative volume of the heart within the 50% isodose lower than 5% (V25Gy < 5%). In this study we have shown that Dmean and V25Gy both have a good linear correlation to TLV, and V25Gy can thus easily be replaced by Dmean without changing the conclusion.
The Eclipse PB algorithm used for dose calculation is not a commonly used algorithm nowadays. From the literature and several years of experience with the Varian Anisotropic Analytical Algorithm (AAA) in our own institution, we know that the position of the 50% isodose is nearly independent of algorithm used, whereas Dmean to the heart decreases using AAA as compared to the PB algorithm [Citation16]. We find an average decrease in Dmean to the heart of 0.41 ± 0.12 Gy, when recalculating the treatment plans for group I with CTV1 as target, using AAA with fixed monitor units. This compares well with the results of Thorsen et al. [Citation17], who found a decrease in average mean heart dose of 0.36 ± 0.14 Gy when using AAA. However, the decrease is linear and the conclusion of this study is not affected by the use of AAA.
Other approaches for selection of patients for respiratory-gated RT have been reported. Qi et al. utilize 4DCT images for selection of patients for gated treatment in FB [Citation18]. This method seems both time and staff consuming, as maximum heart depth and the distance from the left ascending coronary artery to a fixed line connecting middle point of sternum and body (DLAD) have to be manually drawn and measured in three transverse CT slices for three different phases (0%, 20% and 50%) for the reconstructed 4DCT recorded during FB. The radiation dose to the patient from the 4DCT is also increased 10-fold compared to an ordinary 3DCT scan [Citation19], as opposed to the method presented in this study, where the selection of patients for respiratory-gated RT can be done prior to the planning process without additional radiation dose to the patient or extra workload for the RT staff.
In the approach described by Wang et al. [Citation20], the patient also has to be scanned twice if selected for respiratory-gated RT. The first CT scanning in FB is used to decide if the patient needs respiratory-gated RT by running a nine-minute automated planning and evaluation script. If more than 10 cm3 of the heart volume would receive more than 50% of the prescribed dose, the patient was referred for respiratory-gated RT. Subsequently, selected patients underwent repeat CT scanning with a moderate deep inspiration breath-hold (mDIBH) technique, using an Active Breathing Coordinator™ (ABC) device (Elekta).
In conclusion, TLV defined by routine PFTs can be used to identify the subset of left-sided breast cancer patients who require respiratory-gated RT.
Supplementary material available online
Supplementary Figure 1 available online at http://informahealthcare.com/doi/abs/10.3109/0284186X.2014.990107
ionc_a_990107_sm8632.png
Download PNG Image (52.3 KB)Acknowledgements
The authors are grateful to Erna Kjølsvik and Kristin Iversen for technical assistance and Ingvild Dalen for her assistance in performing and interpretation of statistical tests.
Declaration of interest: The authors report no conflicts of interest. The authors alone are responsible for the content and writing of the paper.
We thank the Norwegian Cancer Society for the financial support given towards this study.
References
- Remouchamps VM, Vicini FA, Sharpe MB, Kestin LL, Martinez AA, Wong JW. Significant reductions in heart and lung doses using deep inspiration breath hold with active breathing control and intensity-modulated radiation therapy for patients treated with locoregional breast irradiation. Int J Radiat Oncol Biol Phys 2003;55:392–406.
- Pedersen AN, Korreman S, Nystrom H, Specht L. Breathing adapted radiotherapy of breast cancer: Reduction of cardiac and pulmonary doses using voluntary inspiration breath-hold. Radiother Oncol 2004;72:53–60.
- Hjelstuen MH, Mjaaland I, Vikstrom J, Dybvik KI. Radiation during deep inspiration allows loco-regional treatment of left breast and axillary-, supraclavicular- and internal mammary lymph nodes without compromising target coverage or dose restrictions to organs at risk. Acta Oncol 2012;51:333–44.
- Vikstrom J, Hjelstuen MH, Mjaaland I, Dybvik KI. Cardiac and pulmonary dose reduction for tangentially irradiated breast cancer, utilizing deep inspiration breath-hold with audio-visual guidance, without compromising target coverage. Acta Oncol 2011;50:42–50.
- Shim JG, Kim JK, Park W, Seo JM, Hong CS, Song KW, et al. Dose-volume analysis of lung and heart according to respiration in breast cancer patients treated with breast conserving surgery. J Breast Cancer 2012;15:105–10.
- Hayden AJ, Rains M, Tiver K. Deep inspiration breath hold technique reduces heart dose from radiotherapy for left-sided breast cancer. J Med Imaging Radiat Oncol 2012;56: 464–72.
- Swanson T, Grills IS, Ye H, Entwistle A, Teahan M, Letts N, et al. Six-year experience routinely using moderate deep inspiration breath-hold for the reduction of cardiac dose in left-sided breast irradiation for patients with early-stage or locally advanced breast cancer. Am J Clin Oncol 2013;36:24–30.
- Nissen HD, Appelt AL. Improved heart, lung and target dose with deep inspiration breath hold in a large clinical series of breast cancer patients. Radiother Oncol 2013;106: 28–32.
- Darby SC, Ewertz M, McGale P, Bennet AM, Blom-Goldman U, Bronnum D, et al. Risk of ischemic heart disease in women after radiotherapy for breast cancer. N Engl J Med 2013;368:987–98.
- Miller MR, Hankinson J, Brusasco V, Burgos F, Casaburi R, Coates A, et al. Standardisation of spirometry. Eur Respir J 2005;26:319–38.
- Wanger J, Clausen JL, Coates A, Pedersen OF, Brusasco V, Burgos F, et al. Standardisation of the measurement of lung volumes. Eur Respir J 2005;26:511–22.
- MacIntyre N, Crapo RO, Viegi G, Johnson DC, van der Grinten CP, Brusasco V, et al. Standardisation of the single-breath determination of carbon monoxide uptake in the lung. Eur Respir J 2005;26:720–35.
- Graf J, Santos A, Dries D, Adams AB, Marini JJ. Agreement between functional residual capacity estimated via automated gas dilution versus via computed tomography in a pleural effusion model. Respir Care 2010;55:1464–8.
- Bland JM, Altman DG. Statistical methods for assessing agreement between two methods of clinical measurement. Lancet 1986;1:307–10.
- The Norwegian Directorate of Health [Internet]. Norwegian guideline on breast cancer treatment (in Norwegian only); 2014 [cited 2014 Nov 7]. Available from: http://helsedirektoratet.no/. 2014.
- Wieslander E, Knoos T. A virtual linear accelerator for verification of treatment planning systems. Phys Med Biol 2000;45:2887–96.
- Thorsen LB, Thomsen MS, Berg M, Jensen I, Josipovic M, Overgaard M, et al. CT-planned internal mammary node radiotherapy in the DBCG-IMN study: Benefit versus potentially harmful effects. Acta Oncol 2014;53:1027–34.
- Qi XS, Hu A, Wang K, Newman F, Crosby M, Hu B, et al. Respiration induced heart motion and indications of gated delivery for left-sided breast irradiation. Int J Radiat Oncol Biol Phys 2012;82:1605–11.
- Moorees J, Bezak E. Four dimensional CT imaging: A review of current technologies and modalities. Australas Phys Eng Sci Med 2012;35:9–23.
- Wang W, Purdie TG, Rahman M, Marshall A, Liu FF, Fyles A. Rapid automated treatment planning process to select breast cancer patients for active breathing control to achieve cardiac dose reduction. Int J Radiat Oncol Biol Phys 2012;82:386–93.