Abstract
Anatomical changes in the head-and-neck (H&N) region during the course of treatment can cause deteriorated dose distributions. Different replanning strategies were investigated for volumetric modulated arc therapy (VMAT) and intensity-modulated proton therapy (IMPT).
Material and methods. For six H&N patients two repeated computed tomography (CT) and magnetic resonance (MR) (CT1/MR1 at week 2 and CT2/MR2 at week 4) scans were acquired additionally to the initial planning CT/MR. Organs-at-risk (OARs) and three targets (CTV70Gy, CTV63Gy, CTV56Gy) were delineated on MRs and transferred to respective CT data set. Simultaneously integrated boost plans were created using VMAT (two arcs) and IMPT (four beams). To assess the need of replanning the initial VMAT and IMPT plans were recalculated on repeated CTs. Furthermore, VMAT and IMPT plans were replanned on the repeated CTs. A Demon algorithm was used for deformable registration of the repeated CTs with the initial CT and utilized for dose accumulation. Total dose estimations were performed to compare ART versus standard treatment strategies.
Results. Dosimetric evaluation of recalculated plans on CT1 and CT2 showed increasing OAR doses for both, VMAT and IMPT. The target coverage of recalculated VMAT plans was considered acceptable in three cases, while for all IMPT plans it dropped. Adaptation of the treatment reduced D2% for brainstem by 6.7 Gy for VMAT and by 8 Gy for IMPT, for particular patients. These D2% reductions were reaching 9 Gy and 14 Gy for the spinal cord. ART improved target dose homogeneity, especially for protons, i.e. D2% decreased by up to 8 Gy while D98% increased by 1.2 Gy.
Conclusion. ART showed benefits for both modalities. However, as IMPT is more conformal, the magnitude of dosimetric changes was more pronounced compared to VMAT. Large anatomic variations had a severe impact on treatment plan quality for both VMAT and IMPT. ART is justified in those cases irrespective of treatment modalities.
Intensity-modulated radiotherapy (IMRT) is the current standard of care for locally advanced head-and-neck (H&N) cancer. The advantage of IMRT is its ability to tailor the dose around the target volume in a highly conformal manner and therefore improve organs-at-risk (OARs) sparing [Citation1]. Proton therapy can even further improve treatment plan quality and thus outcome. The inverted depth dose characteristics of protons allow reducing especially the low to medium dose volumes and as a result OAR doses [Citation2,Citation3]. Several theoretical studies explored the advantages of protons over photons for H&N tumors [Citation4–6]. However, to prove the superiority of proton beam therapy certainly more prospective clinical studies are required.
One of the great challenges for fractionated radiotherapy are anatomical changes that can occur during treatment. For H&N cancer patients significant shrinkage of the target volume has been reported [Citation7]. Other potential anatomic changes are weight loss, reduction in swelling or parotid gland shrinkage [Citation8–10].
The clinical implementation of image-guided radiotherapy (IGRT) enabled to monitor and quantify these anatomical changes. Moreover, IGRT combined with IMRT contributed to improve local tumor control and to reduce acute and late complications, e.g. xerostomia or impairment of laryngeal function in case of H&N treatments [Citation11,Citation12]. Adaptive radiotherapy (ART) is expected to bring additional benefits. Repeated imaging together with deformable registration (DIR) and dose accumulation will finally help to refine current knowledge on dose response of OARs.
Several studies have been published on treatment adaptation and replanning strategies either for IMRT or proton beam therapy [Citation7,Citation13–16]. The influence of anatomical changes for proton therapy is more pronounced due to range uncertainties [Citation13,Citation17,Citation18]. According to the authors knowledge there is no study that compares changes of photon and proton dose distributions as a consequence of anatomic changes. Performing ART studies is not straightforward for several reasons, e.g. repeating computed tomographys (CTs) during the course of therapy is not commonly performed, the workload increases with ART in clinical routine and there are limitations and uncertainties of DIR algorithms. Although in photon beam therapy cone beam CTs (CBCT) have become an integral part of today's linear accelerators and enable routine IGRT and even dose adaptation. However, standard CBCT are not sufficient to recalculate the dose in proton therapy. Conventional diagnostic CTs have to be used unless CBCT is further developed, e.g. via dual energy options.
The scope of this study was to compare the need for replanning and ART strategies for H&N cancer patients between advanced photon (VMAT) and proton treatments (IMPT).
Material and methods
Image acquisition and volume delineation
Serial imaging data sets of six patients suffering from locally advanced H&N cancer of the hypopharynx or the oropharynx were utilized for this study. All patients were treated previously with IMRT and underwent chemotherapy prior to radiation therapy. In addition to imaging for treatment planning (CT0 and MR0), repeated CT (CT1, CT2) and magnetic resonance (MR) scans (MR1, MR2) were performed at weeks 2 and 4, respectively, for each patient.
All MR images were used for structure segmentation and were rigidly registered (RIR) to the respective CT for dose calculation, i.e. the GTV and OARs were delineated on MR images and later transferred to CTs. This holds for both imaging at the time of treatment planning and for biweekly examinations. One experienced radiation oncologist delineated all regions of interest (ROI) to eliminate inter-observer variability. To account for microscopic spread the GTV was isotropically expanded by 10 mm to define CTVhigh_risk. Additionally, nodal involvement and potential risk regions were defined and an intermediate as well as a low risk CTV were created. Thus, three separate PTVs (5 mm margin) were considered and for each a different dose level was prescribed [Citation19].
Treatment planning strategies
For all patients simultaneously integrated boost (SIB) plans were created using VMAT and IMPT. The first plan was based on the planning CT0, and replanning was done based on CT1 as well as CT2. SIB plans consisted of three dose levels and were assumed to be delivered in 35 fractions. Seventy gray was prescribed to the high risk planning target volume (PTV70Gy), 63 Gy to the intermediate risk PTV (PTV63Gy) and 56 Gy to the low risk PTV (PTV56Gy). Treatment plans were considered acceptable if 95% of the prescribed dose covered at least 95% of the respective PTV. For both modalities, OAR dose limits were based on the in-house applied IMRT protocol, where D2% for spinal cord and brainstem was 45 Gy and 54 Gy, respectively. Mean doses to larynx were required to be lower than 50 Gy and at least one parotid should receive a Dmean of less than 26 Gy. However, for IMPT plans, the OARs doses were pushed as low as possible while maintaining sufficient target coverage.
For VMAT the treatment planning system (TPS) MONACO (V.3.2; Elekta, USA) was used. Based on our clinical experience and practice VMAT plans with two full arcs (2 × 360°) were created, assuming 10 MV photon beams. The maximum number of control points was 150 with a minimum segment width of 0.5 cm. The dose was calculated using a Monte Carlo (MC) algorithm with a standard deviation of 3% on a grid size of 3 × 3× 3 mm3.
Proton plans were generated with the TPS XiO (V4.41; CMS/Elekta, UK), assuming an RBE of 1.1. In order to increase the robustness of the proton plans, four fields were applied (gantry angles of 40°, 140°, 220°, 320°) to avoid air cavities and to have no beams passing directly through shoulders. Dose calculation was performed with a pencil beam algorithm on a grid size of 3 × 3× 3 mm3. The initial spot sigma, defined in air, was 3 mm. The lateral spot spacing of the pencil beams was 5 mm and the distance between two layers was a magnitude of a single Bragg peak (width at 80% of the peak maximum) at the respective depth. These beam parameters were based on clinically adequate values and reported in previous studies [Citation19,Citation20].
Both VMAT and IMPT plans were planned by the same experienced medical physicist, trained in both photon and particle therapy planning, in order to avoid interobserver differences in plan quality assessment.
Assessment of the influence of anatomical variations on dose distributions
The study was divided into two parts: 1) assessment of the biweekly doses; and 2) assessment of the total “accumulated” doses for adaptive and non-adaptive scenarios.
Biweekly dose assessment
In this approach only fractions of the total dose were assessed on the planning and biweekly CTs, in order to be independent of DIR imperfections. The initial plan was recalculated on the CT1 and CT2, using the same isocenter, to evaluate how anatomical changes influenced the dose distribution. The biweekly doses were assumed to represent one third of the treatment and no dose accumulation was performed at this stage. Therefore, the initial plan based on CT0 corresponded to the dose delivered during weeks 1 and 2, then the initial plan recalculated on the CT1 corresponded to weeks 3 and 4 and finally to represent the dose delivered on weeks 5 and 6, the initial plan was recalculated on the CT2. However, biweekly dose evaluation represents only a “snapshot” of the current situation visualized on the respective CTs. In order to fully understand the effects of the anatomical variation on the total dose distribution, more sophisticated measures had to be applied.
Accumulated dose assessment
In the accumulated dose assessment two scenarios were considered. In the non-adapted scenario, where no replanning was performed, the initial plan was applied for the whole course of the treatment but anatomic variations were taken into account by dose re-calculations on CT1 and CT2. In the adapted scenario, new treatment plans were created based on CT1 and CT2. In both scenarios DIR was performed for CTs in a manually defined ROI using DIRART, a Matlab® based environment with an implemented Demon algorithm [Citation21]. The manually defined ROI was used in order to focus DIR only on the volume of interest, which was large enough to contain all target volumes and OARs, however axial slices were not trimmed. The deformation vector field was subsequently used as basis for dose accumulation for both scenarios. The detailed workflow of the dose accumulation procedure is illustrated in Supplementary Figure 1 (to be found online at http://informahealthcare.com/doi/abs/10.3109/0284186X.2015.1028590).
Evaluation of results and statistics
All results were evaluated for biweekly and dose accumulated scenarios as described above. For the biweekly dose assessment D2%(biweekly) and Dmean(biweekly) values were extracted and compared for OARs, for each patient. Then median values and respective ranges were calculated based on the six patients. The same procedure was applied to D2%(biweekly) and D98%(biweekly) values for all three PTVs.
A similar approach was used for the accumulated scenario, where the same dose indices were extracted for the initial plan, adapted and non-adapted VMAT and IMPT plans. For statistical analysis a non-parametric Wilcoxon Signed-Rank test (p < 0.05) was performed for each dosimetric parameter when comparing the adapted and non-adapted approach. However, due to small sample size the power of the test might be low, therefore rather on population, the focus is placed on the individual cases throughout the paper.
Results
Interpatient variations
summarizes the interpatient variation of GTV and CTV volumes defined on MR0 and the magnitude of the target volume shrinkage based on MR1 and MR2.
The dosimetric analysis of the initial VMAT and IMPT plans recalculated on the repeated CTs revealed target and OAR dose variations during the course of therapy. For those three patients with rather small tumor shrinkage (see patients 1, 4, 6 in ) target coverage of VMAT plans was acceptable at weeks 2 and 4, i.e. V95% was still ≥ 95%, with OAR doses still below the constraints, thus replanning was considered unnecessary. Nevertheless, the adaptation procedure was performed, in order to see if better OARs sparring can be achieved. That was not the case for IMPT plans where coverage dropped significantly. For patient 2 with the largest anatomical variations V95% was only around 30%. IMPT treatment plans had to be adapted for all patients.
shows dose distributions for both treatment modalities of the initial, non-adapted and adapted plan for two patients. The patient on the left in showed pronounced target volume shrinkage, while the patient on the right showed rather small volume changes. The corresponding quantitative dosimetric data is presented as dose volume histograms in .
Figure 2. Dose distribution for the initial plan, non-adapted plan, adapted plan, a) VMAT technique for patient with large volume shrinkage, b) IMPT technique for patient with large volume shrinkage, c) VMAT technique for patient with small volume shrinkage, d) IMPT technique for patient with small volume shrinkage, blue lines – PTV volumes, black lines – OARs, i.e. spinal cord, parotid glands.
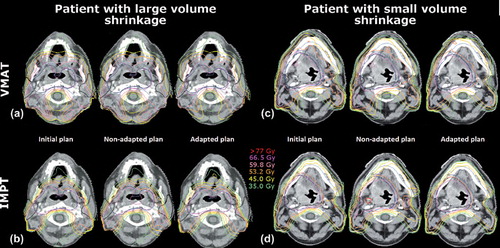
Figure 3. Dose volume histograms for patient with large volume changes a) and small volume changes b).
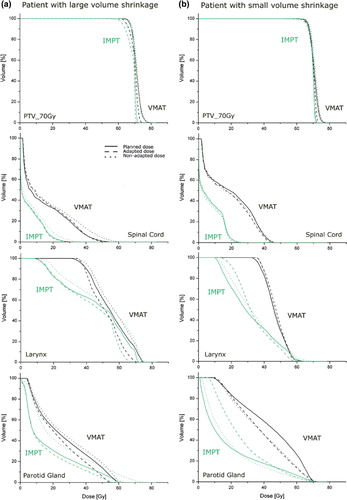
The larger anatomic variations caused an increase in the spinal cord dose for the non-adapted plan with 53 Gy values around the spinal cord for VMAT. Moreover, for IMPT large parts of the parotid gland received much higher doses compared to the initial plan. Adaptation of the plan helped to reduce the dose to spinal cord and parotids for both modalities ().
Looking at the patient with the small volume shrinkage, no significant differences were found between the initial planned dose distributions and non-adapted doses for VMAT (). However, for IMPT even small volume changes resulted again in large dose deviations. The target dose contained many hot spots, even above 77 Gy. Higher doses in the non-adaptive approach were also found for the contralateral parotid gland and the larynx, up to 74 Gy and 76 Gy, respectively (see ).
Dosimetric variations (biweekly dose evaluation)
Evaluation of biweekly doses showed an increased exposure of OARs for both VMAT and IMPT compared to the initial plan. summarizes the variation of dosimetric parameters (median values and corresponding ranges for all patients) for OARs and target structures. An extended version of that table including dosimetric parameters for other PTVs and ipsilateral parotid glands can be found in Supplementary Table I (to be found online at http://informahealthcare.com/doi/abs/10.3109/0284186X.2015.1028590).
Table I. Variation of dosimetric treatment plan parameters for OARs and PTV structures for the various treatment scenarios. Volumes are specified as median and range (min, max) for 6 patients.
For all OARs an increase of D2%(biweekly) was observed. Median D2%(biweekly) of the brainstem increased from 11.2 Gy to 14.9 Gy for VMAT, while for IMPT only an increase of 1.4 Gy was found. Similarly, D2%(biweekly) of the spinal cord increased by 1 Gy for VMAT and even by 3.3 Gy for IMPT. Dmean(biweekly) increased as well for parotid glands and larynx. For both modalities median Dmean(biweekly) increased by 2 Gy and 3 Gy in weeks 3–4 and 5–6, respectively.
For the target volumes, with VMAT no difference was observed in D2%(biweekly). IMPT was much more sensitive and D2%(biweekly) increased up to 3 Gy in following weeks. Differences in D98%(biweekly) were again more pronounced for IMPT compared to VMAT with IMPT median D98%(biweekly) decreased by 2.5 Gy in weeks 5–6.
Dose accumulation: adaptation versus non-adaptation
For both scenarios, i.e. adapted and non-adapted, significant differences were found compared to the initial plan. Patient averaged D2% and Dmean doses for all OARs and averaged D2% and D98% for three target volumes are summarized in . An extended version of that table including dosimetric parameters for other PTV and ipsilateral parotid glands can be found in Supplementary Table II (to be found online at http://informahealthcare.com/doi/abs/10.3109/0284186X.2015.1028590).
Table II. Variation of dosimetric treatment plan parameters for OARs and PTV structures in biweekly intervals. Volumes are specified as median and range (min and max) for 6 patients.
For the spinal cord and brainstem the dosimetric differences between the adaptive and non- adaptive approach were generally small for VMAT, while they were statistically significant for D2% doses in case of IMPT. In the adapted scenario median Dmean for parotid glands and the larynx were also lower, but not statistically significant for neither of the modalities. The D2% difference for target volumes between adapted and non-adapted strategies was found to be statistically significant for both modalities, e.g. for PTV70Gy it decreased by more than 4.4 Gy for IMPT.
D98% for VMAT did not vary much between the adapted and non-adapted treatment concepts, except for PTV70Gy where the median D98% decreased after adaptation. For IMPT D98% values increased in general after plan adaptation, however without statistical significance.
Discussion
The study indicated the importance of repeated imaging in fractionated radiotherapy for H&N cancer. Biweekly dose evaluation was considered to be adequate to quantify the difference in dosimetric parameters for target structures and OARs. When applying the initial plan on the repeated CT obtained at week 2 and 4, increased OAR doses were found paired with deteriorated target dose conformity. Nevertheless, in half of the patients, treatment plan parameters were still acceptable with VMAT (V95% > 95%, OARs within limits) after recalculating the initial plan on the updated CTs. Treatment plan adaptation, performed for those patients, optimized OARs sparing even further. For IMPT none of the recalculated plans was found to be acceptable in terms of target coverage, since even small anatomic variations had a large impact on the resulting dose distributions.
A 5 mm expansion from each CTV was applied to construct PTVs, for both modalities, which is taken from our photon experience. For proton therapy there are different concepts for PTV construction, ranging from beam specific margins, anisotropic margins or more sophisticated methods including robust optimization. However, there are proton facilities which use 5 mm margin expansion for H&N cancer patients in clinical routine [Citation6,Citation15]. Finding the best margin strategy was outside the scope of the study, because we intended to compare directly proton plans with VMAT plans under similar conditions, i.e. we wanted to evaluate the magnitude of dose degradation for the same volume changes.
Even though the magnitude of target coverage changes was large for proton plans, OAR dose limits were never exceeded, even not in the non-adapted approach. This can be explained by the superior initial OARs sparing for IMPT. For VMAT plans OAR doses in the initial plans were very often close to planning constraints and therefore even a small dose increase violated the planning aims. Consequently, it is not straightforward to translate adaptive approaches from the photon experience towards proton therapy. Therefore, it is essential to explore ART for protons separately.
This study was based on two rescans in two weekly intervals. Repetitive CTs on a daily/weekly basis could provide even more information about anatomical variations. At present there is no consensus on how many image series needed to be taken during the course of treatment or how often replanning should be executed. Obviously, if repeated imaging is utilized for ART, the extra dose coming from additional imaging, cannot be neglected [Citation22]. Wu et al. considered different replanning schemes for IMRT and concluded that replanning more often than once a week is unnecessary [Citation7]. However, this might not be valid for IMPT.
The deformation of the CT images was performed in the anti-chronological way, which means that CT1 and CT2 were registered to CT0 and the accumulated dose was projected to CT0. Chronological image deformation and dose accumulation onto the last CT would bring slightly different results due to the difference between the deformed structure and the actual structure drawn by the radiation oncologist on repeated CTs. However, if delineated and deformed structures would be identical no difference should be found between the chronological and anti-chronological dose summation. Nevertheless, using deformed structures without inspection and adaptation by an experienced radiation oncologist is not possible, as current state-of-the-art DIR algorithms are not robust enough. It is still under discussion which summation method should be used [Citation15,Citation23].
The results presented were obtained with a voxel intensity-based DIR algorithm that was applied in other ART studies [Citation24–27]. Nevertheless, the accumulated dose reported above might not be the “true” dose and is rather the best estimation of the true dose. The general and qualitative trends on the differences between VMAT and IMPT in the context of ART described in the present study will not be influenced by an improved dose accumulation process. However, the study is limited by the assumptions that systematic inaccuracies related to DIR have the same order of magnitude for both modalities. As there are different dose gradients towards OAR between photon and proton therapy this needs to be further investigated. The authors appreciate that DIR algorithm validation is certainly a large research topic on its own and detailed investigations in this direction were beyond scope of this study.
Although conformal VMAT and IMPT dose distribution were created at the time of treatment planning based on CT0, recalculating a plan on the repeated CTs (CT1 or CT2) with a different, i.e. smaller, target volume, resulted in a less conformal dose distribution, but with sufficient coverage. For the ART approach, new plans, designed especially for the smaller target, were highly conformal and with high coverage on each repeated CT. After DIR and dose accumulation the smaller target did not exactly reach the initial target size, therefore after dose summation the target coverage (for some cases) was lower compared to non-adapted plans. This is also reflected in decreased D98% values for the target structures. This imperfect behavior of current DIR algorithms with respect to changing tumor volume is a general limitation of ART. For OARs volume changes are less pronounced than for target structures, and consequently assessing the total OAR dose picture in ART by dose accumulation deems to be warranted.
In summary, the assessment of the dosimetric consequences of anatomical variations underlines the benefits of adaptive strategies for both photon and proton therapy. However, the advantages of ART were largely patient dependent. Moreover, the same anatomical variations resulted in a very different impact on dose distributions for IMPT and VMAT. Therefore, the clinical implementation of ART needs to be adjusted to needs of the underlying treatment modality.
Supplementary material available online
Supplementary Figure 1 and Tables I-II to be found online at http://informahealthcare.com/doi/abs/10.3109/0284186X.2015.1028590
ionc_a_1028590_sm1463.pdf
Download PDF (386.8 KB)Acknowledgements
The financial support by the Federal Ministry of Science, Research and Economy and the National Foundation for Research, Technology and Development and The European Community’s Seventh Framework Programme (FP7 2007–2013) under Grant Agreement No. 215849-2 (Project PARTNER) is gratefully acknowledged.
Declaration of interest: The authors report no conflicts of interest. The authors alone are responsible for the content and writing of the paper.
References
- Kristensen CA, Kjaer-Kristoffersen F, Sapru W, Berthelsen AK, Loft A, Specht L. Nasopharyngeal carcinoma. Treatment planning with IMRT and 3D conformal radiotherapy. Acta Oncol 2007;46:214–20.
- Mendenhall NP, Malyapa RS, Su Z, Yeung D, Mendenhall WM, Li Z. Proton therapy for head and neck cancer: Rationale, potential indications, practical considerations, and current clinical evidence. Acta Oncol 2011;50:763–71.
- Thörnqvist S, Muren LP, Bentzen L, Hysing LB, Høyer M, Grau C, et al. Degradation of target coverage due to inter-fraction motion during intensity-modulated proton therapy of prostate and elective targets. Acta Oncol 2013;52:521–7.
- Lomax AJ, Bohringer T, Bolsi A, Coray D, Emert F, Goitein G, et al. Treatment planning and verification of proton therapy using spot scanning: Initial experiences. Med Phys 2004;1:3150–7.
- Steneker M, Lomax A, Schneider U. Intensity modulated photon and proton therapy for the treatment of head and neck tumors. Radiother Oncol 2006;80:263–7.
- Frank S, Cox J, Gillin M, Mohan R, Garden AS, Rosenthal DI, et al. Multifield optimization intensity modulated proton therapy for head and neck tumors: A translation to practice. Int J Radiat Oncol Biol Phys 2014;89:846–53.
- Wu Y, Chi P, Chen Q, Krauss DJ, Yan D, Martinez A. Adaptive replanning strategies accounting for shrinkage in head and neck IMRT. Int J Radiat Oncol Biol Phys 2009;75:924–32.
- Elstrøm UV, Wysocka BA, Muren LP, Petersen JB, Grau C. Daily kV cone-beam CT and deformable image registration as a method for studying dosimetric consequences of anatomic changes in adaptive IMRT of head and neck cancer. Acta Oncol 2010;49:1101–8.
- Hansen EK, Bucci MK, Quivey J, Weinberg V, Xia P. Repeat CT imaging and replanning during the course of IMRT for head-and-neck cancer. Int J Radiat Oncol Biol Phys 2006;64:355–62.
- Barker Jr JL, Garden AS, Ang KK, O’Daniel JC, Wang H, Court LE, et al. Quantification of volumetric and geometric changes occurring during fractionated radiotherapy for head-and-neck cancer using an integrated CT/linear accelerator system. Int J Radiat Oncol Biol Phys 2004;59:960–70.
- Nguyen NP, Ceizyk M, Vos P, Vinh-Hung V, Davis R, Desai A, et al. Effectiveness of image-guided radiotherapy for laryngeal sparing in head and neck cancer. Oral Oncol 2010;46:283–6.
- Wu Q, Mohan R, Morris M, Lauve A, Schmidt-Ullrich R. Simultaneous integrated boost intensity-modulated radiotherapy for locally advanced head-and-neck squamous cell carcinomas. I: Dosimetric results Int J Radiat Oncol Biol Phys 2003;56:573–85.
- Lomax AJ. Intensity modulated proton therapy and its sensitivity to treatment uncertainties 1: The potential effects of calculation uncertainties. Phys Med Biol 2008;53:1027–42.
- Schwartz DL, Garden AS, Thoma J, Chen Y, Zhang Y, Lewin J, et al. Adaptive radiotherapy for head-and-neck cancer: Initial clinical outcomes from a prospective trial. Int J Radiat Oncol Biol Phys 2012;83:986–93.
- Berwouts D, Olteanu L, Duprey F, Vercauteren T, De Gersem W, De Neve W, et al. Three-phase adaptive dose-painting-by-numbers for head-and-neck cancer: Initial results of the phase I clinical trial. Radiother Oncol 2013;107:310–6.
- Simone C, Ly D, Dan T, Ondos J, Ning H, Belard A, et al. Comparison of intensity-modulated radiotherapy, adaptive radiotherapy, proton radiotherapy, and adaptive proton radiotherapy for treatment of locally advanced head and neck cancer. Radiother Oncol 2011;101:376–82.
- Kraan AC, van de Water S, Teguh DN, Al-Mamgani A, Madden T, Kooy HM, et al. Dose uncertainties in IMPT for oropharyngeal cancer in the presence of anatomical, range, and setup errors. Int J Radiat Oncol Biol Phys 2013;87:888–96.
- Hopfgartner J, Stock M, Knäusl B, Georg D. Robustness of IMPT treatment plans with respect to inter-fractional set-up uncertainties: Impact of various beam arrangements for cranial targets. Acta Oncol 2013;52:570–9.
- Góra J, Hopfgartner J, Kuess P, Paskeviciute B, Georg D. Is there room for combined modality treatments? Dosimetric comparison of boost strategies for advanced head and neck and prostate cancer. J Radiat Res 2013;54(Suppl 1):i97–112.
- Góra J, Stock M, Lütgendorf-Caucig C, Georg D. Is there an advantage in designing adapted, patient-specific PTV margins in intensity modulated proton beam therapy for prostate cancer? Int J Radiat Oncol Biol Phys 2013;85:881–8.
- Deshan Y, Scott B, Issam EN, Aditya A, Wu Y, Goddu SM, et al. Technical Note: DIRART – A software suite for deformable image registration and adaptive radiotherapy research. Med Phys 2011;38:67.
- Stock M, Palm A, Altendorfer A, Steiner E, Georg D. IGRT induced dose burden for a variety of imaging protocols at two different anatomical sites. Radiother Oncol 2012; 102:355–63.
- Vercauteren T, De Gersem W, Olteanu L, Madani I, Duprez F, Berwouts D, et al. Deformation field validation and inversion applied to adaptive radiation therapy. Phys Med Biol 2013;58:5269–86.
- Nithiananthan S, Brock K, Daly M, Chan H, Irish JC, Siewerdsen JH. Demons deformable registration for CBCT-guided procedures in the head and neck: Convergence and accuracy. Med Phys 2009;36:4755–64.
- Castadot P, Geets X, Lee J, Christian N, Grégoire V. Assessment by a deformable registration method of the volumetric and positional changes of target volumes and organs at risk in pharyngo-laryngeal tumors treated with concomitant chemo-radiation. Radiother Oncol 2010;95: 209–17.
- Zambrano V, Furtado H, Fabri D, Lütgendorf-Caucig C, Góra J, Stock M, et al. Performance validation of deformable image registration in the pelvic region. J Radiat Res 2013; 54(Suppl 1):i120–8.
- Fabri D, Zambrano V, Bhatia A, Furtado H, Bergmann H, Stock M, et al. A quantitative comparison of the performance of three deformable registration algorithms in radiotherapy. Z Med Phys 2013;23:279–90.