ABSTRACT
Background. Conventionally fractionated radiotherapy (CFRT) has proven ineffective in treating non-small cell lung cancer while more promising results have been obtained with stereotactic body radiotherapy (SBRT). Hypoxic tumours, however, might present a challenge to extremely hypofractionated schedules due to the decreased possibility for inter-fraction fast reoxygenation. A potentially successful compromise might be found in schedules employing several fractions of varying fractional doses. In this modelling study, a wide range of fractionation schedules from single-fraction treatments to heterogeneous, multifraction schedules taking into account repair, repopulation, reoxygenation and radiosensitivity of the tumour cells, has been explored with respect to the probability of controlling lung tumours.
Material and methods. The response to radiation of tumours with heterogeneous spatial and temporal oxygenation was simulated including the effects of accelerated repopulation and intra-fraction repair. Various treatments with respect to time, dose and fractionation were considered and the outcome was estimated as Poisson-based tumour control probability for local control.
Results. For well oxygenated tumours, heterogeneous fractionation could increase local control while hypoxic tumours are not efficiently targeted by such treatments despite reoxygenation. For hypofractionated treatments employing large doses per fraction, a synergistic effect was observed between intra-fraction repair and inter-fraction fast reoxygenation of the hypoxic cells as demonstrated by a reduction in D50 from 53.3 Gy for 2 fractions to 52.7 Gy for 5 fractions.
Conclusions. For well oxygenated tumours, heterogeneous fractionation schedules could increase local control rates substantially compared to CFRT. For hypoxic tumours, SBRT-like hypofractionated schedules might be optimal despite the increased risk of intra-fraction repair due to a synergistic effect with inter-fraction reoxygenation.
Radiobiological modelling enables the exploration of various treatment scenarios with respect to physical parameters, such as treatment time, dose and fractionation, as well as the radiobiological properties of both tumour and normal tissues. While the clinical interpretation of the results of such model calculations is highly dependent on the model and choice of parameters, the relative impact of various processes can be qualitatively studied and assessed. For example, the impact of both radiosensitivity and reoxygenation has previously been studied [Citation1–3] by simulating and mathematically taking into account the spatial and temporal patterns of tumour oxygenation for calculations of cell survival. In order to perform a more comprehensive radiobiological evaluation of a wide range of treatment schedules with respect to the overall treatment time, dose and fractionation, the remaining three Rs of radiobiology – repair, redistribution and repopulation – need to be considered as well [Citation4].
While the inter-fraction repair of sublethal damage is eloquently described by the α/β-ratio in the linear-quadratic (LQ) model [Citation5], treatments with large doses per fraction or complicated beam arrangements might take long enough to also allow for repair during the delivery of one fraction. Depending on the overall delivery time from the first beam-on time to the last, the intra-fraction repair might need to be seriously considered in relation to the success of the treatment. On the other end of the fractionation spectrum, multifraction treatments lasting for several weeks and thus exceeding the kick-off time for accelerated repopulation might compromise the treatment outcome as well [Citation4]. In the search for an optimum manner of delivering radiotherapy, such aspects need to be taken into account.
In this study, a previously used tumour model was further developed to include the effects of intra-fraction repair and accelerated repopulation in addition to modelling the tumour oxygenation and its impact on the radiosensitivity. Furthermore, in the pursuit of an optimal fractionation strategy, schedules employing heterogeneous doses per fraction have also been considered.
Method and materials
The study was performed based on a previously used in silico model originally derived from oxygenation calculations from experimentally obtained inter-vessel distance distributions [Citation6,Citation7]. Voxelised three-dimensional (3D) models can thus be created to simulate tumours with heterogeneous oxygenation including the possibility to model both fast and slow reoxygenation as previously described [Citation6,Citation8–10]. In this study, the model was further developed to include the effects of intra-fraction repair, accelerated repopulation and heterogeneous fractionation by modifying the LQ expression for surviving fraction accordingly.
Thus, in the original model, tumours with heterogeneous and dynamic oxygenations were simulated and the survival was assessed on voxel level using the LQ model and incorporating the dose-modifying effect of hypoxia [Citation11]. The basic LQ- expression for surviving fraction SF after a dose d was hence modified to include oxygen modifying factors (OMFs) depending on the local oxygen tension (pO2) and calculated on voxel level [Citation8,Citation12]:
To study the effect of intra-fraction repair, the expression for survival in Equation 1 was further modified as [Citation13]:
where g is a function of the repair rate μ and the time t it takes to deliver one fraction:
The repair rate μ is calculated as ln(2)/trepair where trepair is the half-time of the repair of sublethal damage. A dose-rate of 2 Gy per minute and a repair half-time of 15 minutes were assumed in the present simulations corresponding to a fast component of repair [Citation14].
For treatments lasting for several weeks, it is expected that an accelerated repopulation of the tumour cells is going to take place for the latter part of the treatment. To take this effect into account, the survival is instead calculated as:
where Tpot is the potential doubling time of the clonogenic cells, T is the overall treatment time and TK is the kick-off time for accelerated repopulation [Citation4]. In this study, Tpot was assumed to be 5 days and TK 14 days [Citation15], corresponding to a loss of 0.4 Gy per day.
As an alternative to both extremely hypofractionated SBRT and conventionally fractionated radiotherapy (CFRT), a different schedule could be considered in which an escalated dose per fraction is delivered on days preceding or following a short interruption of the treatment as represented by the weekends. To simulate such a heterogeneous fractionation schedule, Equation 4 was further modified as:
where fbase and fesc are the frequencies of fractions delivering a baseline dose dbase and an escalated dose desc respectively. The escalated doses per fraction desc were simulated to be delivered on maximally two days per week depending on the overall number of fractions.
Two different heterogeneous oxygen distributions were considered for the simulated tumours in this work. On one hand, an overall well oxygenated tumour was simulated resulting from a mean inter-vessel distance (IVD) of 100 μm, and leading to a hypoxic fraction (HF) less than 5 mmHg of < 1%. On the other hand, a hypoxic tumour with a core of 12 mm (with a mean IVD of 160 μm) surrounded by a less hypoxic external rim (mean IVD of 110 μm) was also simulated. The hypoxic tumour had a resulting HF of about 21%. All modelled tumours had a diameter of 20 mm, corresponding to a volume of 4.2 cm3.
Both static and dynamic oxygenation patterns were considered, either assuming that the oxygen distribution was constant throughout the treatment (no reoxygenation) or that it changed between fractions (reoxygenation). For schedules employing up to 10 fractions, only local changes in the oxygen distribution were considered by randomly redistributing the oxygen tension values without changing the magnitude of the hypoxic fraction. This corresponds to the fast reoxygenation of acutely hypoxic cells. For the hypoxic tumour, shrinkage in the radius of the more hypoxic core of 0.4 mm per fraction was also simulated at every fraction following the 10th fraction, simulating slow reoxygenation coinciding with the onset of accelerated repopulation.
The LQ-model modified according to Equations 1, 2 and 5 was used to calculate cell response on voxel level in simulated tumours with heterogeneous and dynamic oxygenations using an α of 0.35 Gy−1 and an α/β of 10 Gy [Citation15,Citation16]. Overall tumour-response was estimated by calculating the tumour control probability (TCP) using a Poisson-based expression [Citation4]:
where N is the number of clonogenic cells (set to 108) and SF is the surviving fraction given by either Equations 1, 2 or 5. Dose-response curves were generated by systematically increasing the total dose and fitting a logit expression [Citation17] to the data points:
where D50 is the dose required to achieve 50% TCP, γ is the slope of the dose-response curve at D50, and D is the total dose delivered in the fractionated regime.
Results
In , the impact of intra-fraction repair is demonstrated by a higher value of the dose required for 50% TCP (D50) compared to the case of no repair for all schedules. This effect is mostly pronounced for the single-fraction schedules where there is a difference in D50 of 6.5 Gy between repair and no repair. At 10 fractions the corresponding difference has been reduced to 1.1 Gy as a result of the lower dose per fraction. In the case of no reoxygenation, the D50 increases with the increasing number of fractions both with and without intra-fraction repair; this can also be seen in the dose-response curves in . Thus, the impact of inter-fraction repair is not substantially counteracted by the decrease in intra-fraction repair when increasing the number of fractions. However, the overall difference in D50 between the 10-fractions schedule and the single-fraction schedule is 38.5 Gy with intra-fraction repair, and 43.9 Gy assuming no repair during treatment. This demonstrates the relative benefit from employing multiple fractions given the decreased possibility for intra-fraction repair which is mostly pronounced when going from 1 to 2 fractions. In the case of intra-fraction repair, an increase in D50 of 10.7 Gy is required, compared to 12.8 Gy without repair.
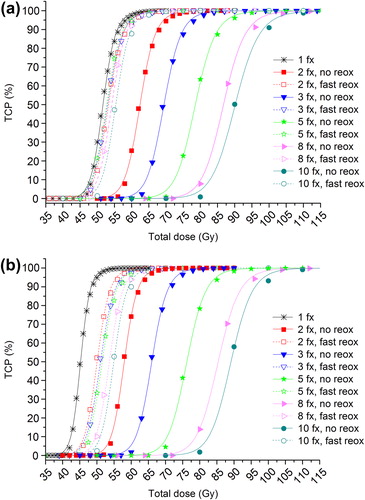
Table I. D50 values for SBRT treatments with and without intra-fraction repair.
Taking into account an oxygenation that changes locally between fractions as a result of fast reoxygenation, the D50 is substantially reduced for all fractionation schedules considered as shown in and illustrated by the dashed curves in . Furthermore, it is interesting to note that if intra-fraction repair is considered, the D50 with reoxygenation de facto decreases when going from 2 to 5 fractions and then increases at 8 and 10 fractions. This phenomenon is only seen when intra-fraction repair is included () and thus demonstrates a synergistic effect of inter-fraction reoxygenation and intra-fraction repair.
The dose-response curves in indicate that a slight acceleration of the treatment by partly escalating the fractional dose could be beneficial with respect to local control for well-oxygenated tumours. For example, if a total dose of 60 Gy will be delivered in 30 equal fractions of 2 Gy, the overall treatment time if one fraction is delivered per day, five days a week, is 40 days. Assuming no reoxygenation, the corresponding TCP is 32%. If a total dose of 60 Gy will be delivered in 9 fractions of 4 Gy and 12 fractions of 2 Gy, the overall treatment time is only 28 days and the corresponding TCP is almost 100%. Thus, schedules with a baseline fractional dose of 2 Gy accompanied by an escalation to 3 or 4 Gy per fraction for a maximum of two out of five days per week lead to an improved response compared to applying 2 Gy-fractions only. In fact, the heterogeneous schedules with no reoxygenation perform better than the homogeneous fractionation schedules assuming fast reoxygenation.
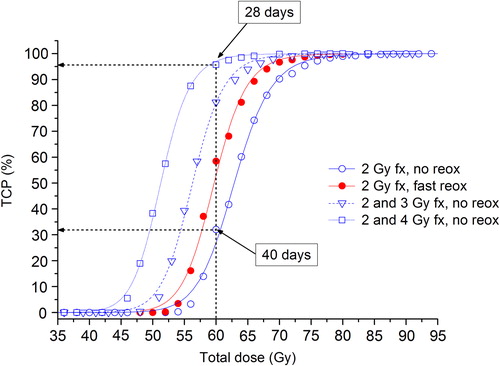
If instead the hypoxic tumour is treated with a heterogeneous schedule, the dose-response curves (dotted lines) in are obtained, displayed together with the dose-response curves from assuming intra-fraction repair. If only fast reoxygenation is assumed to take place during the heterogeneous treatment, the resulting D50 is 67.6 Gy. As such schedules correspond to treatments that extend for several weeks it is reasonable to assume that some slow reoxygenation of chronic hypoxia also takes place. However, even under the rather optimistic assumption of a slow reoxygenation pattern corresponding to shrinkage in the radius of the hypoxic island of 0.4 mm at every fraction after the 10th fraction, the D50 is only reduced to 61.0 Gy, performing slightly better than the 2-fractions schedule assuming no reoxygenation.
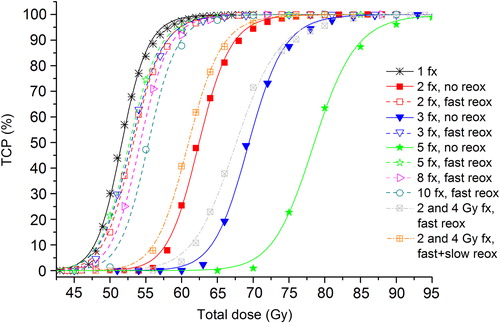
Discussion
The five Rs of radiobiology have long been used in the search of optimum fractionations aiming at maximising the response of tumours to fractionated radiotherapy while minimising the effects of the normal tissues. Nevertheless, the recent years saw an increased interest in hypofractionated treatments making use of advanced imaging and radiation delivery techniques capable of minimising the volume of normal tissue included in the regions receiving therapeutic doses. This is the case of highly conformal or stereotactic treatments limiting the red shell of normal tissue, while delivering increasing doses to the tumours [Citation18]. Thus, while the objective is usually formulated as obtaining local control under the constraints of normal tissue complication probability (NTCP), it could also be hypothesised that the trade-off between the five Rs with respect to their impact on local control represents an optimisation problem in itself. Under the assumption that the NTCP is not jeopardised, the mutual relationship between these processes could thus give an indication of an optimal treatment. While changes in any treatment protocol must be motivated by clinical evidence, there are substantial limitations with respect to what type of clinical trials – or even animal experiments – can be designed, not least from an ethical point of view. Thus, the kind of experimenting with time, dose and fractionation that is required in order to embark on the journey towards an optimal fractionation could almost exclusively be explored by radiobiological modelling. In this study, model parameters for NSCLC have been used, but the same methodology is applicable to any tumour type given relevant parameters. Furthermore, the parameter values for the kick-off time, potential doubling time and repair half-time was assumed to be relatively short (14 days, five days and 15 minutes respectively). This rather extreme choice of parameters was deliberately made in order to study the impact of the individual processes and also to put the results on the safe side with respect to achieving tumour control. However, for this type of study, the results are even less dependent on the specific choice of parameter values and their inherent uncertainties as the emphasis is on the relative impact of different processes on treatment outcome rather than analysing absolute numerical results alone. Nevertheless, it has to be mentioned that although the main aim of the study was not the comparison of the predictions of the model with the actual clinical data, the current results support the previous findings of the study by Lindblom et al. [Citation3] showing that the clinical outcome of hypofractionated SBRT treatments is better predicted when fast reoxygenation is taken into account.
CFRT has generally failed to achieve satisfying rates of local control in non-small cell lung cancer [Citation19] while stereotactic treatments as introduced in the 1990s for extra-cranial sites by Blomgren and Lax [Citation20,Citation21] has achieved promising results [Citation22]. While offering a higher biological effectiveness and avoiding the problems associated with accelerated repopulation, it is however reasonable to question whether such hypofractionated schedules are efficient enough in targeting hypoxic tumours given the limited time for inter-fraction fast reoxygenation. Thus, if hypoxia is the dominant factor influencing the treatment outcome, one might still have to consider multifraction treatments. Furthermore, a higher dose per fraction or more complicated irradiation set-ups as might be required in e.g. SBRT will inevitably increase the risk of intra-fraction repair, potentially contributing to a loss of local control.
The many processes that need to be factored into the alteration or design of a new radiotherapy fractionation schedule hence often have opposite impact on the probability to achieve local control. Therefore, it is not a priori straightforward to predict the outcome of e.g. a reduced number or fractions or a partial escalation of the fractional dose based on educated guessing alone. For this purpose, radiobiological modelling using clinically derived parameters offers a powerful tool.
For example, when the number of fractions is increased, an accompanying increase in the total dose is expected in order to achieve the same level of tumour control due to the inter-fraction repair of sublethal damage. However, it was previously demonstrated that such inter-fraction repair can be effectively counteracted by the fast reoxygenation of hypoxic cells, also taking place between fractions [Citation1,Citation3]. Furthermore, in the present study it was found that fast reoxygenation not only counteracts inter-fraction repair but it also acts synergistically with the reduction in intra-fraction repair for schedules employing 2–5 fractions. Thus, the combined effect when going from 2 to 3 or 5 fractions from the reduction in intra-fraction repair and the increased possibility for inter-fraction reoxygenation leads to an overall reduction in total dose for an isoeffect despite employing more fractions. This reduction in D50 is however limited to these schedules as there is a shift towards higher doses for the 8-fractions schedule which is further pronounced at 10 fractions. The fact that this effect is limited to a certain fractionation range suggests that there might be an optimal number of fractions with respect to the trade-off between a reduced negative impact on local control of repair and a positive impact of reoxygenation for a slightly larger number of fractions.
While the doses required for the 2-fractions schedule might compromise the normal tissue tolerance, as long as the red shell of normal tissue is of less concern, these results could suggest that an optimal treatment schedule for hypoxic NSCLC might be found between 3 and 5 fractions as is delivered in several SBRT treatments [Citation22]. Such schedules would correspond to enough inter-fraction reoxygenation while still delivering a highly effective treatment from a radiobiological point of view.
Heterogeneous fractionation has only been found as a competitive alternative in the case of an overall well-oxygenated tumour. For the hypoxic tumour, a schedule employing 2- and 4 Gy-fractions could not compete with the SBRT-like schedules in terms of achieving high levels of TCP despite considering a rather optimistic decrease in the hypoxic fraction during treatment. This is another example of a result that is perhaps not so intuitive, albeit evident from the present simulations.
In this work, the LQ model has been used to predict cell survival for doses per fraction exceeding what has been quoted as suitable given the discrepancy between in vitro survival data and the continuous bend of LQ survival curve [Citation23]. However, in all the calculations the oxygen tension has been mathematically taken into account as this has been shown to extend the validity of the LQ model over a much larger dose range compared to one of its proposed alternatives [Citation2].
An impact of redistribution of tumour cells within the cell cycle has not been included in the model calculations. At moderate doses per fraction, tumour cells are likely to become arrested in the cell cycle and die by mitotic death, while at the higher, SBRT-like doses per fraction they are likely to die from apoptosis [Citation24]. In either case, any effect of redistribution within the cycle is not expected and it has therefore been omitted in this work.
In relation to the effect of the time point of irradiation, the simulation of the heterogeneous fractionations has not included any modelling of the particular repair status of the tumour cells with respect to when the escalated fractions were delivered. While the relative benefit of heterogeneously fractionated schedules compared to conventionally fractionated treatments might have been observed if this had been included, it would however not lead to a better outcome in terms of the TCP. Nevertheless, in spite of these limitations, the present results have shown the potential of theoretical modelling in optimising fractionated radiotherapy before costly and lengthy experiments or clinical trials could be initiated.
In conclusion, heterogeneously fractionated schedules only proved to be effective in well-oxygenated tumours. Hypofractionated treatments of hypoxic tumours can however exhibit a synergistic effect from inter-fraction fast reoxygenation and intra-fraction repair of sublethal damage for a limited fraction range, indicating the existence of an optimal number of fractions to maximise the response of NSCLC.
Declaration of interests: The authors report no conflicts of interest. The authors alone are responsible for the content and writing of the paper.
References
- Antonovic L, Lindblom E, Dasu A, Bassler N, Furusawa Y, Toma-Dasu I. Clinical oxygen enhancement ratio of tumors in carbon ion radiotherapy: The influence of local oxygenation changes. J Radiat Res 2014;55:902–11.
- Lindblom E, Dasu A, Lax I, Toma-Dasu I. Survival and tumour control probability in tumours with heterogeneous oxygenation: A comparison between the linear-quadratic and the universal survival curve models for high doses. Acta Oncol 2014;53:1035–40.
- Lindblom E, Antonovic L, Dasu A, Lax I, Wersäll P, Toma-Dasu I. Treatment fractionation for stereotactic radiotherapy of lung tumours: A modelling study of the influence of chronic and acute hypoxia on tumour control probability. Radiat Oncol 2014;9:149.
- Mayles P, Nahum A, Rosenwald JC et al. Handbook of radiotherapy physics: Theory and practice. Boca Raton, US: Taylor & Francis Group; 2007.
- Barendsen GW. Dose fractionation, dose rate and iso-effect relationships for normal tissue responses. Int J Radiat Oncol Biol Phys 1982;8:1981–97.
- Dasu A, Toma-Dasu I, Karlsson M. Theoretical simulation of tumour oxygenation and results from acute and chronic hypoxia. Phys Med Biol 2003;48:2829–42.
- Konerding MA, Malkusch W, Klapthor B, van Ackern C, Fait E, Hill SA, et al. Evidence for characteristic vascular patterns in solid tumours: Quantitative studies using corrosion casts. Br J Cancer 1999;80:724–32.
- Dasu A, Toma-Dasu I, Karlsson M. The effects of hypoxia on the theoretical modelling of tumour control probability. Acta Oncol 2005;44:563–71.
- Toma-Dasu I, Dasu A, Brahme A. Dose prescription and optimisation based on tumour hypoxia. Acta Oncol 2009; 48:1181–92.
- Dasu A, Toma-Dasu I. Vascular oxygen content and the tissue oxygenation – a theoretical analysis. Med Phys 2008; 35:539–45.
- Toma-Dasu I, Dasu A. Modelling tumour oxygenation, reoxygenation and implications on treatment outcome. Comput Math Methods Med 2013;2013:141087.
- Alper T. Cellular radiobiology. Cambridge, UK: Cambridge University Press; 1979.
- Thames HD. An ‘incomplete-repair’ model for survival after fractionated and continuous irradiations. Int J Radiat Biol Relat Stud Phys Chem Med 1985;47:319–39.
- Fowler JF, Welsh JS, Howard SP. Loss of biological effect in prolonged fraction delivery. Int J Radiat Oncol Biol Phys 2004;59:242–9.
- Partridge M, Ramos M, Sardaro A, Brada M. Dose escalation for non-small cell lung cancer: Analysis and modelling of published literature. Radiother Oncol 2011;99:6–11.
- Chi A, Tom WA, Fowler J, Komaki R, Nguyen NP, Mehta MP, et al. Stereotactic body radiation therapy in non-small-cell lung cancer: linking radiobiological modeling and clinical outcome.. Am J Clin Oncol 2011;34:432–41.
- Dasu A, Toma-Dasu I. Prostate alpha/beta revisited – an analysis of clinical results from 14 168 patients. Acta Oncol 2012;51:963–74.
- Yang J, Fowler JF, Lamond JP, Lanciano R, Feng J, Brady LW. Red shell: Defining a high-risk zone of normal tissue damage in stereotactic body radiation therapy. Int J Radiat Oncol Biol Phys 2010;77:903–9.
- Onishi H, Araki T, Shirato H, Nagata Y, Hiraoka M, Gomi K, et al. Stereotactic hypofractionated high-dose irradiation for stage I nonsmall cell lung carcinoma. Cancer 2004;101:1623–31.
- Blomgren H, Lax I, Näslund I, Svanström R. Stereotactic high dose fraction radiation therapy of extracranial tumours using an accelerator: Clinical experience of the first thirty-one patients. Acta Oncol 1995;34:861–70.
- Lax I, Blomgren H, Näslund I, Svanström R. Stereotactic radiotherapy of malignancies in the abdomen. Acta Oncol 1994;33:677–83.
- Baumann P, Nyman J, Hoyer M, Wennberg B, Gagliardi G, Lax I, et al. Outcome in a prospective phase II trial of medically inoperable stage I non-small-cell lung cancer patients treated with stereotactic body radiotherapy. J Clin Oncol 2009;27:3290–6.
- Park C, Papiez L, Zhang S, Story M, Timmerman RD. Universal survival curve and single fraction equivalent dose: Useful tools in understanding potency of ablative radiotherapy. Int J Radiat Oncol Biol Phys 2008;70:847–52.
- Song CW, Park H, Griffin RJ, Levitt SH. Radiobiology of stereotactic radiosurgery and stereotactic body radiation therapy. In: Levitt SH, Purdy JA, Perez CA, Poortmans P. Technical basis of radiation therapy – practical clinical applications, 5th ed. Berlin, Heidelberg: Springer-Verlag; 2012. p 51–61.