ABSTRACT
Background. Adaptive radiotherapy (ART) could be a tool to reduce toxicity and to facilitate dose escalation in stage III NSCLC. Our aim was to identify the most appropriate time and potential benefit of ART.
Material and methods. We analyzed volume reduction and dosimetric consequences of 41 patients who were treated with concurrent (cCRT) (n = 21) or sequential (sCRT) chemoradiotherapy to a median dose of 70 Gy, 2 Gy/F. At every treatment fraction a cone-beam CT (CBCT) was performed. The gross tumor volume (GTV-T) was adapted (exclusion of lymph nodes) to create the GTV-T-F1. Every fifth fraction (F5–F30), the GTV-T-F1 was adapted on the CBCT to create a GTV-T-Fx. Dose volume histograms were recalculated for every GTV-T-Fx, enabling to create lookup tables to predict the theoretical dosimetric advantage on common lung dose constraints.
Results. The average GTV reduction was 42.1% (range 4.0–69.3%); 50.1% and 33.7% for the cCRT and sCRT patients, respectively. A linear relationship between GTV-T-F1 volume and absolute volume decrease was found for both groups. The mean V5, V20, V30 and mean lung dose increased by 0.8, 3.1, 5.2 and 3.4%, respectively. A larger increase (p < 0.05) was observed for peripheral tumors and cCRT. Lookup tables were generated.
Conclusion. ART offers the most beneficial dosimetric effects when performed around fraction 15, especially for patients with a large initial GTV-T treated by cCRT.
Lung cancer is the most common cause of cancer death worldwide [Citation1]. Non-small cell lung cancer (NSCLC) accounts for 80–85% of all lung cancers of which about 30% are locally advanced (LA) at diagnosis [Citation2]. Although concurrent chemoradiotherapy (cCRT) improves survival compared to sequential one (sCRT), there remains room for improvement in the treatment of LA-NSCLC [Citation3]. Different strategies have been studied, including altered fractionation [Citation4] and dose escalation [Citation5]. However, dose escalation is severely hampered by normal tissue toxicity [Citation5,Citation6] and can lead to deleterious results when used without taking patient-, tumor- and treatment characteristics into account [Citation7,Citation8]. This hurdle can be overcome by patient-individualized treatment approaches such as individualized dose-escalation using fixed dose constraints [Citation9] or adapting the treatment-fields to the shrinking tumor [Citation10]. However, adaptive radiotherapy (ART) is time consuming and it is not clear which patient is eligible or what the optimal time point for ART should be. Technical advances, such as intensity-modulated radiotherapy (IMRT) and tumor motion strategies, may further improve the therapeutic ratio. To reach full potential, these strategies imply the use of image-guided radiotherapy (IGRT), e.g. by using a cone-beam computed tomography (CBCT) [Citation11,Citation12]. The latter also allows monitoring tumor volume or -position changes over the treatment course [Citation13–16]. In this retrospective study, we quantified tumor volume changes during cCRT and sCRT and analyzed possible predictive factors of volume and dosimetric parameter changes, as well as the potential gain on organs at risk (OAR). Furthermore, we aimed to create a predictive tool that should enable to identify who may benefit from ART, and if so, when.
Material and Methods
Patient, tumor and treatment characteristics
Forty-one consecutive patients (33 male; mean age 64, range: 49–81) were irradiated between March 2010 and December 2012, either with cCRT or sCRT. Chemotherapy consisted of three cycles of platinum-based combination therapy. All patients were treated with IMRT using nine non-coplanar intensity-modulated beams conform an in-house developed class solution [Citation17]. A median dose of 70 Gy (range, 62–70 Gy) delivered in 2 Gy daily fractions was prescribed to the PTV. RT started at Day 1 in the concurrent scheme or within 28 days after completing induction chemotherapy. Baseline tumor characteristics are presented in . The location of the primary tumor allows us to differentiate between centrally and peripherally located lesions.
Table I. Baseline tumor characteristics.
Target delineation and imaging
For every patient a slow planning CT and a 2-deoxy-2-[Citation18] fluoro-D-glucose positron emission tomography (PET) images in treatment position were acquired without any breathing compensation. The initial gross tumor volume (GTV-T) and the involved lymph nodes (GTV-lnn) were respectively delineated in lung window setting and mediastinal window setting on the treatment planning CT using mutual information of both biologic (3D PET/CT) and anatomic imaging (planning CT) according to international guidelines [Citation18]. No elective irradiation of the lymphatic regions was conducted. Both the GTV-T and GTV-lnn were expanded by 5 mm to create a clinical target volume (CTV-T and CTV-lnn). It was then adapted to exclude bony and vascular normal structures. A sum of both CTVs was performed to create a final CTV. A planning target volume (PTV) was created by an isotropic expansion of the CTV by 5 mm. At every treatment fraction, a kV-CBCT scan was performed with the on-board imager (Varian Clinac Systems, Palo Alto, CA, USA). CT-CBCT co-registrations were performed by a group of trained RTTs, supervised and approved by the radiation oncologist. Six-degree co-registration on bony anatomy match was initiated using the region of interest including the ribs, the involved lung and the part of the mediastinum. If required, manual correction was applied in order to achieve accurate match at the level of the tumor and surrounding lung. The acquired CBCT images and their registration to the planning CT were automatically imported into the Eclipse treatment planning system (TPS) (Varian Medical Systems, Palo Alto, CA, USA). Based on the first CBCT, the initial GTV was adapted to create the GTV-T-F1. Adaptation implied excluding the lymph nodes (CBCT imaging quality does not allow its accurate evaluation) and adjusting the GTV to the tumor volume of the first fraction. This GTV-T-F1 was used as baseline. For every fifth fraction during the remainder of the treatment, the GTV-T-F1 was copied to the corresponding CBCT scan. The GTV-T-Fx was shaped by adapting the GTV-T-F1 to the corresponding tumor volume on the CBCT in lung window setting. GTV-T-Fx adaptation was performed only in regions where previously present macroscopic tumor tissue visually disappeared. To avoid inter-observer variations and to ascertain unbiased comparison of the volume evolution, all delineations were performed by one radiation oncologist (P.B.). Contouring of the OAR (lungs, spinal cord, heart, esophagus) was performed on the treatment planning CT by a dedicated dosimetrist (B.G. or C.D.) and reviewed by a radiation oncologist. The structure “lungminusGTV-T-Fx” was defined by subtracting the GTV-T-Fx from the lungs on the treatment planning CT without altering the initial densities and/or recalculating the dose distribution in order to maintain consistent evaluation throughout the treatment.
Analysis of tumor volume and dosimetric parameters
The dose volume parameters of the GTV-T-Fx and lungminusGTV-T-Fx were exported from the Eclipse TPS to Excel v14.0 (Microsoft, Redmond, WA, USA) and SPSS v21 (IBM Corp, Somers, NY, USA). From these files, the volumes of the GTV-T-Fx and the dosimetric parameters V5, V20, V30 and mean lung dose (MLD) of the lungminusGTV-T-Fx were extracted. This allowed analysis of volume and dosimetric parameter changes during CRT and investigation of the impact of the location, histology and CRT sequencing. Due to the absence of pathology specification, one patient was excluded for analysis of the impact of histology on volume and dosimetric parameters ( and ). Due to a strong displacement of the tumor position during treatment and hereby compromising the accurate co-registration another patient was excluded from the study for all dosimetric analyses (). The two-sided t-test for independent samples was used to determine statistical significant differences between two datasets. The Levene's test for equality of variances was used. Linear regression analysis was performed in order to investigate relationship between the initial and the final tumor volumes (GTV-T-F1 vs. GTV-T-F30). Furthermore linear relationship between the GTV-T-F1 and the four corresponding dosimetric parameters (V5, V20, V30, MLD) at each given fraction (5, 10, 15, 20, 25, 30) were analyzed leading to a total of 24 correlations (e.g. one relation is a GTV-T-F1 size vs. lungminusGTV-T-V20 at F25). Values of p < 0.05 were considered as statistically significant.
Table II. Mean percent changes in initial (F1) and final (F30) parameters with regards to location, histology and chemotherapy sequence.
Construction of lookup tables
As the initial GTV-T-F1 size might influence the change of the various dosimetric parameters, the whole population was divided into cohorts of small (0–50 cm3 for sCRT and 0–100 cm3 for the cCRT) and large (50–300 cm3 and 100–300 cm3) GTVs. For each sub-group the median values were used to calculate the change (e.g. 50 for the 0–100 cm3 group). Using the results of the regression analysis between the GTV-T-F1 and the lungminusGTV-T dosimetric parameters, lookup tables were generated. The expected change could be expressed as the multiplication of the GTV-T-F1 (in cm3) with the coefficient and adding the intercept. From these equations, we generated two sets of lookup tables: the first part allows a prediction of the percent change of the dosimetric parameters at a certain fraction given the GTV-T-F1 volume. The second part of the lookup table allows an estimation of the possible gain in function of the remaining fractions.
Calculating the effect of remaining fractions
The possible positive changes of tumor shrinkage might suggest later adaptation, but the maximum gain is fading away with each treated fraction (2.8% ≈ 1/35 for a 35-fraction treatment) in a cumulative manner urging the need for early modification. This conflicting situation leads to a proper identification of the optimal time for possible adaptation, by searching for the highest values of the combined effect.
Results
Only for the chemotherapy sequence, a statistically significant difference was found (p = 0.003) regarding the volume change over the treatment course (). For cCRT the GTV-T volume decrease slowed down as the number of fractions increased: the mean volume decrease was 31.1% between fraction 1 and 15, and 19.0% between fraction 15 and 30. For sCRT, conversely, the volume decrease of the GTV-T was slower during the initial 15 fractions (). Regression analysis confirmed a linear relationship between the GTV-T-F1 and GTV-T-F30 for both groups (p < 0.01), predicting an average shrinkage of 29.4% and 40.5% for the sCRT and the cCRT groups, respectively ().
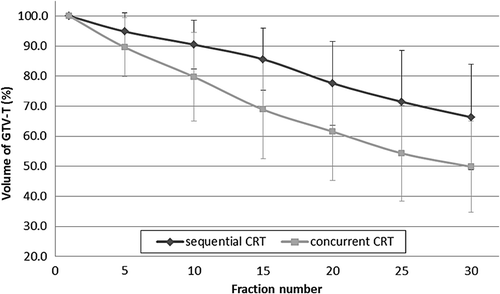
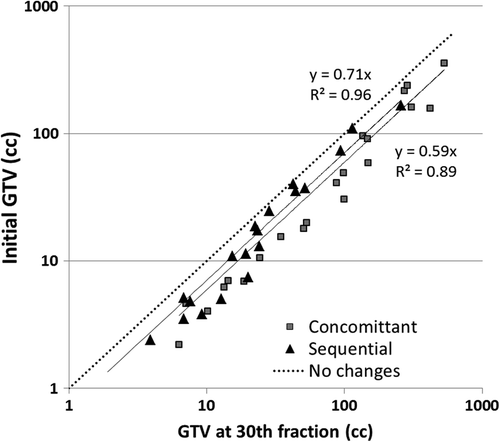
For all investigated parameters, the increase in irradiated normal lung parameters was statistically significantly larger for peripheral locations and cCRT (p < 0.05). No statistically significant differences were observed based on tumor histology (p > 0.05). By rigidly fusing the weekly-adapted volumes to the initial treatment plan, weekly virtual lung DVHs were recalculated showing, an increased mean V20,V30 and MLD of 0.9%, 1.6% and 1.1%, respectively, for the sCRT group and up to 5.3% (V20), 8.8% (V30) and 5.8% (MLD) in the cCRT group for the whole treatment ().
Based on previous findings, distinction was made between cCRT and sCRT. Linear regression analysis of the GTV-T-F1 and lungminusGTV-T dosimetric parameters are summarized in Supplementary Table I (available online at http://informahealthcare.com/doi/abs/10.3109/0284186X.2015.1061209) collecting the coefficients and intercepts for the construction of the lookup tables. With time (/fraction number) each parameter showed an increasing change for all the sub-groups (Supplementary Table II, available online at http://informahealthcare.com/doi/abs/10.3109/0284186X.2015.1061209). For a 35-fraction treatment this reduction effects at 5, 10, 15, 20, 25 and 30 fractions are corresponding to a 86%, 71%, 57%, 43%, 29% and 14%, respectively. Taking the remaining fractions into account we determined the maximum gain for each parameter. For cCRT this momentum is estimated at 15 fractions, for sCRT at 20 fractions (, Supplementary Figure 1, available online at http://informahealthcare.com/doi/abs/10.3109/0284186X.2015.1061209). The absolute percent change of the dosimetric parameters was higher for cCRT than for sCRT, in line with the higher GTV-T volume decrease during cCRT. Also, the percent change of the dosimetric parameters was higher if the initial GTV-T volume is bigger.
Table III. The maximum possible gain of the dosimetric parameters.
Discussion
LA-NSCLC remains a therapeutic challenge. Dose-escalation is one of the radiotherapeutic strategies to increase local control and survival. However, we are limited by normal tissue constraints, which may explain the failure of dose escalation in previous studies [Citation7] and impede further dose escalation strategies. Adaptive RT is one of the possible solutions to overcome this hurdle by avoiding an increase in the dose to the OAR due to tumor volume decrease during treatment. However, this patient individualized treatment is time- and resource consuming and not all patients benefit at the same moment, if at all. Lookup tables, enabling us to predict when to adapt the treatment and for which patients, could allow us to avoid unnecessary efforts. Obviously, this is only useful if our results of tumor shrinkage are in accordance with previously published results.
Our overall volumetric results, measured on weekly cone-beam CT images, showed an average GTV reduction of 42.1% or 1%/day over 30 fractions and a mean period of 42 days. We observed a significant difference between patients treated with cCRT and sCRT with an average GTV reduction over 30 fractions of 50.1% (1.2%/day) and 33.7% (0.8%/day) respectively. A tumor volume reduction of 1.2% per day is in excellent accordance with previously published results of tumor shrinkage data during cCRT or RT only [Citation10,Citation13]. Our overall volume regression data is also in good agreement with previously published reports using various imaging modalities [Citation10,Citation13,Citation19–22], as is the significant impact on tumor regression in case of cCRT compared to a sequential scheme [Citation23]. The latter may be explained by previous tumor shrinkage during chemotherapy and/or accelerated tumor repopulation [Citation24]. The effect of tumor repopulation is well known in limited disease small cell lung cancer and may also explain why tumor regression slows down in the second half of the cCRT regimen. Indeed, in case of cCRT, we observed a deceleration of weekly volume reduction after 15 fractions. This observation is in line with the paper by Woodford suggesting that a GTV decrease of more than 30% in the first 20 fractions would be a useful trigger point for adaptive planning [Citation14]. We preferred to individualize this trigger point per patient by generating lookup tables, which shows a theoretical decrease in lung DVH in case effective ART would be performed. Again, this value was significantly higher in patients treated with cCRT. Also the location of the tumor plays a significant role, in favor of the peripherally located tumors. Taking into account that a peripherally located tumor is totally surrounded by lung tissue, this observation is not surprising.
Using both volume and dosimetric data, we constructed lookup tables in order to predict, according to the timing of the RT and volume of the tumor, if and when ART could be useful, in order to decrease the lung dose or to allow for a patient individualized isotoxic dose-escalation. When looking at the optimal moment to perform a single adaptation during RT we also found a fixed trigger point at 15 fractions and 20 fractions for cCRT and sCRT, respectively. The potential underdosage of microscopic disease after ART is a genuine concern, particularly in case of IMRT planning where steep dose gradients are warranted. Guckenberger et al. addressed this issue and concluded that ART does not compromise dose coverage and tumor control probability of volumes of potential microscopic disease [Citation10].
Limitations of the current study include the small number of patients in each treatment arm and the omission of breathing motion into our calculations. Although Shmidt et al. have shown that the impact of anatomical tumor changes in general resulted in larger dosimetric changes than both respiratory motion and baseline shifts [Citation25], increasing tumor motion with decreasing tumor volume has been described [Citation8]. Another potential shortcoming of our study is that delineation of malignant lymph nodes was not feasible on CBCT and therefore not addressed for. However, their shrinkage may not be ignored when implementing adaptive treatment [Citation26]. Furthermore, our dosimetric parameter calculations are based on rigid fusion and tumor volumes of the consecutive CBCTs during treatment. The latter two shortcomings however imply that the dosimetric benefit might even be higher if effective plan adaptation would be performed.
In conclusion, ART as determined by repeated CBCT imaging might be a feasible strategy to prospectively validate the lookup tables aiming at selecting the optimal candidate and timeframe to benefit from ART to its full potential.
Supplementary material available online
Supplementary Figure 1 and Tables I, II available online at http://informahealthcare.com/doi/abs/10.3109/0284186X.2015.1061209
ionc_a_1061209_sm1936.zip
Download Zip (266.8 KB)Acknowledgements
Dr. Katrien Vandecasteele holds a mandate for fundamental and clinical research of the Foundation against Cancer – Belgium.
Declaration of interest: The authors report no conflicts of interest. The authors alone are responsible for the content and writing of the paper.
References
- Ferlay J, Shin HR, Bray F, Forman D, Mathers C, Parkin DM. Estimates of worldwide burden of cancer in2008: GLOBOCAN 2008.Int J Cancer 2010;127: 2893–917.
- Peters S, Adjei AA, Gridelli C, Reck M, Kerr K, Felip E, et al. Metastatic non-small-cell lung cancer (NSCLC): ESMO Clinical Practice Guidelines for diagnosis, treatment and follow-up. Ann Oncol 2012;23(Suppl 7):vii56–64.
- Auperin A, Le Pechoux C, Rolland E, Curran WJ, Furuse K, Fournel P, et al. Meta-analysis of concomitant versus sequential radiochemotherapy in locally advanced non-small-cell lung cancer. J Clin Oncol 2010;28:2181–90.
- Haslett K, Pottgen C, Stuschke M, Faivre-Finn C. Hyperfractionated and accelerated radiotherapy in non-small cell lung cancer. J Thorac Dis 2014;6:328–35.
- Bradley J. A review of radiation dose escalation trials for non-small cell lung cancer within the Radiation Therapy Oncology Group. Semin Oncol 2005;32:S111–3.
- Belderbos JS, Heemsbergen WD, De Jaeger K, Baas P, Lebesque JV. Final results of a phase I/II dose escalation trial in non-small-cell lung cancer using three-dimensional conformal radiotherapy. Int J Radiat Oncol Biol Phys 2006; 66:126–34.
- Cox JD. Are the results of RTOG 0617 mysterious? Int J Radiat Oncol Biol Phys 2012;82:1042–4.
- Chi A, Nguyen NP, Welsh JS, Tse W, Monga M, Oduntan O, et al. Strategies of dose escalation in the treatment of locally advanced non-small cell lung cancer: Image guidance and beyond. Front Oncol 2014;4:156.
- van Baardwijk A, Reymen B, Wanders S, Borger J, Ollers M, Dingemans AM, et al. Mature results of a phase II trial on individualised accelerated radiotherapy based on normal tissue constraints in concurrent chemo-radiation for stage III non-small cell lung cancer. Eur J Cancer 2012;48:2339–46.
- Guckenberger M, Richter A, Wilbert J, Flentje M, Partridge M. Adaptive radiotherapy for locally advanced non-small-cell lung cancer does not underdose the microscopic disease and has the potential to increase tumor control. Int J Radiat Oncol Biol Phys 2011;81:e275–82.
- Bissonnette JP, Purdie TG, Higgins JA, Li W, Bezjak A. Cone-beam computed tomographic image guidance for lung cancer radiation therapy. Int J Radiat Oncol Biol Phys 2009;73:927–34.
- Guckenberger M, Meyer J, Wilbert J, Richter A, Baier K, Mueller G, et al. Intra-fractional uncertainties in cone-beam CT based image-guided radiotherapy (IGRT) of pulmonary tumors. Radiother Oncol 2007;83:57–64.
- Kupelian PA, Ramsey C, Meeks SL, Willoughby TR, Forbes A, Wagner TH, et al. Serial megavoltage CT imaging during external beam radiotherapy for non-small-cell lung cancer: Observations on tumor regression during treatment. Int J Radiat Oncol Biol Phys 2005;63:1024–8.
- Woodford C, Yartsev S, Dar AR, Bauman G, Van Dyk J. Adaptive radiotherapy planning on decreasing gross tumor volumes as seen on megavoltage computed tomography images. Int J Radiat Oncol Biol Phys 2007;69:1316–22.
- Knap MM, Hoffmann L, Nordsmark M, Vestergaard A. Daily cone-beam computed tomography used to determine tumour shrinkage and localisation in lung cancer patients. Acta Oncol 2010;49:1077–84.
- Persoon LC, Egelmeer AG, Ollers MC, Nijsten SM, Troost EG, Verhaegen F. First clinical results of adaptive radiotherapy based on 3D portal dosimetry for lung cancer patients with atelectasis treated with volumetric-modulated arc therapy (VMAT). Acta Oncol 2013;52:1484–9.
- Derycke S, De Gersem WR, Van Duyse BB, De Neve WC. Conformal radiotherapy of Stage III non-small cell lung cancer: A class solution involving non-coplanar intensity-modulated beams. Int J Radiat Oncol Biol Phys 1998;41: 771–7.
- De Ruysscher D, Faivre-Finn C, Nestle U, Hurkmans CW, Le Pechoux C, Price A, et al. European Organisation for Research and Treatment of Cancer recommendations for planning and delivery of high-dose, high-precision radiotherapy for lung cancer. J Clin Oncol 2010;28:5301–10.
- Britton KR, Starkschall G, Tucker SL, Pan T, Nelson C, Chang JY, et al. Assessment of gross tumor volume regression and motion changes during radiotherapy for non-small-cell lung cancer as measured by four-dimensional computed tomography. Int J Radiat Oncol Biol Phys 2007;68: 1036–46.
- Fox J, Ford E, Redmond K, Zhou J, Wong J, Song DY. Quantification of tumor volume changes during radiotherapy for non-small-cell lung cancer. Int J Radiat Oncol Biol Phys 2009;74:341–8.
- Ramsey CR, Langen KM, Kupelian PA, Scaperoth DD, Meeks SL, Mahan SL, et al. A technique for adaptive image-guided helical tomotherapy for lung cancer. Int J Radiat Oncol Biol Phys 2006;64:1237–44.
- Sonke JJ, Belderbos J. Adaptive radiotherapy for lung cancer. Semin Radiat Oncol 2010;20:94–106.
- Seibert RM, Ramsey CR, Hines JW, Kupelian PA, Langen KM, Meeks SL, et al. A model for predicting lung cancer response to therapy. Int J Radiat Oncol Biol Phys 2007;67:601–9.
- Chen CP, Weinberg VK, Jahan TM, Jablons DM, Yom SS. Implications of delayed initiation of radiotherapy: Accelerated repopulation after induction chemotherapy for stage III non-small cell lung cancer. J Thorac Oncol 2011; 6:1857–64.
- Schmidt ML, Hoffmann L, Kandi M, Moller DS, Poulsen PR. Dosimetric impact of respiratory motion, interfraction baseline shifts, and anatomical changes in radiotherapy of non-small cell lung cancer. Acta Oncol 2013;52:1490–6.
- Pantarotto JR, Piet AH, Vincent A, van Sornsen de Koste JR, Senan S. Motion analysis of 100 mediastinal lymph nodes: Potential pitfalls in treatment planning and adaptive strategies. Int J Radiat Oncol Biol Phys 2009;74:1092–9.