ABSTRACT
Background. The bladder is a tumour site well suited for adaptive radiotherapy (ART) due to large inter-fractional changes, but it also displays considerable intra-fractional motion. The aim of this study was to assess target coverage with a clinically applied method for plan selection ART and to estimate population-based and patient-specific intra-fractional margins, also relevant for a future re-optimisation strategy.
Material and methods. Nine patients treated in a clinical phase II ART trial of daily plan selection for bladder cancer were included. In the library plans, 5 mm isotropic margins were added to account for intra-fractional changes. Pre-treatment and weekly repeat magnetic resonance imaging (MRI) series were acquired in which a full three-dimensional (3D) volume was scanned every second min for 10 min (a total of 366 scans in 61 series). Initially, the bladder clinical target volume (CTV) was delineated in all scans. The t = 0 min scan was then rigidly registered to the planning computed tomography (CT) and plan selections were simulated using the CTV_0 (at t = 0 min). To assess intra-fractional motion, coverage of the CTV_10 (at t = 10 min) was quantified using the applied PTV. Population-based margins were calculated using the van Herk margin recipe while patient-specific margins were calculated using a linear model.
Results. For 49% of the cases, the CTV_10 extended more than 5 mm outside the CTV_0. However, in 58 of the 61 cases (97%) CTV_10 was covered by the selected PTV. Population-based margins of 14 mm Sup/Ant, 9 mm Post and 5 mm Inf/Lat were sufficient to cover the bladder. Using patient-specific margins, the overlap between PTV and bowel-cavity was reduced from 137 cm3 with the plan selection strategy to 24 cm3.
Conclusion. In this phase II ART trial, 5 mm isotropic margin for intra-fractional motion was sufficient even though considerable intra-fractional motion was observed. In online re-optimised ART, population-based margin can be applied although patient-specific margins are preferable.
Many tumour sites treated with radiotherapy (RT) display considerable internal motion during a course of treatment. Adaptive RT (ART) is currently investigated in several institutions to account for these changes [Citation1–4]. The urinary bladder is a highly mobile organ and was one of the first sites where ART has been explored clinically [Citation5–7]. In ART, patient- specific information from, e.g. cone beam computed tomography (CBCT) scans is applied to individualise the treatment allowing inter-fractional changes in the bladder target to be accounted for. However, there may also be considerable changes of volume, position and shape of the bladder during delivery of each fraction. Varying margins have been used to cover for intra-fractional motion in online plan selection RT of bladder cancer, ranging from 3 to 7 mm isotropic, but also an-isotropic margins have been suggested [Citation4,Citation5,Citation8]. The margin needed to account for intra-fractional changes will depend on the filling status of the bladder and the drinking instructions (if applied) as well as the time interval from CBCT acquisition to the last monitor unit (MU) is delivered [Citation9,Citation10]. The introduction of treatment techniques, such as volumetric arc therapy (VMAT) has decreased the treatment (i.e. fraction) delivery time considerably and thereby potentially decreased the intra-fractional margin needed to cover the bladder. Studies have investigated intra-fractional changes using CBCTs acquired after treatment delivery [Citation11]. A few studies have used cine-magnetic resonance imaging (MRI) scans to identify the intra-fractional bladder shape changes, however, over time intervals up to 20–30 min [Citation12,Citation13]. MRI has the advantage of being non-ionising and ‘non-invasive’ and moreover can be acquired repeatedly within a short acquisition time.
In our clinical phase II trial of plan selection for bladder cancer, patients were treated with daily selection from a library of three VMAT plans, each generated with a 5 mm isotropic intra-fractional margin [Citation14]. The aim of this study was therefore to investigate bladder motion patterns during the time frame of a typical VMAT treatment fraction and to assess whether target coverage was retained for the patients treated in the protocol under the assumption that the motion seen during the MRI scans was representative for the motion occurring during a fraction delivery. Furthermore, we wanted to estimate the intra- fractional margins needed to assure target coverage in the setting of online adaptive re-optimisation.
Material and methods
The first 10 patients included in a clinical trial of adaptive plan selection for bladder cancer were considered for this study. One patient was excluded because of a massively enlarged seminal vesicle. One female and eight male patients with muscle-invasive urinary bladder cancer (one with T4a disease) underwent MRI scanning both pre-treatment as well as every week throughout their course of RT. All treatment sessions were delivered, and all scans were acquired on voided bladders. Each MRI series consisted of an mDixon sequence (voxel size: 0.9 × 0.9 × 1.5 mm; scan time: 40 s; Philips Ingenia 1.5T) acquired at t = 0, 2, 4, 6, 8, and 10 min. A total of 366 scans were acquired in 61 series (6–7 MRI scans per patient). Post-treatment CBCTs were acquired on the same day as the weekly repeat MRI series. The bladder clinical target volume (CTV) was delineated on all MRI scans as well as the weekly CBCTs.
Adaptive planning and evaluation of target coverage
In the ART plan selection protocol, the patients were in the first 5 fractions treated non-adaptively, i.e. using large population-based margins. The bladder was delineated on the first four CBCTs and copied to the planning CT using the online registration on bony anatomy. From the four CBCT delineations and the planning CT, composite CTVs were created for small- and medium-sized plan selection volumes (PSVSmall and PSVMedium) [Citation15]. PSVsmall was based on the volume contained in at least two of five CTVs as delineated on the planning CT and the CBCTs acquired during the first four fractions whereas PSVmedium was based on the union of the same five CTVs. To account for intra-fractional motion a 5 mm isotropic margin was added to create the corresponding PTVs. PTVlarge is defined as the CTV with population-based inter-fractional margins [Citation16]. The dose prescription was 60 Gy in 30 fractions to the bladder.
For the purpose of this study, the weekly t = 0 min MRI scan of each patient was rigidly registered by bony anatomy to the planning CT and the plan selection procedure was performed using the bladder/CTV at t = 0 min (CTV_0). First, the PSVSmall overlaid on the MRI was evaluated and if the CTV_0 was covered the patient was considered treated with the small-sized treatment plan. Otherwise, the PSVMedium was evaluated and if the CTV_0 was covered by this volume, the medium-sized plan was selected. If neither of these two PSVs covered the CTV_0, the large-sized treatment plan was chosen. In very few occasions, the CTV_0 was not covered by the PTVLarge. Using the selected plans, coverage of the CTV at t = 10 min (CTV_10) by the corresponding PTV was assessed (Supplementary Figure 1, available online at http://www.informahealthcare.com/doi/abs/10.3109/0284186X.2015.1062138). Time from CBCT acquisition to last MU, defined as session delivery time (SDT), was calculated from timestamp data (ARIA, Varian Medical Systems, Palo Alto, CA, USA).
Population-based margin calculations
For all further margin calculations, all bladder CTVs were registered to the first scan (pre-treatment MRI at t = 0 min) and the resulting structure sets were exported to MATLAB for analysis. The centre of the volume for the CTV at t = 0 min was used as the centre for the CTVs at times t = 2, 4, 6, 8, 10 min. The CTVs were interpolated and represented by spherical coordinates.
The method used to calculate population-based margins was based on previous work of Meijer et al. [Citation8]. The random errors were calculated using the following equation:
In Equation 1, P is the number of patients, N the number of scans per patient while r0 and r10 are the radii in the same direction at time t = 0 and t = 10 min. is the average bladder expansion for each patient in each direction and δj the angle between the normal vector on the surface of the bladder and the direction vector from the centre of the bladder to the surface point. In the calculations only bladder expansions were considered, and the few occasions where a bladder contraction occurred the expansion was set to zero.
The formula used to calculate the systematic error is shown in Equation 2, where is the average of
for all patients:
The random and systematic errors were combined as shown in Equation 3, to give margins (m) that ensure a minimal radiation dose of 95% to the target for 90% of the patients [Citation17]:
In steps of 10 degrees (φ: –90˚–90˚, θ: 0˚–360˚), a set of 19 × 37 points on the bladder surface was used to estimate the margin (maps).
Patient-specific margin calculations
To estimate patient-specific margins, intra-fractional changes in eight directions (superior, inferior, anterior, posterior, left, right, sup-ant, inf-pos) were calculated. A linear regression model was applied for a point in each of the eight directions and for each series (an overview of the bladder movements is presented in Supplementary Figure 2, available online at http://www.informahealthcare.com/doi/abs/10.3109/0284186X.2015.1062138). Second, we calculated the radial difference between the bladder contours at t = 0 min and t = 10 min, again in steps of 10˚ (φ: –90˚–90˚, θ: 0˚–360˚), resulting in the 19 × 37 points on the bladder surface. The patients were categorised as having either small or large bladder expansions based on the linear regression model together with the complete spherical model: A patient with the 95% confidence interval for the average linear expansion exceeding 5 mm in one or more of the directions were considered as having large intra-fractional movement.
The first two MRI-series were used to calculate the patient-specific margins. The expansion during the 10 min for a point in the six directions (superior, inferior, anterior, posterior, left, right) was estimated. If the expansion in one of the directions was larger than 5 mm the margin for this patient was estimated in the specific direction by linear regression. The linear model was forced through the origin. The slope represented the bladder expansion per minute, so this value (i.e. the slope) was multiplied by 10 to give the expansion after 10 min. The 95% confidence interval for the slope was used as the estimate for patient-specific margin.
Volume of bowel cavity and rectum in the PTVs
To assess the implications for normal tissue irradiation, we determined the intersection between the PTVs resulting from the two intra-fractional margins to be used in the re-optimisation strategy and the bowel-cavity/rectum, and compared these to the intersection from the PTVs applied in the actual treatment (plan selection ART); these calculations were performed based on the Eclipse treatment planning system (Varian Medical Systems).
Statistics
The volumes on pre-treatment CBCT and the CTV_0 were compared using Bland-Altman plots as well as paired t-tests. The Bland-Altman plots showed that the data was normally distributed.
Results
The initial bladder volume varied from 40 cm3 to 340 cm3. The median volume increase between CTV_0 and CTV_10 was 11 cm3 (90% percentile: 35 cm3). The 90% percentile of SDT for all fractions delivered was 10 min (median: 8 min). No significant difference between the pre-treatment CBCT and the MRI bladder volumes were found.
In 49% of the 61 MRI sessions the CTV_10 extended more than 5 mm outside the CTV_0. Nevertheless, in 59 of 61 (97%) of the cases, the CTV_10 was covered by the corresponding PTV in the plan selection procedure. In two of the cases where CTV_10 was outside the PTVLarge also the CTV_0 extended outside the PTVlarge, and according to our protocol, the patients would have been instructed to empty the bladder before a new scan would be acquired. A motion episode in one patient and two episodes in a second patient resulted in transient incomplete bladder coverage.
In the population-based margin calculation with only the male patients included we found that margins in the superior, anterior and posterior directions were largest; 14 mm, 14 mm and 9 mm, respectively (). The margins were calculated both with and without the data from the female patient as her data showed unexpected movements (changing the margins with 4 mm). Approximating the margin for the inferior, left and right directions to 5 mm, three of the eight male patients did not have their entire bladder covered in one of the series. For these three cases the volume coverage ranged between 98.1% and 99.9%.
Figure 1. 2D-maps of the population-based margins, showing both the margins for all nine patients (upper) and the margins when the one female patient was excluded due to anatomically unexpected motion (lower). Superior direction is found at θ = 90˚ and inferior at θ = –90˚. Anterior, posterior as well as left and right directions are seen for θ = 0˚, with posterior at φ = 90˚, anterior at φ = 270˚, left at φ = 0˚/360˚, and right at φ = 180˚. The top and the bottom line of all the 2D-images describe the same point. The colour difference describes the intra-fractional changes in mm.
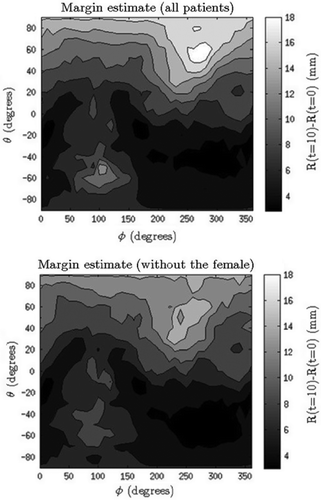
Six of the patients were categorised as having large bladder movements. In 10% of the scan series bladder shifts larger than 5 mm were observed, but only in one occasion was the movement not observable in the 10 min scan.
Six patients had patient-specific intra-fractional margins estimated in the directions where the bladder expansions exceeded 5 mm; shows the result of the linear regression, with the upper 95% confidence interval (CI) limit of the slopes reaching a maximum of around 1.2 mm/min. also shows the average coverage for each patient from the second week onwards. The bladder volumes were covered with an average of over 99%.
Table I. The result of a linear regression model for patient-specific margins. Margin is determined as the upper 95% CI times 10 min, since margin should account for a 10 min interval. The bottom table shows the average bladder volume covered by PTV for each patient. The bladders are almost completely covered, with an average of over 99%.
The intersections between the resulting PTVs and the bowel cavity for the two re-optimisation methods as well as the intersections between the clinically applied plan selection are shown in . The average volume (range) for the three approaches at t = 10 min were 136.9 cm3 (range 58.5–199.0 cm3) for plan selection, 69.0 cm3 (range 20.9–136.4 cm3) for population-based margins and 24.0 cm3 (range 6.4–50.7 cm3) for patient-specific margins. The corresponding comparison for the rectum with the three margin alternatives showed the same trend, with 5.3 cm3 (range 0.0–15.0 cm3) for plan selection, 0.6 cm3 (range 0.0–3.5 cm3) for population-based and 0.0 cm3 (range 0.0–0.3 cm3) for patient-specific margins.
Discussion
The main finding of this study was that our clinically used plan selection strategy resulted in good target coverage, with CTV_10 covered by the corresponding PTV in all but two of the cases. In those two cases the CTV_0 was not covered either and in the clinical plan selection procedure the patient would have been asked to empty the bladder before a new CBCT acquisition could be performed. The 5 mm margin for intra-fractional motion used clinically was therefore sufficient despite that considerable intra-fractional motion was observed. This is in contrast to a study by Foroudi et al. that showed that a margin of 7 mm was insufficient [Citation18]. In the Foroudi study, the 7 mm margin was only added to the CTV like in the re-optimisation strategy, while in this study it is added to the selected PSV, which always encompasses the bladder with an additional margin. Furthermore another reason could be that the patients in the Foroudi study also received chemotherapy, which increases the urine flow.
The intra-fractional bladder motion was examined, and margins relevant for an online re-optimisation calculated. All patients had intra-fractional changes larger than 5 mm, so a 5 mm margin is unlikely to be sufficient within a re-optimisation strategy. Some patients had bladder displacements where the bladder shape and size did not change. Only in one occasion of 61 was there a displacement that was not observable in the 10 min scan, as the bladder had moved towards the starting position. This suggests that the margin models can be based solely on the 0 and 10 min scans.
An analysis of intra-fractional changes using 2D-maps was presented also by Lotz et al. [Citation19]. Overall our results are comparable to those of Lotz et al, with expansions primarily in the anterior and posterior directions. Their two-dimensional (2D)-maps contained the average root mean square value of the radial difference for all the patients, showing a maximum expansion of 20 mm in the superior-anterior direction. This is larger than the expansions seen in this study; the average expansions in our series was at most 7.2 mm in the superior direction and 6.4 mm in the anterior direction. The difference in the expansions may be due to the time interval in this study being shorter; the models in the Lotz study estimated the bladder changes over a time interval of 30 min.
The amount of bowel-cavity in the PTV was reduced using the re-optimisation method compared to what was achieved with our plan selection protocol. The patient-specific method resulted in a 45 cm3 reduction of the average bowel volume inside the PTV compared to the population-based approach. Using patient-specific margins is more time consuming, as a new margin has to be calculated for each patient. Even though only two MRI series were used, it was possible to select the patients with large bladder expansions and increase the margin for these patients. More patients are needed to confirm these findings before the method could be used clinically. In clinical practice the inaccuracy in the bladder segmentation by deformable registration would have to be taken into account, either by adding additional margin or as in this study, where manual corrections were considered. The accuracy of the deformable image registration is important, because of the time used for the manual corrections of the targets and normal tissues [Citation20].
The method used to determine the radii applied for both margin calculations has some uncertainties, e.g. when the data is exported to MATLAB the bladder contours are translated into binary matrices. Due to the pixel size this leads to uncertainties of around 1 mm in the representation in the bladder surface.
We found that the expansion was largest in the superior and anterior direction and this has also been shown in other studies. Foroudi et al. [Citation11] found that the bladder expansion happened primarily in the superior and anterior direction and that anisotropic margins are necessary. The intra-fractional margins in the Foroudi study ranged between 7 and 13 mm across the six directions (upper confidence intervals ranged between 9 and 15 mm), quite comparable to the population-based margins calculated in this study. An alternative to using population-based margin models is online MRI-guidance [Citation21], this method has also been investigated for cervix cancer patients [Citation22].
It would be interesting to investigate data from more female patients, as it appears that there could be a difference in bladder movement for the female patients. It is not unlikely that a separate margin model should be developed for female patients.
The patients in this study had a weekly post-fraction delivery CBCT scan (on the same day as the MRI scans) and the population-based margin-map was also calculated for these (Supplementary Figure 3, available online at http://www.informahealthcare.com/doi/abs/10.3109/0284186X.2015.1062138). The female patient had normal bladder movements on the CBCT scans and consequently did not have a special effect on the margin. It turned out that the margins found using the CBCT scans were smaller than those found based on the MRI data. The margin map had a maximum of 11 mm without the female patient compared to 16 mm for the margin-map based on MRI scans.
In conclusion, this study has showed that our clinically used plan selection strategy resulted in good target coverage. Population-based intra- fractional margin was found to be large in the superior and anterior directions. Patient-specific margins for intra-fractional motion may be feasible, as we could identify and estimate such margins from limited data from scans taken before or early in the treatment. Use of patient-specific margins resulted in a considerable reduction in the bowel-cavity volume encompassed by the PTV.
Supplementary material available online
Supplementary Figures 1–3 available online at http://www.informahealthcare.com/doi/abs/10.3109/0284186X.2015.1062138.
ionc_a_1062138_sm3919.docx
Download MS Word (5.7 MB)Declaration of interest: The authors report no conflicts of interest. The authors alone are responsible for the content and writing of the paper.
References
- Heukelom J, Hamming O, Bartelink H, Hoebers F, Giralt J, Herlestam T, et al. Adaptive and innovative Radiation Treatment For improving Cancer treatment outcomE (ARTFORCE); a randomized controlled phase II trial for individualized treatment of head and neck cancer. BMC Cancer 2013;13:84.
- Thörnqvist S, Hysing LB, Zolnay AG, Söhn M, Hoogeman MS, Muren LP, et al. Adaptive radiotherapy in locally advanced prostate cancer using a statistical deformable motion model. Acta Oncol 2013;52:1423–9.
- Grau C, Høyer M, Alber M, Overgaard J, Lindegaard JC, Muren LP. Biology-guided adaptive radiotherapy (BiGART) – more than a vision? Acta Oncol 2013;52:1243–7.
- McDonald F, Lalondrelle S, Taylor H, Warren-Oseni K, Khoo V, McNair HA, et al. Clinical implementation of adaptive hypofractionated bladder radiotherapy for improvement in normal tissue irradiation. Clin Oncol 2013;25:549–56.
- Tuomikoski L, Collan J, Keyriläinen J, Visapää H, Saarilahti K, Tenhunen M. Adaptive radiotherapy in muscle invasive urinary bladder cancer – An effective method to reduce the irradiated bowel volume. Radiother Oncol 2011;99:61–6.
- Thariat J, Aluwini S, Pan Q, Caullery M, Marcy PY, Housset M, et al. Image-guided radiation therapy for muscle-invasive bladder cancer. Nat Rev Urol 2011;9:23–9.
- Lotz HT, Pos FJ, Hulshof MC, van Herk M, Lebesque JV, Duppen JC, et al. Tumor motion and deformation during external radiotherapy of bladder cancer. Int J Radiat Oncol Biol Phys 2006;64:1551–8.
- Meijer GJ, Rasch C, Remeijer P, Lebesque JV. Three-dimensional analysis of delineation errors, setup errors, and organ motion during radiotherapy of bladder cancer. Int J Radiat Oncol Biol Phys 2003;55:1277–87.
- Tuomikoski L, Korhonen J, Collan J, Keyriläinen J, Visapää H, Sairanen J, et al. Implementation of adaptive radiation therapy for urinary bladder carcinoma: imaging, planning and image guidance. Acta Oncol 2013;52:1451–7.
- Dees-Ribbers HM, Betgen A, Pos FJ, Witteveen T, Remeijer P, van Herk M. Inter- and intra-fractional bladder motion during radiotherapy for bladder cancer: A comparison of full and empty bladders. Radiother Oncol 2014;113:254–9.
- Foroudi F, Pham D, Bressel M, Gill S, Kron T. Intrafraction bladder motion in radiation therapy estimated from pretreatment and posttreatment volumetric imaging. Int J Radiat Oncol Biol Phys 2012;86:77–82.
- McBain CA, Khoo VS, Buckley DL, Sykes JS, Green MM, Cowan RA, et al. Assessment of bladder motion for clinical radiotherapy practice using cine–magnetic resonance imaging. Int J Radiat Oncol Biol Phys 2009;75:664–71.
- Mangar SA, Scurr E, Huddart RA, Sohaib HA, Horwich A, Dearnaley DP, et al. Assessing intra-fractional bladder motion using cine-MRI as initial methodology for Predictive Organ Localization (POLO) in radiotherapy for bladder cancer. Radiother Oncol 2007;85:207–14.
- Vestergaard A, Muren LP, Søndergaard J, Elstrøm UV, Høyer M, Petersen JB. Adaptive plan selection vs. re-optimisation in radiotherapy for bladder cancer: A dose accumulation comparison. Radiother Oncol 2013;109:457–62.
- Vestergaard A, Muren LP, Lindberg H, Jakobsen KL, Petersen JB, Elstrøm UV, et al. Normal tissue sparring in a phase II trial on daily adaptive plan selection in radiotherapy for urinary bladder cancer. Acta Oncol 2014;53:997–1004.
- Muren LP, Smaaland R, Dahl O. Organ motion, set-up variation and treatment margins in radical radiotherapy of urinary bladder cancer. Radiother Oncol 2003;69:291–304.
- Van Herk M. Errors and margin in radiotherapy. Semin Radiat Oncol 2004;14:52–64.
- Foroudi F, Pham D, Rolfo A, Bressel M, Tang CI, Tan A, et al. The outcome of a multi-centre feasibility study of online adaptive radiotherapy for muscle-invasive bladder cancer TROG 10.01 BOLART. Radiother Oncol 2014;111:316–20.
- Lotz HT, Remeijer P, van Herk M, Lebesque JV, de Bois JA, Zijp LJ, et al. A model to predict bladder shapes from changes in bladder and rectal filing. Med Phys 2004;31:1415–23.
- Thor M, Andersen ES, Petersen JBB, Sørensen TS, Noe KØ, Tanderup K, et al. Evaluation of an application for intensity-based deformable image registration and dose accumulation in radiotherapy. Acta Oncol 2014;53:1329–36.
- Vestergaard A, Hafeez S, Muren LP, Nill S, Høyer M, Hansen VN, et al. The potential of MRI-guided online adaptive re-optimisation in radiotherapy of urinary bladder cancer. Radiother Oncol Forthcoming 2015.
- Oh S, Stewart J, Moseley J, Kelly V, Lim K, Xie J, et al. Hybrid adaptive radiotherapy with on-line MRI in cervix cancer IMRT. Radiother Oncol 2014;110:323–8.