ABSTRACT
Background. Onboard magnetic resonance imaging (OB-MRI) for daily localization and adaptive radiotherapy has been under development by several groups. However, no clinical studies have evaluated whether OB-MRI improves visualization of the target and organs at risk (OARs) compared to standard onboard computed tomography (OB-CT). This study compared visualization of patient anatomy on images acquired on the MRI-60Co ViewRay system to those acquired with OB-CT.
Material and methods. Fourteen patients enrolled on a protocol approved by the Institutional Review Board (IRB) and undergoing image-guided radiotherapy for cancer in the thorax (n = 2), pelvis (n = 6), abdomen (n = 3) or head and neck (n = 3) were imaged with OB-MRI and OB-CT. For each of the 14 patients, the OB-MRI and OB-CT datasets were displayed side-by-side and independently reviewed by three radiation oncologists. Each physician was asked to evaluate which dataset offered better visualization of the target and OARs. A quantitative contouring study was performed on two abdominal patients to assess if OB-MRI could offer improved inter-observer segmentation agreement for adaptive planning.
Results. In total 221 OARs and 10 targets were compared for visualization on OB-MRI and OB-CT by each of the three physicians. The majority of physicians (two or more) evaluated visualization on MRI as better for 71% of structures, worse for 10% of structures, and equivalent for 14% of structures. 5% of structures were not visible on either. Physicians agreed unanimously for 74% and in majority for > 99% of structures. Targets were better visualized on MRI in 4/10 cases, and never on OB-CT.
Conclusion. Low-field MR provides better anatomic visualization of many radiotherapy targets and most OARs as compared to OB-CT. Further studies with OB-MRI should be pursued.
Magnetic resonance imaging (MRI)-based radiotherapy (RT) has recently become a prominent interest in radiation oncology. It is well accepted that MRI can provide advantages over x-ray-based imaging for pretreatment planning of targets in the head and neck, central nervous system, and pelvis [Citation1,Citation2]. The additional benefit of image acquisition without the use of ionizing radiation makes MRI a particularly attractive modality for weekly, daily, or even continuous imaging throughout treatment. These advantages have motivated the development of onboard MRI for daily patient setup, anatomical localization and adaptive treatment.
Several groups have made substantial progress in the development of onboard MRI technology, despite considerable technical challenges. The first MRI-RT device is now in clinical use [Citation3] and several other variants of onboard MRI-RT devices exist in various stages of development. Research teams in the Netherlands [Citation4] and Canada [Citation5] are each developing systems integrating linear accelerators with onboard MRI. Groups from Melbourne [Citation6] and Cleveland [Citation7] have proposed an alternative design, using a 60Co treatment approach to simplify the technical considerations. A less integrated solution, where the MRI is de-coupled from the treatment system, has also been developed [Citation8]. Many of these MRI-RT devices are designed to utilize low-field MRI (0.2–0.35T) to reduce interference with radiation therapy delivery and decrease geometric distortion [Citation8].
The clinical advantage of onboard low-field MRI for daily anatomical position verification versus available onboard imaging with integrated kV imagers has not been established. There are many studies comparing MRI simulation images to computed tomography (CT) simulation images which have shown that MRI can more accurately represent soft tissue anatomy [Citation1,Citation2], however this is not necessarily translatable to onboard imaging technology. Low-field MRI is characterized by a relatively low signal-to-noise ratio, and the implications of this technology for clinical target and normal structure visualization are unknown. A comparison of low-field onboard MRI to the onboard CT-based standard for image-guided RT is warranted to identify the optimal tissue types that may benefit from onboard MRI.
We have conducted a physician-based comparison for visualization of patient anatomy between onboard MR images acquired with a hybrid low-field MRI-RT system and OB-CT images acquired as standard of care. We sought to evaluate the utility of onboard MRI for anatomic visualization of target and critical structures for a preliminary investigation of localization and onboard image segmentation.
Material and methods
Patient and clinical imaging
Fourteen patients undergoing fractionated RT for cancer in the thorax (n = 2), pelvis (n = 6), abdomen (n = 3) or head and neck (n = 3) were enrolled onto an Institutional Review Board (IRB)-approved protocol for MRI with the ViewRay device (ViewRay Inc., Cleveland, OH, USA). Ten of the 14 patients had intact tumors. The hybrid MRI-treatment unit is comprised of an open, split-solenoid low-field MRI co-registered to a three-head 60Co γ-ray radiation delivery device. The MRI has nominal field strength of 0.35T and is a variant of the Siemens MAGNETOM product used for intraoperative imaging. A full description of the device and treatment process has been described by Mutic et al. [Citation9]. The onboard MRI system was used to image each patient prior to installation of source heads on the machine. All patients received additional standard of care image-guided treatment with routine daily/weekly onboard CT imaging on the TrilogyTM (Varian Medical Systems, Palo Alto, CA, USA) or the Tomotherapy® (Accuray Inc., Sunnyvale, CA, USA) treatment systems.
MRI and data
During each imaging session, patients were laid flat on the MRI table and fitted with a 12-channel torso or 11-channel head and neck receiver coil. Patients were positioned on the center of the table and their treatment position was reproduced as closely as possible, with feet secured together and arms placed on their chest or above their head and treatment site longitudinally aligned with the imaging/treatment isocenter. Patient-specific immobilization devices were not used due to size constraints and/or MRI-compatibility issues. Volumetric axial-plane MR images were acquired for each patient using a fast gradient echo sequence (TrueFISP or turbo FLASH) [Citation10] with parallel imaging techniques, as set by the manufacturer. Each image set was acquired with an in-plane resolution of 1.5 × 1.5 mm, slice thickness of 1.5 mm (n = 10) or 3.0 mm (n = 4), and field of view of 400–540 mm × 228–449 mm × 264–432 mm in the lateral, anterior-posterior, and superior-inferior directions, respectively. Each volumetric scan lasted 1–3 minutes.
OB-CT data
Cone beam CT (CBCT) and mega-voltage CT (MVCT) image sets used for routine treatment localization were acquired on the Trilogy or Tomotherapy treatment systems within one week (11/14 patients) or two weeks (3/14 patients) of MRI for each patient. CBCT image sets (n = 13) were acquired with an in-plane resolution of 0.7–1.2 mm, slice thickness of 2–2.5 mm, and a radial field of view of 250 mm (n = 2), 450 mm (n = 9), or 465 mm (n = 2). One patient being treated for a neck sarcoma on Tomotherapy was imaged with MVCT, acquired with an in-plane resolution of 1.5 mm, slice thickness of 4 mm, and radial field of view of 400 mm.
Evaluation
For each of the 14 patients, the volumetric onboard MRI and OB-CT image sets were displayed side-by-side on clinical image viewing software (Pinnacle, Philips Healthcare) and independently reviewed by three experienced radiation oncologists. Each physician was given a survey and instructed to indicate which image set (if either) offered better visualization of the target and individual organs at risks (OARs), as derived from a standardized list of site-specific critical structures [Citation11]. For each image set pair, physicians recorded one of four responses for each structure: “Better visualized on OB-MRI”, “Better visualized on OB-CT”, “Equivalent”, “Unable to see on either image set”. Physicians were permitted to freely scroll through axial images and adjust the zoom and window/level settings for optimal visualization. Fifteen to 24 OARs per patient were planned for evaluation, depending on the anatomical site [n = 24 for the thorax and head and neck, n = 15 for the abdomen, n = 20 for the pelvis (female), n = 21 for the pelvis (male)]. Ten of 14 target structures were evaluable (i.e. intact tumors) and included in the evaluation.
Additionally, a quantitative segmentation study was performed to assess if OB-MRI could offer improved structure localization between independent observers. The abdomen was chosen as the focus of this secondary study. Three physicians performed manual segmentation of eight abdominal OARs (colon, duodenum, kidney, liver, small bowel, stomach, spinal cord) and a target structure on OB-CT and OB-MRI image sets for two patients. Contours were compared between the three physicians for OB-CT and OB-MRI image sets to assess inter-observer agreement.
Analysis
To assess the overall level of agreement among physician ratings, the proportion of structures resulting in majority (2/3 physicians) and unanimous consensus (3/3 physicians) agreement was computed. Additionally, agreement was measured by computing Fleiss’ κ, a commonly used statistical metric of inter-rater reliability [Citation12]. The proportion of structures rated as better visualized on MRI or OB-CT was computed overall, and by anatomical site. To investigate if visualization was dependent on the type of structure, ratings were also analyzed after stratifying structures into five categories: soft tissue, central nervous system (CNS), vasculature, bone, pulmonary/airways, and target structure. One-way χ2 statistical testing (α = 0.05) was performed to test for significant differences between the proportion of structures better visualized on OB-MRI and the proportion better visualized on OB-CT (overall, stratified by anatomical site, and stratified by structure type). To assess inter-observer localization agreement of segmented structures, a baseline structure was constructed using the STAPLE method for each set of three physician contours [Citation13], and the centroid difference and Dice coefficient (a measure of structure overlap) [Citation14] was computed for each OB-CT structure and each OB-MRI structure.
Results
Overall, 296 structure pairs were planned for evaluation on 14 OB-MRI/OB-CT images set pairs. Seven of these structures were not evaluable due to previous surgical removal. Fifty-eight of the remaining 289 structures were not within the field of view of the OB-CT image sets (n = 49, 17%), MRI image sets (n = 1, < 1%), or both image sets (n = 8, 3%), and were omitted from the evaluation. Thus, 231 structure pairs were evaluated in total (nthorax = 46, npelvis = 70, nabdomen = 48, nhead and neck = 67) by each of the three physicians.
Agreement between the independent evaluations by physicians was high, indicating a high degree of inter-rater reliability. Physicians agreed in majority and in unanimous consensus for > 99% and 74% of cases, respectively. In a single case, the three physician ratings were split between three categories, yielding no majority result. Fleiss’ κ was calculated as 0.63, indicating “substantial” agreement between physicians. The following results are represented by majority agreement, unless otherwise stated.
Twenty-two (10%) structures were better visualized on OB-CT and 163 (71%) structures were better visualized on OB-MRI, which was found to be a significant difference (p < 0.001). For thorax, pelvis, abdomen, and head and neck sites, the proportion of structures better visualized on OB-MRI were 63, 63, 77, and 79%, respectively (), and significantly greater than the proportion of structures better visualized on OB-CT by both majority and unanimous agreement (p < 0.001). Consensus was not reached for one thorax structure (the trachea), as reflected in . Structures that were consistently better visualized on OB-MRI 100% of the time are displayed in .
Table I. List of evaluated OARs, by site. OARs which were better visualized on MRI or OB-CT for 100% of cases are indicated. OARs visualized equivalently on MRI and OB-CT (or better in some cases but not in others) are listed at the bottom of the table.
Stratification of ratings based on structure-type categories revealed stronger trends (). OB- MRI provided better visualization for a vast majority of soft tissue (92%), vasculature (94%), and CNS structures (100%). The MV-CT image set provided better visualization for two of 98 soft tissue structures (the larynx and the glottis), however these two structures were better visualized on MR image sets when compared to CBCT. The remaining 6% of structures in each of the soft tissue and vasculature categories were evaluated as “unable to see on either image set”.
Bony anatomy was better visualized on OB-CT for 48% of structures, and equivalently visualized on both OB-MRI and OB-CT for 52% of structures. All pulmonary structures were visualized equivalently on OB-MRI and OB-CT datasets, although majority agreement was not reached for one pulmonary structure (the trachea), as reflected in .
Target structures varied in location and size, and included three thoracic tumors, three pelvic tumors, two abdominal tumors, and two head and neck tumors. Four of 10 target structures were better visualized on OB-MRI, one was visualized equivalently on OB-MRI and OB-CT, and five were not visualized on either. Targets were never better visualized on OB-CT, as compared to OB-MRI. Physicians were in unanimous agreement for five of 10 target evaluations (). Examples of MRI and OB-CT image sets are displayed for four targets in .
Figure 3. Example OB-MRI/OB-CT image set pairs for four targets: lumpectomy cavity, lung tumor, liver tumor, pancreas tumor (from top to bottom). Contours as drawn by a physician on the OB-MRI (left) and OB-CT (right) image sets are shown for the liver and pancreas cases. Images were selected to display the target, and may not necessarily capture the same exact anatomical slice.
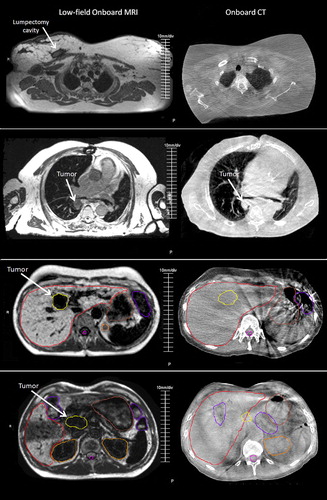
Table II. Majority and physician-specific ratings for the 10 evaluated targets.
Quantitative analysis of physician contours for two abdominal patients demonstrated that OB-MRI improved centroid agreement for the kidneys, liver, stomach and spinal cord as compared to OB-CT by up to 1 cm (). Structure overlap as indicated by the Dice coefficient was also improved for all four structures, for one or both patients (). Comparatively, inter-observer centroid agreement and structure overlap was not improved for the colon, duodenum and small bowel. Inter-observer centroid agreement was improved for the liver target by up to 2 mm (μall physicians = 1 mm); comparatively, centroid agreement was worse for the pancreatic target (). These targets can be seen in .
Table III. Centroid agreement and Dice coefficients (indicating overlap) for structures from two abdominal patients contoured by three physicians.
Discussion
Results provide preliminary evidence that onboard low-field MRI offers better visualization for selected RT targets and most critical structures compared to OB-CT. Soft tissue, vasculature, and CNS structures were consistently better visualized on OB-MRI. Pulmonary structures such as the lungs and airways were comparably visualized for OB-MRI and OB-CT. OB-CT never outperformed OB-MRI for visualization of pulmonary structures, which is an interesting finding since CT is generally well suited for imaging high-contrast interfaces. A quantitative analysis of segmented abdominal structures provides evidence that OB-MRI may improve patient positioning for some abdominal targets and certain structures. Inter-observer centroid agreement for the liver tumor analyzed was improved by 2 mm for 2/3 physicians, suggesting that OB-MRI may also be advantageous in reduced margin planning for certain targets. Results also suggest that OB-CT may be more advantageous for localizing targets marked by internal fiducials, such as the pancreatic case presented in this study ().
This study was performed in the midst of installation of the first clinically available MRI-guided radiation device. The goal of the study was a physician-based evaluation of the localization images compared with other devices in the clinic; and most importantly to decide whether further interest in this line of research was worth the investment. Other onboard MRI devices under development should also be considered as they become commercially available. 1.5T onboard MRI may offer some different tradeoffs compared to a low-field system, as it offers higher signal-to-noise, but more technical challenges and is prone to greater geometric distortions due to higher field strength [Citation15].
OB-MRI may be particularly useful for some specific indications, including improving visualization for solid tumors in the head and neck, liver, and lumpectomy cavity in the breast. There was some discordance among physicians on the usefulness of OB-MRI images for providing visualization of targets in the anus, cervix, esophagus, and pancreas. The anal and esophageal targets were smaller and less discreet compared to the other targets evaluated, possibly causing them to be less easily visualized by physicians. The pancreatic tumor () was not seen by 2/3 physicians, possibly due to a metal clip implanted at the target site which created an image artifact. For those targets not as easily visualized, it is possible that the MRI sequence used was not tailored to optimize tumor-specific imaging, as MRI sequence acquisition was limited to pre-programmed sequences available on the commercial software. Optimization of onboard MRI imaging techniques and sequences should be investigated in future work.
OB-CT may offer benefit when imaging high-density structures. While OB-MRI provided equivalent visualization for a majority of bony anatomy, OB-CT was evaluated as superior for a considerable number of bony structures; specifically the ribs and some vertebral bodies. In one comparison case, OB-CT provided better visualization for two soft tissue structures – the larynx and the glottis – which are partially composed of cartilage. However, in the remaining two cases, the larynx and glottis were evaluated as better visualized on OB-MRI. As the majority of IGRT localization is currently based on boney anatomy, this will require a change in technique when using onboard MRI. Fortunately, alignment for the spinal cord can be done directly with the MRI without reliance on vertebral bodies.
Enhanced anatomical visualization suggests that OB-MRI may be better suited than OB-CT for adaptive RT as well. MRI is known to improve the delineation of targets in the head and neck, central nervous system, and pelvis for RT treatment planning [Citation1,Citation2], and may potentially replace CT in the future as the primary planning image set for some treatment sites. Efforts are currently underway to demonstrate the feasibility of MRI-only treatment planning. Research investigating techniques for creating pseudo-CT models from MRI data has been underway, and studies have found this approach to be applicable for MRI-only treatment planning [Citation16,Citation17]. Jonsson et al. performed a planning study for 40 patients with targets in the prostate, lung, head and neck or brain, and found that MRI-only treatment planning could be performed with a maximum dosimetric error of 1.6% [Citation18]. Similar results have been reported by other studies investigating prostate RT planning [Citation19–21], which has been the most frequently studied site for MRI-based planning and a likely target for its early clinical use. A publication by Kapanen et al. describes the commissioning of a RT process for prostate treatment using MRI-only planning, which authors conclude is sufficiently accurate to replace CT-based planning and slated for use in their clinic [Citation22]. Additionally, work has been done exploring the use of MRI for image guidance through co-registration with onboard CT images. Investigators have demonstrated the feasibility of this approach, which further supports the use of MRI-only treatment simulation and planning [Citation23,Citation24].
There is much more work necessary to fully evaluate low-field onboard MRI for radiation therapy delivery. Establishing MRI sequences for optimal visualization of different organ sites will be very important. Specific organ site tasks (localization, segmentation, adaptive planning) should also be evaluated in larger populations. Comparison of this technology with high-field MRI-guided RT devices (still only in development) will allow the field to assess overall costs and benefits of different approaches to MRI-guided radiation therapy.
There are some specific limitations to this study. First, qualitative physician-based evaluation is subjective in nature. However, results of Cohen's κ indicate a substantial level of agreement between independent reviews performed by the three physicians, suggesting that results are generalizable across multiple observers. Results may not necessarily be generalizable across the general patient population. In particular, our ability to assess visualization of all types of tumors was limited since only 10 patients had evaluable target structures. Still, the sample comprised a good variety of targets, varied in size, shape, location and composition. We were also able to evaluate a large variation of critical structures by selecting patients with different treatment sites. Utilizing a published structure taxonomy ensures that this evaluation can be widely interpreted in standard clinical context [Citation11].
Perhaps the major limitation of this study was that MRI of patients was performed without the use of treatment immobilization devices, which precluded comparative plan generation and comparison. Additionally, OB-MRI and OB-CT image sets were acquired up to 12 days apart, and thus growth or shrinkage of the observed anatomy may have occurred. However, no correlation was observed between the amount of time between image set acquisition and visualization outcome, suggesting that this was not an important factor. With the first clinical treatments underway, subsequent imaging studies are now being performed at our clinic with patients in MRI-compatible immobilization devices suitable for clinical use.
While this should be considered preliminary work, our results suggest that low-field MR provides better anatomic visualization of many RT targets and many organs at risk as compared to CBCT/MVCT, which is currently the clinical standard for volumetric localization. This finding was consistent across all major anatomical sites (thorax, pelvis, abdomen, and head and neck), and supports implementation of this technology for visualization of RT targets and critical structures in the clinic.
Acknowledgments
This publication was supported by the Washington University Institute of Clinical and Translational Sciences grant UL1 TR000448, sub-award TL1 TR000449, from the National Center for Advancing Translational Sciences. The content is solely the responsibility of the authors and does not necessarily represent the official views of the NIH. The following authors report financial relationships relating to the work under consideration for publication: Camille E. Noel, Ph.D. – Grant (NIH); Parag J. Parikh, M.D. - Grant to Institution (ViewRay, Inc.); Provision of equipment and technical support; Olga L. Green, Ph.D. – Consulting Fee or Honorarium (ViewRay, Inc.); Sasa Mutic, Ph.D. – Grant to Institution (ViewRay, Inc.); Consulting Fee or Honorarium (ViewRay, Inc.); Provision of equipment and technical support (ViewRay, Inc.). The following authors report financial relationships outside of the submitted work: Sasa Mutic, Ph.D. – Grants/Grants Pending (Varian Medical Systems, Elekta); Payment for lectures including service on speakers bureaus (Varian Medical Systems); Consulting/Advisory Committees (ViewRay, Inc.); Stock/Stock Options (Radialogica, LLC; TreatSafely LLC); Payment for lectures including service on speakers bureaus (Varian Medical Systems; ViewRay, Inc.; Philips Healthcare); Parag J. Parikh, M.D. - Grants/Grants Pending (Varian Medical Systems, Philips Healthcare); Payment for lectures including service on speakers bureaus (Varian Medical Systems); Stock/Stock Options (Holaira); Jeffrey R. Olsen, M.D. – Grants/Grants Pending (ViewRay, Inc., RadioMed, Inc.); Consulting Fee or Honorarium (ViewRay, Inc.). Authors not listed report no financial relationships.
Declaration of interest: The authors report no conflicts of interest. The authors alone are responsible for the content and writing of the paper.
References
- Khoo VS, Dearnaley DP, Finnigan DJ, Padhani A, Tanner SF, Leach MO. Magnetic resonance imaging (MRI): considerations and applications in radiotherapy treatment planning. Radiother Oncol. 1997;42:1–15.
- Devic S. MRI simulation for radiotherapy treatment planning. Med Phys. 2012;39:6701–11.
- ViewRay™ Announces World's First Patients Treated Using MRI-Guided Radiation Therapy PR Newswire2014. 2014, February 5. Available from: http://www.prnewswire.com/news-releases/viewray-announces-worlds-first-patients-treated-using-mri-guided-radiation-therapy-243806571.html. Accessed 2014, February 7.
- Raaymakers BW, Lagendijk JJ, Overweg J, Kok JG, Raaijmakers AJ, Kerkhof EM, et al. Integrating a 1.5 T MRI scanner with a 6 MV accelerator: proof of concept. Phys Med Biol. 2009;54:N229–37.
- Fallone BG, Murray B, Rathee S, Stanescu T, Steciw S, Vidakovic S, et al. First MR images obtained during megavoltage photon irradiation from a prototype integrated linac-MR system. Med Phys. 2009;36:2084–8.
- Kron T, Eyles D, John SL, Battista J. Magnetic resonance imaging for adaptive cobalt tomotherapy: A proposal. J Med Phys. 2006;31:242–54.
- Dempsey JF, Benoit D, Fitzsimmons JR, Haghighat A, Li JG, Low DA, et al. A Device for Realtime 3D Image-Guided IMRT. Int J Radiat Oncol Biol Phys. 2005;63:S202.
- Jaffray DA, Carlone M, Menard C, Breen S. Image-guided radiation therapy: emergence of MR-guided radiation treatment (MRgRT) systems. Proc SPIE 7622, Medical Imaging 2010:Physics of Medical Imaging, 762202 (March 18, 2010).
- Mutic S, Dempsey JF. The ViewRay system: magnetic resonance-guided and controlled radiotherapy. Semin Radiat Oncol. 2014;24:196–9.
- Barkhausen J, Ruehm SG, Goyen M, Buck T, Laub G, Debatin JF. MR evaluation of ventricular function: true fast imaging with steady-state precession versus fast low-angle shot cine MR imaging: feasibility study. Radiology. 2001;219:264–9.
- Santanam L, Hurkmans C, Mutic S, van Vliet-Vroegindeweij C, Brame S, Straube W, et al. Standardizing naming conventions in radiation oncology. Int J Radiat Oncol Biol Phys. 2012;83:1344–9.
- Landis JR, Koch GG. The measurement of observer agreement for categorical data. Biometrics. 1977;33:159–74.
- Warfield SK, Zou KH, Wells WM. Simultaneous truth and performance level estimation (STAPLE): an algorithm for the validation of image segmentation. IEEE Trans Med Imaging. 2004;23:903–21.
- Zou KH, Warfield SK, Bharatha A, Tempany CM, Kaus MR, Haker SJ, et al. Statistical validation of image segmentation quality based on a spatial overlap index. Acad Radiol. 2004;11:178–89.
- Baldwin LN, Wachowicz K, Thomas SD, Rivest R, Fallone BG. Characterization, prediction, and correction of geometric distortion in 3 T MR images. Med Phys. 2007;34: 388–99.
- Johansson A, Garpebring A, Karlsson M, Asklund T, Nyholm T. Improved quality of computed tomography substitute derived from magnetic resonance (MR) data by incorporation of spatial information – potential application for MR-only radiotherapy and attenuation correction in positron emission tomography. Acta Oncol. 2013;52:1369–73.
- Kapanen M, Tenhunen M. T1/T2*-weighted MRI provides clinically relevant pseudo-CT density data for the pelvic bones in MRI-only based radiotherapy treatment planning. Acta Oncol. 2013;52:612–8.
- Jonsson JH, Karlsson MG, Karlsson M, Nyholm T. Treatment planning using MRI data: an analysis of the dose calculation accuracy for different treatment regions. Radiat Oncol. 2010;5:62.
- Lee YK, Bollet M, Charles-Edwards G, Flower MA, Leach MO, McNair H, et al. Radiotherapy treatment planning of prostate cancer using magnetic resonance imaging alone. Radiother Oncol. 2003;66:203–16.
- Chen L, Price RA, Jr., Wang L, Li J, Qin L, McNeeley S, et al. MRI-based treatment planning for radiotherapy: dosimetric verification for prostate IMRT. Int J Radiat Oncol Biol Phys. 2004;60:636–47.
- Lambert J, Greer PB, Menk F, Patterson J, Parker J, Dahl K, et al. MRI-guided prostate radiation therapy planning: Investigation of dosimetric accuracy of MRI-based dose planning. Radiother Oncol. 2011;98:330–4.
- Kapanen M, Collan J, Beule A, Seppala T, Saarilahti K, Tenhunen M. Commissioning of MRI-only based treatment planning procedure for external beam radiotherapy of prostate. Magn Reson Med. 2013;70:127–35.
- Korhonen J, Kapanen M, Sonke JJ, Wee L, Salli E, Keyrilainen J, et al. Feasibility of MRI-based reference images for image-guided radiotherapy of the pelvis with either cone-beam computed tomography or planar localization images. Acta Oncol. 2015;54:889–95.
- Buhl SK, Duun-Christensen AK, Kristensen BH, Behrens CF. Clinical evaluation of 3D/3D MRI-CBCT automatching on brain tumors for online patient setup verification – A step towards MRI-based treatment planning. Acta Oncol. 2010;49:1085–91.