ABSTRACT
Background. Prostate cancer is now the only solid organ cancer in which therapy is commonly applied to the whole gland. One of the main challenges in adopting focal boost or true focal therapy is in the accurate mapping of cancer foci defined on magnetic resonance (MR) images onto the computerised tomography (CT) images used for radiotherapy planning.
Material and methods. Prostate cancer patients (n = 14) previously treated at the Edinburgh Cancer Centre (ECC) were selected for this study. All patients underwent MR scanning for the purpose of diagnosis and staging. Patients received three months of androgen deprivation hormone therapy followed by a radiotherapy planning CT scan. The dominant focal prostate lesions were identified on MR scans by a radiologist and a novel image analysis approach was used to map the location of the dominant focal lesion from MR to CT. An offline planning study was undertaken on suitable patients (n = 7) to investigate boosting of the radiation dose to the tumour using a stereotactic ablative body radiotherapy (SABR) technique.
Results. The non-rigid registration algorithm showed clinically acceptable estimates of the location of the dominant focal disease on all CT image data of patients suitable for a boost treatment. Standard rigid registration was found to produce unacceptable estimates of the dominant focal lesion on CT. A SABR boost dose of 47.5 Gy was delivered to the dominant focal lesion of all patients whilst meeting all dose-volume histogram (DVH) constraints. Normal tissue complication probability (NTCP) for the rectum decreased from 1.28% to 0.73% with this method.
Conclusions. These preliminary results demonstrate the potential of this image analysis method for reliably mapping dominant focal disease within the prostate from MR images onto planning CT images. Significant dose escalation using a simultaneous integrated SABR boost was achieved in all patients.
Prostate cancer, which accounts for 25% of new male cancers, is now the only solid organ cancer in which therapy is applied to the whole gland [Citation1]. Traditionally whole gland ablative treatment with radiotherapy or surgery has been utilised due to the perception that disease is multifocal within the prostate. There has however been significant progress in detecting localised low-risk prostate cancer through prostate-specific antigen (PSA) screening and in identifying its location within the prostate gland using trans-rectal biopsies, template mapping biopsies and multi-parametric MR imaging. Identification of early disease with small dominant focal volumes opens up the possibility of truly focal treatment of the cancer within the prostate using techniques, such as radiotherapy, thus preserving genitourinary and rectal function in low- to moderate-risk prostate cancer patients [Citation2]. Alternatively a boost may be applied to the tumour whilst a tumouricidal lower dose is delivered to the rest of the prostate gland providing that dose constraints to the surrounding normal tissues are met. This form of treatment may be applicable to patients with low-risk unifocal disease and patients with high-risk multi-focal disease.
Histological validation studies have confirmed that a dominant unifocal lesion greater than 0.5 cm3 may be present in up to 70% of men newly diagnosed with localised prostate cancer [Citation3–5]. Treating the dominant focus or boosting the dose to this area while reducing the dose to as much healthy tissue as possible has significant potential for improving treatment [Citation1]. The availability of multi-parametric MR imaging, which incorporates a number of MR sequences, such as T2- and diffusion-weighted; dynamic contrast enhanced (DCE); and spectroscopy has opened up the possibility of reliably identifying cancer foci within the prostate gland [Citation6] (). However, although MR is commonly used for diagnosis, staging and performing MR-guided prostate core biopsies, it is not used routinely in radiotherapy planning to identify focal areas for localised radiotherapy in prostate cancer. With the capabilities of modern linear accelerators, which now include high-definition multi-leaf collimators (MLC), increased dose rates, six-degree of freedom couches and cone-beam computed tomography (CBCT) as standard, there are no technical barriers to delivering radiotherapy to small focal lesions within the prostate gland [Citation7].
Figure 1. Left: Radiotherapy planning CT acquired on a Philips Brilliance Big Bore CT scanner fitted with a flat couch. Right: T2-weighted MR image set acquired on a Siemens Symphony 1.5T scanner without a flat couch, which has been co-registered to the planning CT volume so that the image sets have the same resolution and geometry. The bladder, prostate and rectum contours were originally defined on the planning CT and then mapped to the MR using a rigid registration transform. It is clear from the position of these structures on the MR images that rigid registration results in significant errors in cross-modality mapping.
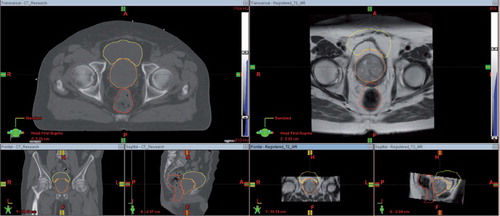
Mapping the dominant cancer foci defined on diagnostically acquired MR images, where there is no haemorrhage or distortion as a result of biopsy and gold marker implantation, onto CT is essential for radiotherapy planning. However, this is technically challenging. The focus of this paper, and our research, is in developing novel image analysis techniques to help identify the dominant tumour focus on MR and then to automatically and accurately replicate these volumes on the planning CT scan in all dimensions to allow truly focal boost or focal only therapy. Here, the image analysis methodology was tested in a planning study that shows the potential tumour dose escalation that could be achieved using a SABR technique combined with a simultaneous integrated boost (SIB) (SABR-SIB), when the dominant focal lesion was accurately mapped from MR to the radiotherapy planning CT images.
In this pilot study a novel image analysis/registration algorithm was developed to map dominant focal cancerous lesions identified on diagnostic pre-biopsy T2-weighted MR images, where they are visible, onto radiotherapy planning CT images, where they are not visible. Using a SABR-SIB planning approach dose escalation was applied to the CT images where the dominant focal lesion was obtained from the MR images. The primary objectives were two-fold: 1) to determine if the proposed registration algorithm provides superior and reliable automatic mapping of focal prostatic tumour volume identified on T2-weighted MR images to radiotherapy planning CT images; and 2) to determine if this registration method would permit reliable localised dose-escalation.
Material and methods
Clinical data acquisition and planning
Archival data from 14 prostate cancer patients previously treated at the ECC with whole gland radiotherapy were selected for the study. The planning CT images, diagnostic T2-weighted MR images, radiotherapy treatment plan, and clinical structure set were available for each case. Pixel resolution in the transaxial plane was in the range 0.84–1.19 mm for CT and 0.39–0.70 mm for MR. All patients had gold fiducial markers implanted for treatment verification prior to the acquisition of the planning CT and received androgen deprivation therapy in the time between the acquisition of the MR and CT images. Each patient was treated using a volumetric arc therapy (VMAT) technique where the prescription dose was chosen from one of the two arms of the Hypofractionated High Dose Intensity Modulated Radiotherapy for Prostate Cancer (CHHIP) trial protocol [Citation8]. Specifically, 57 Gy was prescribed in 19 fractions (fx) to the whole prostate including the seminal vesicles, or 74 Gy was prescribed in 37 fx with 74 Gy prescribed to the whole prostate and 60 Gy prescribed to an expanded volume including the seminal vesicles.
The Registration Framework
Image registration, or image fusion, is the process of aligning images acquired at different time points or from different modalities in order to establish the correspondence between complementary image features so that direct comparisons can be made [Citation9]. Rigid transformation methods usually involve translating and rotating one image to find the optimal correspondence in the other. An example of a rigid registration used in clinical practice is shown in where a radiotherapy planning CT image and diagnostic T2-weighted MR image have been co-registered. From the position of the bladder, prostate and rectum contours originally defined on the planning CT and mapped to the MR it is clear that rigid registration results in significant errors in cross-modality mapping.
Non-rigid, or deformable, registration is more appropriate for matching soft tissue structures that have undergone elastic deformation between image acquisitions [Citation10–14]. However, non-rigid registration is not used as extensively as it could be due to the lack of confidence in the mapping between the reference and floating image. This is because there is no guarantee that a feature, or detail, on the reference image will be present on the target image and therefore an approximation has to be made based on the optimal correspondence between the two images. The constraints used to define the allowable range of movement of any single feature, or pixel, also play an important role in defining the final outcome. For example, as shown in (left), when using a rigid registration approach to map the position of a dominant focal lesion firstly identified on a diagnostic MR onto a radiotherapy planning CT, it is possible that the lesion will be placed outside of the prostate volume on the CT. By calculating the non-rigid deformation pattern that the prostate undergoes between MR and CT acquisition it is possible to obtain a more logical position for the focal lesion inside the prostate, as demonstrated in (right). This is because each point in the MR prostate volume has a unique position in the CT prostate volume and therefore voxels inside the MR volume will remain inside the CT volume.
Figure 2. Left: Section of a T2-weighted MR image showing the prostate (red) and focal lesion (blue). Right: Section of the planning CT image with prostate (red) and rigidly registered focal contour (blue), which lies outside of the prostate volume. The result of non-rigidly registering the focal lesion (yellow) using the same deformation pattern that the prostate has undergone between MR and CT acquisition places the focal lesion inside the prostate.
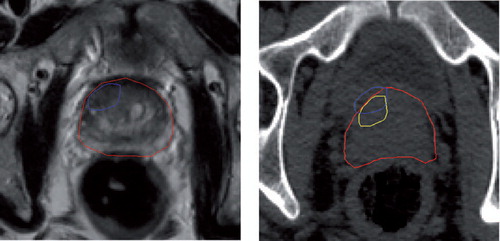
Regardless of the approach used for image registration the underlying registration mechanism remains unchanged. Typically, given a fixed reference image and a floating image, image registration is the process of finding the transformation T that maximises a measure of similarity, or the match between common features, on two images. However, it is often difficult to identify suitable features acquired on different imaging modalities because of differences in resolution, scale and position. The algorithm developed here overcomes these issues and has the ability to perform rigid only registration in situations where there is limited distortion, or rigid combined with non-rigid registration when there is significant distortion.
The rigid registration method presented here contains four key stages: pre-processing; feature extraction with the scale invariant feature transform (SIFT); calculation of the mutual information (MI); and optimisation [Citation15,Citation16]. De-noising, resampling of the original data set and the down-sampling of the resulting images were included in the pre-processing stage. To ensure a one-to-one mapping between the pixels on the MR and CT images bilinear interpolation was used to resample the images to have the same resolution and geometry. Common scale invariant features were identified on the reference and floating images using the SIFT method [Citation15]. Using
a difference of Gaussian filtering function the SIFT method detects key points on the MR and CT images that are invariant to changes in scale. The most stable key points, or features, across all scales are used to map information between the MR and CT images. To reduce the error between corresponding feature points due to the difference in MR and CT image resolution, the feature points were expanded into three-dimensional (3D) cubes. Each cube, which was made up of 3×3×3 pixels, removed the sensitivity of the approach to the pixels surrounding each SIFT feature point. The MI of each feature point and neighbouring volume on the reference and target image was calculated to assess the degree of shared information between them. The matching of points was performed over a 7D parameter space involving translations (Tx, Ty, Tz), rotations (Rx, Ry, Rz) and a scale factor . From this the optimum rigid transform between the reference and target image was found by maximising the MI of corresponding cubes.
Non-rigid registration was applied after rigid registration to form a general framework for dealing with image distortion between MR and CT images of the prostate. A commonly used interpolation technique for deformable registration is the cubic B-spline interpolation method [Citation17]. This method was used to control the smooth transformation of the target image, in this case the rigidly obtained MR image, by making appropriate adjustments to the coefficients of the B-spline parameters (basis functions). Here the transformation was driven by optimisation of the previously calculated SIFT features and neighbouring volumes.
The registration framework developed here and used to establish the mapping between dominant cancerous foci on MR and CT comprises two stages: 1) Rigid registration using 3D SIFT-based features identified on the reference and target image and an MI-based similarity function for identifying the best match between 3D SIFT features on the reference and target images; and 2) Part 1 is followed by the application of a cubic B-spline approach to non-rigidly establish a mapping between the reference and target image. This stage also contains a constraint to ensure that the dominant focal lesion remains inside the prostate volume after the non-rigid transformation and avoids the issue highlighted in .
Integrated focal boost planning
Using the Varian EclipseTM treatment planning system (Varian Medical Systems, Inc. Palo Alto, CA, USA) SABR-SIB plans were produced for the seven patients who were originally treated with 57 Gy in 19 fx to a single volume. Patients in the 74 Gy in 37 fx cohort had more advanced disease with much larger focal lesion volumes and were therefore not suitable candidates for SABR-SIB. Dual arc VMAT deliveries using 10 MV flattening filter free (10FFF) beams were planned for all cases and a plan comparison was made with the original whole prostate plans used to treat each patient.
The prostate clinical target volume (CTV) was identified on the planning CT images as the prostate plus seminal vesicles. For the SABR-SIB plans, a prostate CTV-planning target volume (PTV) margin of 5 mm in all directions except for 3 mm posteriorly was used to define the PTV(prostate). This margin was smaller than the 10 mm in all directions except for 5 mm posteriorly used in the original treatment plans. The dominant focal lesion CTV was identified on the planning CT using the image registration process presented. A uniform margin of 3 mm was applied to the CTV to form the PTV(focal) and the PTV(focal) was subtracted from the PTV(prostate). The rectum, bladder and femoral heads were manually outlined according to ECC protocols.
A radiation dose of 36.25 Gy in 5 fx was planned to the whole prostate according to the guidelines set out in the PACE trial [Citation18] with an SIB dose to the focal lesion of 47.5 Gy. The organ at risk (OAR) constraints from the PACE trial were also applied () [Citation19]. The planning aim was to cover 95% of the PTV(focal) with 95% of the boost dose and 100% of the boost dose to at least 95% of the CTV(focal). To meet these criteria plans were normalised so that 36.25 Gy covered 95% of the PTV(prostate).
Table I. The OAR planning constraints used for the SABR-SIB plans, which were taken from the PACE radiotherapy trial [Citation13].
The original whole prostate plans selected for this study satisfied the CHHIP trial constraints. The SABR-SIB plans were compared with the original whole prostate plans by calculation of the biological parameters tumour control probability (TCP) and normal tissue complication probability (NTCP) [Citation20]. TCP was calculated for the PTV(prostate) and PTV(focal). NTCP was calculated for the rectum and bladder. The models and parameters used are summarised in . The NTCP for rectum and bladder was compared between SABR-SIB and whole prostate plans using a Wilcoxon ranked signs test (significance threshold p < 0.05).
Table II. The biological models and parameters used in calculation of the TCP and NTCP between SABR-SIB plans and the original whole prostate plans [Citation22–24].
Results
Non-rigid registration
An example of a typical focal lesion within the prostate on a T2-weighted MR slice mapped to the corresponding CT slice by the registration framework is shown in . The result of a standard rigid registration results in the dominant focal lesion being placed outside of the prostate volume on CT (blue). The result of using the framework described to non-rigidly register the dominant focal lesion (yellow) using the same deformation pattern that the prostate has undergone between MR and CT acquisition places the dominant focal lesion inside the prostate. Evaluation of the contours produced by the framework was performed by an experienced radiation oncologist and the clinical acceptability of the contour recorded, as shown in . The best performing method, rigid or non-rigid, was also assessed and recorded as shown in .
Table III. The dominant focal lesion and prostate volume for all patients and the results of assessment of the mapped contours using the non-rigid method. The p-value represents the difference between the ratio of the focal disease volume to the whole prostate volume between the two prescription groups.
In 11 of the 14 cases investigated the non-rigid method gave clinically acceptable results and an overall improvement over the standard rigid method. The ratio of focal lesion volume to the prostate volume was statistically significantly smaller in the 57 Gy prescription group than the 74 Gy group (using a two sample t-test, with significance threshold of p < 0.05). The non-rigid result was unacceptable in all patients in the 74 Gy prescription group. With lesions that occupied a greater proportion of the prostate, patients in this group would not be suitable for treatment using a focal technique.
Planning
The results of the SABR-SIB planning study are shown in . All SABR-SIB plans met every mandatory constraint defined in and clinically acceptable PTV coverage was achieved for both the prostate and focal regions. The maximum point dose ranged from 108% to 118% of the boost dose and was located within the PTV(focal) in all cases. The comparison of NTCP and TCP between SABR-SIB plans and the original whole prostate plans is shown in . The NTCP for bladder was negligible in both cases. The NTCP for the rectum showed a statistically significant decrease with the SABR-SIB planning approach. The TCP for the whole prostate was significantly greater in the SABR-SIB plans.
Table IV. The results obtained from SABR-SIB plans produced to treat the dominant focal lesion.
Table V. Results of a comparison of NTCP and TCP between SABR-SIB plans and the original whole prostate plans.
Discussion
External beam radiotherapy is an important curative treatment modality for men with prostate cancer. There are very few men who are potentially unsuitable for treatment and therefore it will continue to play a major role. The ability to accurately target the prostate while sparing the surrounding normal tissues has resulted in a significant increase in the radiation doses that can be tolerated [Citation24,Citation25]. Radiotherapy techniques such as high dose rate (HDR) and low dose rate (LDR) brachytherapy deliver very high radiation doses to the prostate with excellent long-term cure rates [Citation26]. However not all men are suitable for these techniques and there is a clear need to deliver equivalently high radiotherapy doses using external beam to treat these men.
SABR is a hypofractionated regime where a high biological dose of radiation is delivered in 3–8 fx over a 2–3 weeks time period. Significant improvements in local control have been reported for SABR compared to more conventional radiotherapy (55–66 Gy in 20–33 fx) delivered over 4–6 weeks using conventional delivery mechanisms [Citation27,Citation28]. The problem however is that the accurate localisation of the tumour within the prostate is very difficult because the planning CT lacks soft tissue contrast. Modern multi-parametric MR, using techniques such as diffusion-weighing and dynamic contrast enhancement provide excellent soft tissue contrast, especially when it is performed pre-biopsy where image interpretation is not affected by haemorrhage. Furthermore, the ability of MR to accurately identify dominant focal prostate lesions in 3D is particularly valuable for radiotherapy planning. However, there is currently no single method capable of mapping pre-biopsy MR image information onto radiotherapy planning CT images in a reliable and accurate way. Currently clinicians use MR images to aid prostate contouring on the planning CT by visual cross-referencing of the MR to the planning CT. This technique requires significant clinical interpretation, which in turn leads to inter- and intra-operator variability in the accuracy of the prostate and tumour outlining. Larger margins are ultimately added to the CTV to account for this variability thus larger volumes of normal tissue are irradiated. The registration approach presented in this paper summarises our progress in developing an image analysis-based solution to this problem. From the preliminary results presented this approach, with further development and validation involving a much larger data set, offers a solution to the problem of accurately mapping dominant focal lesion contours from diagnostic MR onto planning CT. This will potentially enable accurate focal targeting of the localised cancerous volume as identified prior to androgen deprivation therapy, which can result in a shrinkage of the prostate and may be best characterised by a non-rigid transform as used in the registration framework. Although the method was demonstrated here using T2-weighted MR images, it could be extended to work on multiparametric images, or functional imaging, including PET.
The novel registration technique used in this study has allowed SABR-SIB plans of a clinically acceptable quality to be produced. The success of the non-rigid registration method for patients in the 57 Gy group led to SABR-SIB plans being prepared for this group. These patients had low to intermediate risk disease, while patients in the 74 Gy group had more advanced disease and subsequently a larger lesion volume on the MR images. Although these cases were used in developing and assessing the performance of the registration framework they do not represent cases that are likely to be considered for integrated boost therapy. For this reason SABR-SIB plans were produced for the 57 Gy group only. The PACE trial constraints for the bladder, rectum and femoral heads were met in all cases despite the inclusion of a focal boost at 47.5 Gy in 5 fx [biologically effective dose (BED) 348 Gy, assuming an α/β of 1.5 Gy] [Citation29]. This represents a significant dose escalation compared with the whole prostate dose of 36.25 Gy (BED 211 Gy) as specified in the trial [Citation30].
Although the planning study used only a small sample of seven patients, a range of focal lesion positions were encountered including some located posteriorly and close to the rectum. The fact that the constraints were met in all cases is encouraging, however, focal lesion position and size is likely to impact on the ability to meet OAR constraints and must be investigated on a larger group of patients. The main purpose of this study was to establish a methodology for reliably mapping the dominant tumour focus on MR onto the radiotherapy planning CT. As demonstrated by the results of the SABR-SIB plans, having confidence in the position of the dominant focal lesion makes it possible to deliver steep dose gradients to these foci and a reduced dose to the remainder of the prostate.
The ability to identify the true position of the dominant focal lesion with improved confidence on radiotherapy planning CT will allow higher doses to be delivered to the tumour, thus improving the care of patients with prostate cancer. The importance of image registration for this cannot be over-emphasised. This paper presents our progress on the development of a new registration framework and demonstrates that it is possible to treat the dominant cancer focus using a SABR boost with clinically acceptable PTV coverage and without compromising normal tissue constraints.
Acknowledgements
The authors gratefully acknowledge the support of the staff in the Department of Oncology Physics at the ECC for their help in preparation of the data used in this study. The authors are also grateful to staff in the Institute for Digital Communications, College of Science and Engineering at the University of Edinburgh for helpful discussion about this work. The work was generously supported by NHS Lothian, Edinburgh and Lothians Health Foundation (charity number SC007342), the James Clerk Maxwell Foundation, the Jamie King Uro-Oncology Endowment Fund and a University of Edinburgh Darwin Award.
Declaration of interest: The authors report no conflicts of interest. The authors alone are responsible for the content and writing of the paper.
References
- Valerio M, Emberton M, Ahmed HU. Focal therapy will become a standard option for selected men with localized prostate cancer. J Clin Oncol 2014;32:3680–1.
- Ahmed HU, Pendse D, Illing R, Allen C, van der Meulen JHP, Emberton M. Will focal therapy become a standard of care for men with localized prostate cancer? Nat Clin Pract Oncol 2007;4:632–42.
- Djavan B, Zlotta A, Kratzik C, Remzi M, Seitz C, Schulman CC, et al. PSA, PSA density, PSA density of transition zone, free/total PSA ratio, and PSA velocity for early detection of prostate cancer in men with serum PSA 2.5 to 4.0 ng/mL. Urology 1999;54:517–22.
- Scales Jr. CD, Presti Jr. JC, Kane CJ, Terris MK, Aronson WJ, Amling CL, et al. Predicting unilateral prostate cancer based on biopsy features: implications for focal ablative therapy—results from the SEARCH database. J Urol 2007;178:1249–52.
- Mouraviev V, Mayes JM, Sun L, Madden JF, Moul JW, Polascik TJ. Prostate cancer laterality as a rationale of focal ablative therapy for the treatment of clinically localized prostate cancer. Cancer 2007;110:906–10.
- Rothwax JT, George AK, Wood BJ, Pinto PA. Multiparametric MRI in biopsy guidance for prostate cancer: Fusion-guided. BioMed Res Int 2014;2014:e439171.
- Ahmad SS, Duke S, Jena R, Williams MV, Burnet NG. Advances in radiotherapy. Br Med J 2012;345:e7765.
- Dearnaley D, Syndikus I, Sumo G, Bidmead M, Bloomfield D, Clark C, et al. Conventional versus hypofractionated high-dose intensity-modulated radiotherapy for prostate cancer: Preliminary safety results from the CHHiP randomised controlled trial. Lancet Oncol 2012;13:43–54.
- Pluim JPW, Fitzpatrick JM. Image registration. IEEE Trans Med Imaging 2003;22:1341–3.
- Thor M, Petersen JB, Bentzen L, Høyer M, Muren LP. Deformable image registration for contour propagation from CT to cone-beam CT scans in radiotherapy of prostate cancer. Acta Oncol 2011;50:918–25.
- Tho rnqvist S, Petersen JB, Høyer M, Bentzen L, Muren LP. Propagation of target and organ at risk contours in radio-therapy of prostate cancer using deformable image registration. Acta Oncol 2010;49:1023–32.
- Leibfarth S, Mönnich D, Welz S, Siegel C, Schwenzer N, Schmidt H, et al. A strategy for multimodal deformable image registration to integrate PET/MR into radiotherapy treatment planning. Acta Oncol 2013;52:1353–9.
- Thor M, Bentzen L, Elstrøm UV, Petersen JB, Muren LP. Dose/volume-based evaluation of the accuracy of deformable image registration for the rectum and bladder. Acta Oncol 2013;52:1411–6.
- Thor M, Andersen ES, Petersen JB, Sørensen TS, Noe KØ, Tanderup K, et al. Evaluation of an application for intensity-based deformable image registration and dose accumulation in radiotherapy. Acta Oncol 2014;53:1329–36.
- Lowe DG. Distinctive image features from scale-invariant keypoints. Int J Comput Vis 2004;60:91–110.
- Maes F, Collignon A, Vandermeulen D, Marchal G, Suetens P. Multimodality image registration by maximization of mutual information. IEEE Trans Med Imaging 1997;16:187–98.
- Rueckert D, Sonoda LI, Hayes C, Hill DLG, Leach MO, Hawkes DJ. Nonrigid registration using free-form deformations: Application to breast MR images. IEEE Trans Med Imaging 1999;18:712–21.
- PACE study research summary. ClincalTrials.gov Identifier: NCT01584258[Internet]. [cited 2015 Apr 29]. Available from: http://public.ukcrn.org.uk/search/StudyDetail.aspx? StudyID = 12628.
- Tree A, Jones C, Sohaib A, Khoo V, van As N. Prostate stereotactic body radiotherapy with simultaneous integrated boost: Which is the best planning method? Radiat Oncol 2013;8:228.
- Li XA, Alber M, Deasy JO, Jackson A, Jee K-WK, Marks LB, et al. The use and QA of biologically related models for treatment planning: Short report of the TG-166 of the therapy physics committee of the AAPM. Med Phys 2012;39: 1386–409.
- Rancati T, Fiorino C, Gagliardi G, Cattaneo GM, Sanguineti G, Borca VC, et al. Fitting late rectal bleeding data using different NTCP models: Results from an Italian multi-centric study (AIROPROS0101). Radiother Oncol 2004;73:21–32.
- Cronqvist A-KA. Quantification of the response of heterogeneous tumours and organized normal tissues to fractionated radiotherapy. Ph.D. thesis, Stockholm University; 1995.
- Cheung R, Tucker SL, Lee AK, de Crevoisier R, Dong L, Kamat A, et al. Dose-response characteristics of low- and intermediate-risk prostate cancer treated with external beam radiotherapy. Int J Rad Oncol Biol Phys 2005;61: 993–1002.
- Sartor AO, Hricak H, Wheeler TM, Coleman J, Penson DF, Carroll PR, et al. Evaluating localized prostate cancer and identifying candidates for focal therapy. Urology 2008; 72(6 Suppl):S12–24.
- Ohori M, Eastham J, Koh H, et al. Is focal therapy reasonable in patients with early stage prostate cancer (CaP)-an analysis of radical prostatectomy (RP) specimens. J Urol 2006;175 (Suppl)507(Abstract 1574).
- White EC, Kamrava MR, Demarco J, Park S-J, Wang P-C, Kayode O, et al. High-dose-rate prostate brachytherapy consistently results in high quality dosimetry. Int J Radiat Oncol 2013;85:543–8.
- Lagerwaard FJ, Verstegen NE, Haasbeek CJA, Slotman B, Paul MA, Smit EF, et al. Outcomes of stereotactic ablative radiotherapy in patients with potentially operable stage I non-small cell lung cancer. Int J Rad Oncol Biol Phys 2012;83:348–53.
- Lanni TB, Grills IS, Kestin LL, Robertson JM. Stereotactic radiotherapy reduces treatment cost while improving overall survival and local control over standard fractionated radiation therapy for medically inoperable non-small-cell lung cancer. Am J Clin Oncol 2011;34:494–8.
- Oliveira SM, Teixeira NJ, Fernandes L. What do we know about the alpha/beta ratio for prostate cancer? Med Phys 2012;39:3189–201.
- Prostate accurately targeted radiotherapy investigation of overall treatment time – ClinicalTrials.gov Identifier: NCT01423474 [Internet]. [cited 2015 May 14]. Available from: https://clinicaltrials.gov/ct2/show/NCT01423474.