ABSTRACT
Background. Hypoxia is a characteristic feature of solid tumours that significantly reduces the efficacy of conventional radiation therapy. In this study we investigated the role of hypoxia in a stereotactic radiation schedule by using a variety of hypoxic modifiers in a preclinical tumour model.
Material and methods. C3H mammary carcinomas were irradiated with 3 × 15 Gy during a one-week period, followed three days later by a clamped top-up dose to produce a dose response curve; the endpoint was tumour control. The hypoxic modifiers were nimorazole (200 mg/kg), nicotinamide (120 mg/kg) and carbogen (95% O2 + 5% CO2) breathing, OXi4503 (10 mg/kg), and hyperthermia (41.5°C; 1 h).
Results. The radiation dose controlling 50% of clamped tumours (TCD50) following 3 × 15 Gy was 30 Gy. Giving nimorazole or nicotinamide+ carbogen prior to the final 15 Gy fraction non-significantly (χ2-test; p < 0.05) reduced this TCD50 to 20–23 Gy; when administered with each 3 × 15 Gy fraction these values were significantly reduced to ≤ 2.5 Gy. Injecting OXi4503 or heating after irradiating significantly reduced the TCD50 to 9–12 Gy regardless of whether administered with one or all three 15 Gy fractions. Combining OXi4503 and heat with the final 15 Gy had a significantly larger effect (TCD50 = 2 Gy).
Conclusions. Clinically relevant modifiers of hypoxia effectively enhanced an equivalent stereotactic radiation treatment confirming the importance of hypoxia in such schedules.
In an effort to obtain the oxygen and nutrients necessary for growth and development, solid tumours establish their own vascular supply form the host normal vessels by the process of angiogenesis [Citation1]. However, this neo-vasculature is structurally and functionally abnormal and fails to meet the demands of the growing tumour mass. As a result, regions are created within the tumour that exhibit glucose and energy deprivation, high lactate levels and extracellular acidosis, and oxygen deficiency [Citation2]. From a radiation standpoint it is the oxygen status that is the most critical. There is now definitive evidence that the presence of low oxygenation (hypoxia) has a negative impact on radiation response [Citation3]. This hypoxia is generally classified as chronic or acute in nature [Citation3,Citation4]. Chronic hypoxia results from an oxygen diffusion limitation that can be caused by abnormalities of the tumour vasculature, reduced oxygen delivery, or oxygen consumption by the non-hypoxic population [Citation3,Citation4]. Acute hypoxia, also called cyclic or transient hypoxia, is a consequence of a perfusion limitation that is believed to result from either vascular abnormalities, vessels blockage by circulating tumour or host blood cells, or high interstitial pressure [Citation3–5]. Both chronic and acute hypoxia can now be identified using non-invasive approaches [Citation3,Citation6,Citation7].
Substantial effort has been made to find clinically relevant approaches to overcome this hypoxia-induced resistance [Citation8,Citation9]. These approaches include radiosensitising the hypoxic cells using drug-based approaches, such as nimorazole [Citation10]. Attempts have also been made to decrease the level of hypoxia by improving oxygen supply. This can be achieved by allowing the patient to breathe high oxygen content gas. However, since this will primarily affect chronic hypoxia it was suggested that such treatments should be combined with an agent that specifically targets acute hypoxia. One such agent is nicotinamide, and this has now been combined in clinical trials with carbogen breathing [Citation11,Citation12]. The use of treatments that can effectively kill hypoxic cells is another method that may be beneficial in enhancing radiation response. There are several approaches to achieve this, but our previous studies have focussed on two clinically applicable techniques. The first is to specifically damage the tumour vasculature; agents that induce such damage will shut-down tumour blood flow thus further decreasing oxygen availability and the cells most likely to die from such a treatment are those that are already oxygen depleted. Previous studies have shown this to be an effective method for improving tumour radiation response [Citation13,Citation14] and one of the most recently developed vascular disrupting agents (VDAs) in clinical evaluation is the combretastatin analogue OXi4503 [Citation15]. The second approach is to use hyperthermia, which is a powerful radiosensitiser when applied simultaneously with radiation, but an effective hypoxic cell cytotoxin when given after irradiation [Citation8].
While there is clear evidence that the presence of hypoxic cells in tumours is a negative factor limiting the efficacy of radiation applied in a conventional fractionated radiation schedule, a relatively recent modelling study suggested that such hypoxic cells in tumours may play a more significant role in influencing curability of tumours when using a stereotactic radiation schedule [Citation16]. Thus, the aim of the current study was to investigate this issue by using a range of different hypoxic modifiers to improve the response of our well established murine C3H mammary carcinoma model to a stereotactic radiation treatment. The hypoxic modifiers were the radiosensitiser nimorazole, the oxygen delivery modulators nicotinamide and carbogen breathing, the VDA OXi4503, and mild temperature hyperthermia.
Material and methods
Animals and tumour model
All experiments were performed using a C3H mammary carcinoma that was implanted in the right rear foot of 10–14-week-old female CDF1 mice. This tumour model is an anaplastic adenocarcinoma that arose spontaneously in a C3H mouse at our institute and was originally designated as HB [Citation17]; the name was changed to C3H mammary carcinoma when it was grown in the more stable CDF1 mouse variant [Citation18]. C3H mammary carcinomas do not grow in culture, thus experimental tumours were produced following sterile dissection of large flank tumours as previously described [Citation19]. Basically, macroscopically viable tumour tissue was minced with scissors and 5–10 μl of this material implanted into the foot. Treatments were started when foot tumours reached a volume of 200 mm3, which generally occurred within 3 weeks after inoculation; tumour volume being determined by the formula D1 × D2× D3×π/6 (where the D values represent three orthogonal diameters). All animal studies were conducted according to the animal welfare policy of Aarhus University (http://dyrefaciliteter.au.dk), and with the Danish Animal Experiments Inspectorate's approval.
Drug preparation
Nimorazole was supplied by Centaur Pharmaceuticals Ltd. (Mumbai, India), nicotinamide was purchased from Sigma Chemical Co. (St. Louis, MO, USA), and OXi4503 was supplied by OXiGENE Inc. (South San Francisco, CA, USA). All drugs were freshly prepared before each experiment by dissolving in a sterile saline (0.9% NaCl) solution. Stock solutions were kept cold and protected from light. They were injected intraperitoneally (i.p.) in a volume of 0.02 ml/g mouse body weight to give final drug doses of 200 mg/kg (nimorazole), 120 mg/kg (nicotinamide), and 10 mg/kg (OXi4503).
Carbogen breathing
Carbogen breathing was achieved by placing mice in specially constructed Lucite jigs, which allowed the animals to be restrained without the need for anaesthesia. A nozzle was attached to the head end of this jig through which carbogen (95% oxygen + 5% carbon dioxide) gas flowed at a rate of 2.5 l/min.
Hyperthermia treatment
Tumour heating was achieved as described previously [Citation20]. Essentially, non-anaesthetised mice were placed in the specially constructed Lucite restraining jigs that allowed their tumour bearing legs to be exposed and loosely attached to the jig with tape. Hyperthermia was delivered by immersing the leg into a circulating water bath (type TE 623; Heto, Birkerød, Denmark). The water bath was covered with a Lucite plate with holes allowing immersion of the foot approximately 1 cm below the water surface. Previous measurements of intratumoural temperature have shown stabilisation within 1–2 minutes to approximately 0.2°C below the water bath temperature [Citation21]. The temperature of the water bath was therefore adjusted to 41.7°C, 0.2°C above the desired tumour temperature of 41.5°C. All temperature measurements were calibrated against a certified precision mercury thermometer. Tumours were heated for a 60-minute period.
Radiation treatments
Irradiations were given with a conventional therapeutic Philips X-ray machine (230 kV; 15 mA; 2-mm Al filter; 1.1-mm Cu half-value layer; dose rate of 2.3 Gy/min) as previously described [Citation22]. Dosimetry was accomplished by use of an integrating chamber. All treatments to tumour bearing feet were administered to non-anaesthetised mice placed in Lucite jigs as described above. Tumours only were irradiated, the remainder of the animal being shielded by lead. To secure homogeneity of the radiation dose, tumours were immersed in a water bath with about 5 cm of water between the x-ray source and the tumour. The temperature of this water-bath was maintained at 25°C, which is the normal temperature of these foot tumours [Citation21].
Treatment scheduling
Tumours were irradiated with 3 × 15 Gy (each fraction given with an interval of 2–3 days over a one-week period). Three days after the final 15 Gy fraction the tumours were subjected to a varying clamped top-up radiation dose with the tumour bearing leg clamped for 5 minutes before and during irradiation. The clamped top-up treatment was given in order to produce a dose response curve, where conditions, with respect to hypoxia, were the same for all treatment groups. In the combination studies the various hypoxic modifiers were given either with each of the 3 × 15 Gy fractions or only the final 15 Gy fraction. These hypoxic modifiers were given as follows: nimorazole and nicotinamide were injected 30 and 20 minutes prior to irradiating, respectively; carbogen breathing was started 5 minutes prior to irradiation and gassing continued throughout the radiation period; OXi4503 was injected one hour after irradiation; and hyperthermia treatment started four hours after irradiating.
Tumour response endpoint
The response of tumours to treatment was assessed using a tumour control assay, as described previously [Citation20]. This involved observing mice on a weekly basis up to 90 days post-treatment and recording the number of animals in each treatment group showing tumour control within this 90-day period. The tumour control data was then subjected to logit analysis and from the radiation dose response curves the TCD50 value (radiation dose producing 50% tumour control) calculated along with the 95% confidence intervals. Statistical analysis of this data was achieved using a χ2-test, with a significance level of p < 0.05.
Eppendorf oxygenation measurements
Tumour oxygenation measurements were made as previously described [Citation22]. Basically, non-anaesthetised tumour bearing mice were transferred to the Lucite jigs and restrained as described above. A fine needle autosensitive electrode probe (Eppendorf, Hamburg, Germany) was then inserted about 1 mm into the tumour, and the needle then moved automatically through the tissue in 0.7 mm increments, followed each time by a 0.3 mm backward step prior to measurement. Response time was 1.4 seconds. After making measurements along one track in the tumour the probe was removed and repeated parallel insertions were made. The average number of tracks per tumour was six (range 4–10) with the mean total number of measurements being 74 (range 48–119). The relative frequency of the oxygen partial pressure (pO2) measurements was automatically calculated and displayed as a histogram. From the raw data various parameters may be selected, but we chose to use the percentage of pO2 values ≤ 10 mmHg because this is likely to include all the radiobiologically hypoxic cells in the tumour. Measurements were made either in control mice (thus indicative of the oxygenation status at the time of irradiating with 1 × 15 Gy), two days after giving 1 × 15 Gy (indicating the oxygenation status at the time of irradiating with the second 15 Gy fraction), or three days after giving 2 × 15 Gy (thus measuring the oxygenation status at the time of irradiating with the third 15 Gy fraction). Statistical comparisons were made using a Student's t-test after testing for variance homogeneity using an F-test, with a significance level of p < 0.05.
Results
Irradiating tumours with 3 × 15 Gy over a one-week period and then giving a clamped top-up dose three days after the final 15 Gy fraction resulted in the dose response curve shown in , with a TCD50 value of around 30 Gy (). Also shown in is the effect of combining this stereotactic radiation treatment with either one or three treatments of nimorazole. Giving a single injection of nimorazole prior to the last 15 Gy fraction resulted in a 10 Gy reduction in the TCD50 value, but this decrease was non-significant. Injecting nimorazole with all 3 fractions significantly reduced the TCD50 value when compared to the TCD50 value for radiation only. Similar results were seen when nicotinamide and carbogen were combined with the stereotactic treatment ( and ). Injecting mice with nicotinamide prior to irradiating with only the last 15 Gy fraction and allowing the animals to breathe carbogen gas before and during this irradiation resulted in a small, yet non-significant decrease in the TCD50 value. However, as with nimorazole, combining these oxygen modifiers with each of the three 15 Gy fractions was far more effective. In fact, this treatment induced some 70–80% tumour control before any clamped top-up dose was administered. As there were no tumour control values below 50% it was impossible to calculate a TCD50 value.
Figure 1. Effect of nimorazole or nicotinamide and carbogen on a stereotactic radiation treatment. C3H mammary carcinomas were irradiated with 3 × 15 Gy during a one-week period, followed three days later by a clamped top-up dose to produce a dose response curve for tumour control. (A) Nimorazole was given before either the last 15 Gy fraction (○) or all three 15 Gy fractions (●). (B) Nicotinamide and carbogen breathing were combined, and given either with the last 15 Gy fraction (○) or with all three 15 Gy fractions (●). The response for radiation only is shown in both figures (∆). Points are for an average of eight mice.
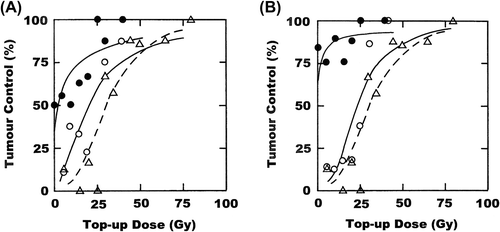
Table I. TCD50 values calculated from the data of and , and their statistical comparison.
illustrates the potential of OXi4503 to enhance tumour control in this C3H mammary carcinoma model. Injecting OXi4503 after irradiating tumours with the final 15 Gy fraction significantly reduced the clamped top-up TCD50 value to around 12 Gy (). Unlike the results with the other treatments, injecting OXi4503 after each 15 Gy fraction did not change this value; it remained at 12 Gy. Similar results were seen when tumours were given a mild temperature heating after irradiating. When combined with the final 15 Gy fraction the TCD50 was significantly reduced to around 10 Gy, but an almost identical value was obtained when the heat was applied after each 15 Gy fraction; the values obtained with a single or triple heat treatment were not significantly different (). Also shown in is the effect of combining OXi4503 and heat. When administered after the final 15 Gy fraction the TCD50 value was significantly reduced to 2 Gy (). As this was such a substantial decrease it was decided not to apply this combination with each of the three 15 Gy fractions.
Figure 2. Effect of OXi4503 or hyperthermia on a stereotactic radiation treatment. C3H mammary carcinomas were irradiated with 3 × 15 Gy during a one-week period, followed three days later by a clamped top-up dose to produce a dose response curve for tumour control. (A) OXi4503 was given after either the last 15 Gy fraction (○) or all three 15 Gy fractions (●). (B) Hyperthermia was given after either the last 15 Gy fraction (○) or with all three 15 Gy fractions (●). (C) OXi4503 and hyperthermia were combined with the final 15 Gy fraction only (●). The response for radiation only is shown in all figures (∆). Points are for an average of eight mice.
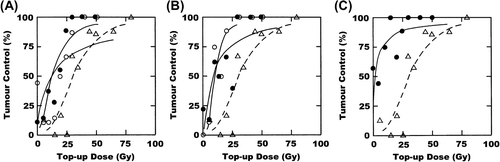
Eppendorf electrode measurements were made in our C3H mammary carcinoma at times equivalent to each 15 Gy fraction and the pooled histograms obtained are shown in . The mean (± 1 S.E.) percentage of values less than or equal to 10 mmHg measured at the time of administering the first 15 Gy fraction was found to be 70% (65–74). This was significantly reduced to 50% (45–55) two days after giving one 15 Gy fraction (equivalent to the time of the second 15 Gy fraction). However, three days after 2 × 15 Gy fractions (at a time equivalent to the last 15 Gy fraction) this value had recovered reaching 74% (68–80); it was not significantly different to the first set of measurements, but was significantly higher than the second set.
Figure 3. Tumour oxygenation status before each fraction. Representative histograms showing the oxygen partial pressure (pO2) profiles obtained in C3H mammary carcinomas with the Eppendorf oxygen electrode. Results show the relative frequency of the various pO2 values measured either under control conditions or 2–3 days after administering 1 or 2 fractions of 15 Gy. Each histogram is a summary from eight different animals.
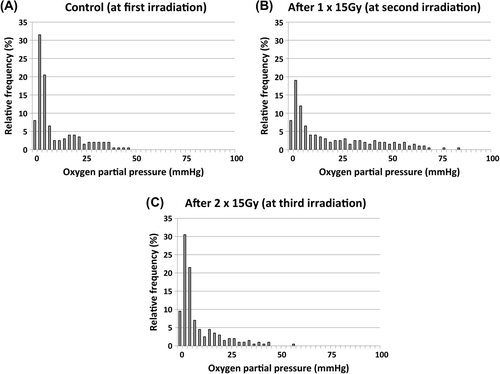
Discussion
Recent technological advancements have allowed markedly better precision when delivering radiation therapy, thereby enabling larger doses to be delivered directly to tumours with reduced doses to the surrounding normal tissues. As a result, stereotactic radiation schedules have become a desirable alternative to more often used conventional fractionation schedules [Citation16]. Numerous clinical trials using such stereotactic radiotherapy schedules have been performed, with the number of fractions and the dose per fraction being highly variable [Citation23,Citation24]. In the present study, we used a 3 × 15 Gy schedule delivered over a one-week period; such a schedule is typical of that used to treat cancer patients in our clinic [Citation25,Citation26]. We also used our C3H mammary carcinoma model, which is a model we have used extensively and is ideal for producing data using the more clinically relevant endpoint for radiation response of tumour control [Citation19,Citation20,Citation27]. This model has also been reported to result in data that is equivalent to that obtained in humans [Citation28]. However, the 3 × 15 Gy stereotactic schedule used did not result in any tumour control, thus we applied an artificial clamped top-up treatment to produce full dose response curves.
Hypoxia is a characteristic feature of most solid animal [Citation29] and human [Citation2] tumours, which has a negative impact on the ability of conventional radiotherapy to treat cancer [Citation3]. It has now been suggested that hypoxia may play a more significant role in stereotactic radiation treatments [Citation16]. In the current study, we investigated this issue by combining known modifiers of hypoxia with the 3 × 15 Gy radiation schedule. These were the radiosensitiser nimorazole; the oxygen supply modifiers nicotinamide and carbogen; the VDA OXi4503; and mild temperature hyperthermia. Our results showed that all four approaches significantly improved tumour response to the applied 3 × 15 Gy stereotactic treatment.
When using nimorazole or nicotinamide and carbogen, similar results were obtained. A small yet non-significant effect was observed when these modifiers were combined with the final 15 Gy fraction, but when administered with all three 15 Gy fractions a large and highly significant effect was obtained ( and ). Nimorazole in this latter situation resulted in some 50% of tumours being controlled even before the clamped top-up was started, while for nicotinamide and carbogen the effect was slightly larger with some 70–80% of tumours controlled. This suggests that with a single treatment of either of these hypoxic modifiers only a small percentage of hypoxic cells are eliminated and that the observed effects can be substantially increased by giving the hypoxic modifiers more often.
Our studies with the Eppendorf oxygen electrode showed that the level of tumour hypoxia at the time of administering the first 15 Gy fraction was significantly reduced when the second 15 Gy fraction was given. This is consistent with our previous study in the same tumour model where we found reoxygenation within 24 hours after a single dose of radiation [Citation30]. In fact, in that study the fraction of radiobiologically hypoxic cells decreased from about 20% to 10%. The Eppendorf measurements in our current study showed that by the time of giving the third 15 Gy fraction the level of hypoxia had returned to pre-treatment values. Whether these results suggests that rather than administering nimorazole or nicotinamide + carbogen with all three 15 Gy fractions we only needed to combine them with the first and last fraction, is not clear; although the level of hypoxia was significantly reduced at the time of the second 15 Gy fraction tumours still had a considerable level of hypoxia to target.
Although nimorazole and nicotinamide + carbogen have different modes of action the cells they affect are probably the same. Nimorazole will presumably radiosensitise any hypoxic cell whether it be acutely or chronically hypoxic, while both cell types can be targeted by combining nicotinamide and carbogen; nicotinamide has been shown to prevent the fluctuations in tumour blood flow that cause acute hypoxia and carbogen will increase the oxygen diffusion distance thus oxygenating chronically hypoxic cells [Citation31]. The failure to see any significant benefit when combining either of these modifying treatments with just a single 15 Gy fraction is in contradiction to the predicted beneficial effect for another radiosensitiser, etanidazole [Citation16]. There are several possible explanations for this apparent discrepancy. First, the radiation dose we applied was lower than the 20–25 Gy used in the modelling study. Second, etanidazole is generally considered to be a more efficient sensitiser than nimorazole [Citation32,Citation33] and while the radiosensitisation with etanidazole increases with drug dose [Citation32] the effect of nimorazole is relatively constant at drug concentrations above 100–300 mg/kg [Citation33]. The dose we used (200 mg/kg) was selected because the total dose per week in the triple treatment schedule was 600 mg/kg and in mice this is equivalent to the total weekly dose achieved in patients [Citation10,Citation34]. With nicotinamide we used 120 mg/kg. We could have also used a higher dose, but our previous studies have suggested this to be the highest maximum dose possible in humans that produces maximal enhancement of radiation damage in this C3H mammary carcinoma model [Citation35]; previous clinical studies have tended to use a reduced dose [Citation11,Citation12].
With OXi4503 and mild temperature hyperthermia the results obtained were different from those with the other two treatments. Both OXi4503 and heat resulted in similar TCD50 values in the range of 9–12 Gy. These were somewhat intermediate between those values obtained with one and three treatments with nimorazole or nicotinamide and carbogen, and in certain situations these intermediate values were significantly different from the sensitiser or oxygen modifier results (). Furthermore, identical values were found regardless of whether OXi4503 or mild temperature hyperthermia were given with one or all three 15 Gy fractions. These results suggest that only a certain percentage of hypoxic cells are killed with one drug or heat treatment, and that this effect cannot be increased by giving those treatments more often. Surprisingly, combining OXi4503 and mild temperature hyperthermia with only the final 15 Gy fraction had an even bigger effect with some 50% of tumours being controlled before the clamped top-up dose response was even initiated. This enhancement was significantly greater than all the other treatment schedules except the triple treatments with nimorazole and probably that with nicotinamide and carbogen (). The fact that a larger effect can be seen when OXi4503 and heat are combined suggests that the hypoxic cells killed by OXi4503 and mild temperature hyperthermia are not the same. It is likely that the more severely hypoxic cells close to necrosis are the ones primarily killed by OXi4503. We do not actually have any direct data to support this suggestion. In fact, measurements of tumour oxygenation with the Eppendorf oxygen electrode actually show that after treatment with OXi4503 there is a substantial increase in the percentage of low values [Citation36] consistent with vascular shut-down. But there is also a major increase in necrosis following treatment with OXi4503 (unpublished observations) and it has been suggested that necrosis can predict the effects of hypoxia-modifying therapies [Citation37] so the results are not inconsistent with our suggestion. Hypoxic cells are preferentially killed by hyperthermia, but the greatest effect was on cells at lower pH [Citation38]. Carbonic anhydrase IX is a protein involved in pH regulation [Citation39] that is upregulated in tumours [Citation40]. It has been suggested that this upregulation, and presumably associated tumour acidification, can occur under conditions of both severe (≤ 10 mmHg) and intermediate (10–30 mmHg) hypoxia [Citation40]. If heat were to preferentially kill those cells at the intermediate levels of hypoxia it would certainly explain the additional benefit when combined with OXi4503. Of course, the above suggestions only apply to possible effects on chronic hypoxia. As OXi4503 targets the tumour vascular supply it could also have a major influence on acute hypoxia; acutely hypoxic cells are unlikely to exist in regions of reduced pH and thus not influenced by heating. Clearly, additional studies are required to prove or disprove these findings.
In conclusion, this study has demonstrated that using a variety of hypoxic modifying techniques the effect of a stereotactic radiation treatment could be enhanced. This supports the earlier suggestion that hypoxia plays a significant role in determining the response to stereotactic radiotherapy. All the techniques used have undergone clinical evaluation [Citation10–12,Citation15], so any one of them or even combinations of these treatments could have a major potential application in clinical treatments where stereotactic radiation therapy is applied.
Acknowledgements
The authors thank Ms. Dorthe Grand, Ms. Pia Schjerbeck, Ms. Inger Marie Horsman and Mr. Mogens Johanssen for excellent technical assistance, and Ms. Marianne Verner Bjerre and Ms. Marianne Kristiansen for animal care. This study was made possible by financial support from the Danish Cancer Society and the Danish Council for Independent Research: Medical Sciences.
Declaration of interest: The authors report no conflicts of interest. The authors alone are responsible for the content and writing of the paper.
References
- Brem S, Brem H, Folkman J, Finkelstein D, Patz A. Prolonged tumor dormancy by prevention of neovascularization in the vitreous. Cancer Res 1976;36:2807–12.
- Vaupel P, Kallinowski F, Okunieff P. Blood flow, oxygen and nutrient supply, and metabolic microenvironment of human tumors: A review. Cancer Res 1989;49:6449–65.
- Horsman MR, Mortensen LS, Petersen JB, Busk M, Overgaard J. Imaging hypoxia to improve radiotherapy outcome. Nat Rev Clin Oncol 2012;9:674–87.
- Dewhirst MW. Concepts of oxygen transport at the microcirculatory level. Semin Radiat Oncol 1998;8:143–50.
- Jain RK. Determinants of tumor blood flow: A review. Cancer Res 1988;48:2641–58.
- Busk M, Jakobsen S, Horsman MR, Mortensen LS, Iversen AB, Overgaard J, et al. PET imaging of tumor hypoxia using 18F-labeled pimonidazole. Acta Oncol 2013;52: 1300–7.
- Nielsen T, Nielsen NC, Holm TH, Ostergaard L, Horsman MR, Busk M. Ultra-high field 1H magnetic resonance imaging approaches for acute hypoxia. Acta Oncol 2013;52:1287–92.
- Horsman MR, Overgaard J. Hyperthermia: A potent enhancer of radiotherapy. Clin Oncol 2007;19:418–26.
- Horsman MR, Overgaard J, Siemann DW. Impact on radiotherapy. In: Siemann DW, editor. Tumor microenvironment. Chichester: John Wiley & Sons, Ltd; 2011. p. 353–76.
- Overgaard J, Hansen HS, Overgaard M, Bastholt L, Berthelsen A, Specht L, et al. A randomized double-blind phase III study of nimorazole as a hypoxic radiosensitizer of primary radiotherapy in supraglottic larynx and pharynx carcinoma. Results of the Danish Head and Neck Cancer Study (DAHANCA) Protocol 5-85. Radiother Oncol 1998; 46:135–46.
- Hoskin PJ, Rojas AM, Bentzen SM, Saunders MI. Radiotherapy with concurrent carbogen and nicotinamide in bladder carcinoma. J Clin Oncol 2010;28:4912–8.
- Janssens GO, Rademakers SE, Terhaard CH, Doornaert PA, Bijl HP, van den Ende P, et al. Accelerated radiotherapy with carbogen and nicotinamide for laryngeal cancer: Results of a phase III randomized trial. J Clin Oncol 2012;30: 1777–83.
- Siemann DW, Warrington KH, Horsman MR. Targeting tumor blood vessels: An adjuvant strategy for radiation therapy. Radiother Oncol 2000;57:5–12.
- Siemann DW, Horsman MR. Vascular targeted therapies in oncology. Cell Tissue Res 2009;335:241–8.
- Patterson DM, Zweifel M, Middleton MR, Price PM, Folkes LK, Stratford MR, et al. Phase I clinical and pharmacokinetic evaluation of the vascular-disrupting agent OXi4503 in patients with advanced solid tumors. Clin Cancer Res 2012;18:1415–25.
- Brown JM, Diehn M, Loo BW, Jr. Stereotactic ablative radiotherapy should be combined with a hypoxic cell radiosensitizer. Int J Radiat Oncol 2010;78:323–7.
- Overgaard K, Overgaard J. Investigations on the possibility of a thermic tumour therapy. I. Short-wave treatment of a transplanted isologous mouse mammary carcinoma. Eur J Cancer 1972;8:65–78.
- Overgaard J. Simultaneous and sequential hyperthermia and radiation treatment of an experimental tumor and its surrounding normal tissue in vivo. Int J Radiat Oncol 1980;6:1507–17.
- Horsman MR, Murata R, Breidahl T, Nielsen FU, Maxwell RJ, Stodkiled-Jorgensen H, et al. Combretastatins novel vascular targeting drugs for improving anti-cancer therapy. Combretastatins and conventional therapy. Adv Exp Med Biol 2000;476:311–23.
- Horsman MR, Murata R. Combination of vascular targeting agents with thermal or radiation therapy. Int J Radiat Oncol 2002;54:1518–23.
- Horsman MR, Christensen KL, Overgaard J. Hydralazine-induced enhancement of hyperthermic damage in a C3H mammary carcinoma in vivo. Int J Hyperther 1989;5: 123–36.
- Horsman MR, Murata R. Vascular targeting effects of ZD6126 in a C3H mouse mammary carcinoma and the enhancement of radiation response. Int J Radiat Oncol 2003;57:1047–55.
- Solda F, Lodge M, Ashley S, Whitington A, Goldstraw P, Brada M. Stereotactic radiotherapy (SABR) for the treatment of primary non-small cell lung cancer; systematic review and comparison with a surgical cohort. Radiother Oncol 2013;109:1–7.
- Helou J, Morton G, Zhang L, Deabreu A, D’Alimonte L, Elias E, et al. A comparative study of quality of life in patients with localized prostate cancer treated at a single institution: Stereotactic ablative radiotherapy or external beam+ high dose rate brachytherapy boost. Radiother Oncol 2014;113:404–9.
- Kopek N, Holt MI, Hansen AT, Hoyer M. Stereotactic body radiotherapy for unresectable cholangiocarcinoma. Radiother Oncol 2010;94:47–52.
- Worm ES, Hoyer M, Fledelius W, Poulsen PR. Three- dimensional, time-resolved, intrafraction motion monitoring throughout stereotactic liver radiation therapy on a conventional linear accelerator. Int J Radiat Oncol 2013;86:190–7.
- Horsman MR, Chaplin DJ, Overgaard J. Combination of nicotinamide and hyperthermia to eliminate radioresistant chronically and acutely hypoxic tumor cells. Cancer Res 1990;50:7430–6.
- Nielsen T, Murata R, Maxwell RJ, Stodkilde-Jorgensen H, Ostergaard L, Horsman MR. Preclinical studies to predict efficacy of vascular changes induced by combretastatin a-4 disodium phosphate in patients. Int J Radiat Oncol 2008;70:859–66.
- Moulder JE, Rockwell S. Hypoxic fractions of solid tumors: Experimental techniques, methods of analysis, and a survey of existing data. Int J Radiat Oncol 1984;10:695–712.
- Horsman MR, Grau C, Overgaard J. Reoxygenation in a C3H mouse mammary carcinoma. The importance of chronic rather than acute hypoxia. Acta Oncol 1995;34: 325–8.
- Horsman MR, Nordsmark M, Khalil AA, Hill SA, Chaplin DJ, Siemann DW, et al. Reducing acute and chronic hypoxia in tumours by combining nicotinamide with carbogen breathing. Acta Oncol 1994;33:371–6.
- Brown JM, Yu NY, Brown DM, Lee WW. SR-2508: A 2-nitroimidazole amide which should be superior to misonidazole as a radiosensitizer for clinical use. Int J Radiat Oncol 1981;7:695–703.
- Overgaard J, Overgaard M, Nielsen OS, Pedersen AK, Timothy AR. A comparative investigation of nimorazole and misonidazole as hypoxic radiosensitizers in a C3H mammary carcinoma in vivo. Br J Cancer 1982;46:904–11.
- Overgaard J, Overgaard M, Timothy AR. Studies of the pharmacokinetic properties of nimorazole. Br J Cancer 1983;48:27–34.
- Horsman MR, Hoyer M, Honess DJ, Dennis IF, Overgaard J. Nicotinamide pharmacokinetics in humans and mice: A comparative assessment and the implications for radiotherapy. Radiother Oncol 1993;27:131–9.
- Iversen AB, Busk M, Horsman MR. Induction of hypoxia by vascular disrupting agents and the significance for their combination with radiation therapy. Acta Oncol 2013;52: 1320–6.
- Eustace A, Irlam JJ, Taylor J, Denley H, Agrawal S, Choudhury A, et al. Necrosis predicts benefit from hypoxia-modifying therapy in patients with high risk bladder cancer enrolled in a phase III randomised trial. Radiother Oncol 2013;108:40–7.
- Overgaard J, Bichel P. The influence of hypoxia and acidity on the hyperthermic response of malignant cells in vitro. Radiology 1977;123:511–4.
- Thiry A, Dogne JM, Masereel B, Supuran CT. Targeting tumor-associated carbonic anhydrase IX in cancer therapy. Trends Pharmacol Sci 2006;27:566–73.
- Rademakers SE, Span PN, Kaanders JH, Sweep FC, van der Kogel AJ, Bussink J. Molecular aspects of tumour hypoxia. Mol Oncol 2008;2:41–53.