To the Editor,
Concurrent chemo-radiotherapy (CRT) is a guideline recommended treatment for fit patients presenting with locally advanced non-small cell lung cancer (NSCLC) [Citation1]. However, for many patients, overall survival (OS) remains limited [Citation2,Citation3]. Doses of at least 60 Gy in 2 Gy/fraction are frequently used [Citation4] and clinically relevant acute radiation esophagitis (RE) is relatively common [Citation5]. RE is found to correlate with different dose-volume parameters [Citation6–10] and normal tissue complication probability (NTCP) models have been developed for RE [Citation11,Citation12].
However, strategies for esophagus sparing are not routinely used. Often, the esophagus lies in, or adjacent to, the planning target volume (PTV), leading to concerns about deliberately underdosing part of the PTV. This modeling study investigates the tradeoffs between lowering esophagus dose and tumor control. We studied patients with large PTVs (defined here as ≥700 cm3) as this is often associated with more dose to normal tissues [Citation3]. Volumetric modulated arc therapy (VMAT) plans with reduced PTV dose in the region of PTV-esophagus overlap were compared to non-esophagus sparing plans using tumor control probability (TCP), equivalent uniform dose (EUD) [Citation13], NTCP, and various dosimetric parameters. Different NTCP models were used to explore different treatment approaches, e.g. concurrent CRT or radiotherapy (RT) alone. The approach to esophagus sparing used in this study differs from previous work. Chapet et al. proposed a method for esophagus sparing lung cancer treatment with intensity-modulated radiotherapy (IMRT) and EUD-based optimization [Citation14], but they focused on the possibility of dose escalation in the PTV outside the esophagus and EUD-based optimization is not widely available. Niedzielski et al. evaluated esophagus sparing IMRT plans, but did not deliberately attempt to reduce dose in the PTV-esophagus overlap region, and did not investigate effects on TCP and NTCP [Citation15].
Materials and methods
Patient selection and contouring
Planning computed tomography (CT) scans from 10 patients who had previously undergone CRT for locally advanced NSCLC were used, with detailed patient information available in the Supplementary Table I (available online at http://www.informahealthcare.com). All had a PTV >700 cm3, and the esophagus was at least partially within the PTV [mean overlap between four-dimensional (4D) esophagus and PTV = 13.3 cm3±10.2]. All scans had been acquired using a free-breathing, non-coached 4D CT protocol (LightSpeed RT, General Electric, USA and Real-time Position Management™ system, Varian Medical Systems, Palo Alto, CA, USA). The internal target volume (ITV) was contoured and expanded by 10 mm in all directions to create the PTV. The esophagus was also contoured on all phases of the 4DCT (Eso), and expanded by 3 mm (Eso + 3) to create a planning organ at risk (OAR) volume [Citation16]. The following structures were created for planning and reporting purposes: overlap volume of PTV and Eso (Eso&PTV), PTV not overlapping Eso + 3 (PTV-Eso), Eso + 3 outside the PTV (Eso-PTV), and a 3 mm ring structure in-between Eso and PTV-Eso (Ring). A typical example of delineation is shown in the Supplementary Figure 1 (available online at http://www.informahealthcare.com).
Treatment planning
All plans were made with a two-arc VMAT (RapidArc®, Varian Medical Systems) technique [Citation17,Citation18]. Plan optimization and dose calculation [anisotropic analytical algorithm (AAA)] were performed using the Eclipse™ treatment planning system (version 10.0.28) and 6MV flattened photons (TrueBeam™, Varian Medical Systems). OAR objectives were adapted by manual interaction during optimization. A clinically acceptable reference plan [Citation18] with a prescribed dose of 60 Gy in 30 fractions and no esophagus sparing was made for all cases. Planning goals reflected our clinical practice [Citation18], minimizing volume of contralateral lung receiving >5 Gy (CL-V5) and minimizing volume of total lung minus PTV receiving >20 Gy (TL-V20) and >5 Gy (TL-V5). The goal was to cover 95% of the PTV by 95% of the prescribed dose (V95%>95%).
Scenario 1, controlled esophagus sparing: All reference plans were re-optimized to deliver 60 Gy to PTV-Eso, 50 Gy to Eso&PTV and 54 Gy to the Ring. Dose to the Eso-PTV region was kept as low as possible. All other constraints were as in the reference plan.
Scenario 2, extreme esophagus sparing: Additional plans were made for patients 1–5 in which 60 Gy was prescribed to the PTV-Eso volume, while the dose to the entire Eso was minimized, including in the overlap region with the PTV. All other constraints were applied as in the reference plan.
Data analysis and statistics
Esophagus V30, V40, V50, V60 and mean esophageal dose (MED) were recorded. NTCP for RE was calculated using two models: first, Lyman-Kutcher-Burman (LKB) using the parameters contained in the Eclipse biological evaluation module and reported by Chapet et al. [Citation11]: TD50 = 51 Gy, n = 0.44, m = 0.32. The endpoint for this model is RE grade 2 or higher, and it is derived from patients treated with RT alone or with RT and neoadjuvant chemotherapy. The second, two-variable logistic model takes into account whether or not patients receive concurrent chemotherapy. The parameters used were published by Huang et al.: β0 = −3.13, β1 = 1.50 (for concurrent CRT) and β2 = 0.0688 (for MED) [Citation9].
PTV mean dose, V95% and maximum dose were recorded. The Poisson linear quadratic (P-LQ) model [Citation19] was used to calculate TCP with parameters contained in Eclipse: D50 = 49.2 Gy, γ = 1, α/β = 10. EUD was calculated for a = −5 (EUD−5) and −20 (EUD−20), which have been described as representative for a radiosensitive and an aggressive tumor, respectively [Citation13,Citation14]. An in-house developed Matlab® (The MathWorks, Inc) routine applied to exported dose-volume histogram (DVH) data was used to derive all NTCP and TCP results and dosimetric parameters. Statistical tests were performed using IBM SPSS statistics (version 20). A paired, two-tailed Wilcoxon signed rank test (significance level 5%) was used to compare lung and PTV parameters. Esophagus parameters, TCP, NTCP and EUD were evaluated using a paired one-tailed test Wilcoxon signed rank test.
Results
PTV and lung dosimetry, and TCP and EUD in both the non-sparing and controlled sparing plans, are summarized in . In the non-sparing and the controlled sparing plans (average over all patients), the mean Eso&PTV dose was 60.8 Gy (SD = 0.6 Gy) and 52.3 Gy (SD = 0.9 Gy), respectively. This is illustrated for one patient in . In the Ring, the mean dose in the non-sparing and the controlled sparing plans was 60.7 Gy (SD = 0.6 Gy) and 54.9 Gy (SD = 0.6 Gy). A statistically non-significant decrease in TCP was observed, the mean reduction was 0.5% (range −1–3%). EUD was significantly decreased by an average of 0.4 Gy (range −0.1–1.5 Gy) and 1.5 Gy (range 0.3–4.3 Gy) for a = −5 and −20, respectively. Lung dose was slightly, but significantly, higher with the sparing strategy.
Figure 1. Dose-volume histogram (DVH) for three plans for Patient 2. Mean esophageal dose (esophagus contoured with 4D information, Eso) is reduced from an average of 29.0 Gy in the non-sparing plan to 23.5 Gy and 15.8 Gy for the controlled sparing and extreme sparing plans, respectively. The largest dose reduction takes place in the overlapping area (Eso&PTV). DVH for total lung-PTV (TL) and contralateral lung (CL) are also shown. Squares: reference plan, triangles: extreme sparing plan, dots: controlled sparing plan.
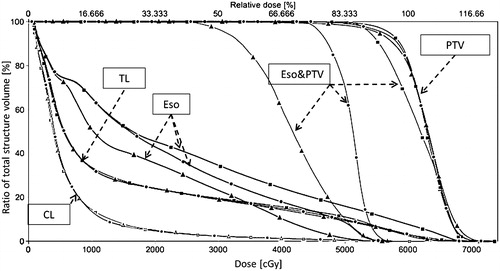
Table I. Dosimetric parameters of planning target volume and lung for controlled esophagus sparing and non-sparing plans.
Dosimetric and NTCP values for the esophagus are shown in . For the non-sparing plans, the estimated NTCP for acute RE with concurrent CRT was approximately 2.5 times higher than for RT alone, when the Huang model was applied (average NTCP 25.8% and 58.0%, respectively). With controlled sparing, the relative mean reduction in estimated risk of acute RE for the various models were: 15% (Huang concurrent CRT), 25% (Huang RT alone) and 47% (Chapet). All esophagus dosimetric and NTCP reductions were found to be significant (p < 0.01). The relationship between NTCP and the esophagus-PTV overlap is shown in Supplementary Figure 2 (available online at http://www.informahealthcare.com).
Table II. Dosimetric parameters of the esophagus for controlled esophagus sparing and non-sparing treatment plans.
Compared to the non-sparing plans, the average absolute reduction in the extreme sparing plans for esophagus V30, V40 and V50 were 11.6%, 18.5% and 23.3%, respectively (p < 0.03). V60 for the esophagus was reduced to 0%. The dose in Eso&PTV, Ring and the esophagus outside the PTV was reduced by 20.3 Gy (SD = 0.7 Gy), 8.7 Gy (SD = 0.6 Gy) and 6.0 Gy (SD = 2.8 Gy), respectively. Relative NTCP reduction for acute RE was: 28% (Huang concurrent CRT), 40% (Huang RT alone) and 73% (Chapet) (p = 0.02 for all models). The average reduction of PTV mean dose, EUD-5 and EUD-20 was 0.3 Gy (p = 0.23), 1.7 Gy (p = 0.02) and 21.6 Gy (p = 0.02), respectively. TCP was reduced by 2% for Patients 1 and 5, and 1% for Patients 2, 3 and 4.
Discussion
By minimizing the esophagus dose outside the PTV, and by prescribing 50 Gy instead of 60 Gy in the esophagus-PTV overlap region and 54 Gy adjacent to the esophagus, clinically relevant reductions in NTCP for acute RE were obtained with minimal estimated impact on TCP and EUD. The use of more extreme sparing led to a slight decrease in mean PTV dose and TCP, but unacceptably large reductions in EUD-20. These findings suggest that controlled esophagus sparing strategies merit further consideration as a way of improving the therapeutic ratio of CRT in certain groups of patients, e.g. those at high risk of acute RE and with a poorer prognosis, or those expected to tolerate acute RE poorly. We have shown that there may be some tradeoffs when sparing the esophagus, with slightly more dose in other organs (we have shown lung data, but dose to other organs such as the heart should also be evaluated).
Although different parameters have been reported as being predictive for acute RE, accurately estimating the risk for an individual patient remains a challenge. A review of various predictive models for acute RE suggested that models based on dosimetric parameters alone were likely to be too simplistic [Citation20], and the large differences in the results of the two NTCP models used in this study indicate that the output values should be regarded with caution. Nonetheless, both models predicted a clinically relevant reduction in NTCP, with minimal reduction in TCP and EUD. The dependance of NTCP on the overlapping esophagus-PTV indicates that this could be used as a first-order predictor for acute RE, after contouring, but prior to the treatment planning process.
A number of potential limitations of our study deserve comment. The effects of controlled esophagus sparing down to 50 Gy on both dosimetric parameters for the PTV as well as on TCP were found to be minimal. However, these models also need to be interpreted with caution. All the patients had a large PTV, which decreases the influence of a cold spot on the mean PTV dose. This is relevant to the TCP model, e.g. which is volume independent as the total PTV is used as the reference volume. This results in a model that loses accuracy when the patient under consideration deviates from the patients that were used for determination of the model parameters. Furthermore, the P-LQ model assumes that all voxels (or dose bins) are equally sensitive to radiation, an assumption which seems unlikely [Citation21]. Assumptions can be introduced into models to try and correct for possible spatial variation in the effect on TCP, e.g. Guckenberger et al. used a factor of 0.1 or 0.01 to correct for the lower clonogenic cell density outside the gross tumor volume (GTV) [Citation22]. If clinically validated, such models may predict that the esophagus dose inside the PTV could be lowered even further while maintaining TCP. Finally, the approach described could be adapted if there is gross tumor in contact with the esophagus, by requesting that a minimum dose is delivered to the GTV, while sparing the rest of the esophagus as much as possible.
In conclusion, current radiobiological models suggest that a strategy that prescribes 60 Gy in 30 fractions to the PTV, but reduces dose to 50 Gy in the 4D esophagus-PTV overlap region and 54 Gy in a 3 mm ring around it, while also attempting to lower dose to the esophagus located outside the PTV, can reduce the estimated risk of acute RE by a clinically meaningful amount, with relatively small effects on TCP and EUD. Appropriate studies are needed to validate the predictions of radiobiological models and to determine whether sparing strategies like the one described here might be clinically useful in appropriately selected patients.
Daan Hoffmans, Max Dahele, Suresh Senan & Wilko F. A. R. Verbakel
Department of Radiation Oncology, VU University Medical Center
De Boelelaan 1117, Amsterdam, 1081HV, The Netherlands
Supplement.docx
Download MS Word (849.3 KB)Declaration of interest
The Department of Radiation Oncology VU University medical center has research collaborations with Varian Medical Systems, Brainlab AG and Velocity Medical Solutions. Max Dahele has received travel support and honoraria from Varian Medical Systems, Brainlab AG and Lilly. Suresh Senan has received travel support and honoraria from Varian Medical Systems. Wilko Verbakel has received travel support and honoraria from Varian Medical Systems.
References
- Vansteenkiste J, De Ruysscher D, Eberhardt WEE, Lim E, Senan S, Felip E, et al. Early and locally advanced non-small-cell lung cancer (NSCLC): ESMO Clinical Practice Guidelines for diagnosis, treatment and follow-up. Ann Oncol 2013;24(Suppl 6):vi89–98.
- Bradley JD, Paulus R, Komaki R, Masters G, Blumenschein G, Schild S, et al. Standard-dose versus high-dose conformal radiotherapy with concurrent and consolidation carboplatin plus paclitaxel with or without cetuximab for patients with stage IIIA or IIIB non-small-cell lung cancer (RTOG 0617): a randomised, two-by-two factorial p. Lancet Oncol 2015;16:187–99.
- Wiersma TG, Dahele M, Verbakel WFaR, van de Ven PM, de Haan PF, Smit EF, et al. Concurrent chemoradiotherapy for large-volume locally-advanced non-small cell lung cancer. Lung Cancer 2013;80:62–7.
- Bradley J, Paulus R, Komaki R. A randomized phase III comparison of standard-dose (60 Gy) versus high-dose (74 Gy) conformal chemoradiotherapy with or without cetuximab for stage III non-small. J Clin Oncol 2013;17–18.
- O’rourke N, Roqué I Figuls M, Farré Bernadó N, Macbeth F. Concurrent chemoradiotherapy in non-small cell lung cancer. Cochrane Database Syst Rev. 2010;CD002140
- Kwint M, Uyterlinde W, Nijkamp J, Chen C, de Bois J, Sonke J-J, et al. Acute esophagus toxicity in lung cancer patients after intensity modulated radiation therapy and concurrent chemotherapy. Int J Radiat Oncol Biol Phys 2012;84:e223–8.
- Palma Da, Senan S, Oberije C, Belderbos J, Dios NR, De, et al. Predicting Esophagitis After Chemoradiation Therapy for Non-Small Cell Lung Cancer: An Individual Patient Data Meta-analysis. Int J Radiat Oncol Biol Phys 2013;87:690–6.
- Uyterlinde W, Chen C, Kwint M, de Bois J, Vincent A, Sonke J-J, et al. Prognostic parameters for acute esophagus toxicity in intensity modulated radiotherapy and concurrent chemotherapy for locally advanced non-small cell lung cancer. Radiother Oncol 2013;107:392–7.
- Huang EX, Bradley JD, El Naqa I, Hope AJ, Lindsay PE, Bosch WR, et al. Modeling the risk of radiation-induced acute esophagitis for combined Washington University and RTOG trial 93-11 lung cancer patients. Int J Radiat Oncol Biol Phys 2012;82:1674–9.
- Singh A, Lockett M, Bradley J. Predictors of radiation-induced esophageal toxicity in patients with non-small-cell lung cancer treated with three-dimensional conformal radiotherapy. Int J Radiat Oncol 2003;55:337–41.
- Chapet O, Kong F-M, Lee JS, Hayman JA, Ten Haken RK. Normal tissue complication probability modeling for acute esophagitis in patients treated with conformal radiation therapy for non-small cell lung cancer. Radiother Oncol 2005;77:176–81.
- Lyman J. Complication probability as assessed from dose-volume histograms. Radiat Res 1985;19:13–19.
- Niemierko A. Reporting and analyzing dose distributions: a concept of equivalent uniform dose. Med Phys 1997;24:103–10
- Chapet O, Thomas E, Kessler ML, Fraass BA, Ten Haken RK. Esophagus sparing with IMRT in lung tumor irradiation: an EUD-based optimization technique. Int J Radiat Oncol Biol Phys 2005;63:179–87.
- Niedzielski JS, Bluett JB, Williamson RT, Liao Z, Gomez DR, Court LE. Analysis of esophageal-sparing treatment plans for patients with high-grade esophagitis. J Appl Clin Med Phys 2013;14:163–70.
- Dieleman EMT, Senan S, Vincent A, Lagerwaard FJ, Slotman BJ, van Sörnsen de Koste JR. Four-dimensional computed tomographic analysis of esophageal mobility during normal respiration. Int J Radiat Oncol Biol Phys 2007;67:775–80.
- Blom GJ, Verbakel Wfar Dahele M, Hoffmans D, Slotman BJ Senan S. Improving radiotherapy planning for large volume lung cancer: A dosimetric comparison between hybrid-IMRT and RapidArc. Acta Oncol (Madr) 2015;54:427–32.
- Verbakel Wfar van Reij E, Ladenius-Lischer I, Cuijpers JP, Slotman BJ Senan S. Clinical application of a novel hybrid intensity-modulated radiotherapy technique for stage III lung cancer and dosimetric comparison with four other techniques. Int J Radiat Oncol Biol Phys 2012;83:e297–303.
- Källman P, Ågren A, Brahme A. Tumour and normal tissue responses to fractionated non-uniform dose delivery. Int J Radiat Biol 1992;62:249–62.
- Werner-Wasik M, Yorke E, Deasy J, Nam J, Marks LB. Radiation dose-volume effects in the esophagus. Int J Radiat Oncol 2010;76:S86–93.
- Aerts HJ, van Baardwijk AA, Petit SF, Offermann C, Loon J, Van, et al. Identification of residual metabolic-active areas within individual NSCLC tumours using a pre-radiotherapy (18)Fluorodeoxyglucose-PET-CT scan. Radiother Oncol 2009;91:386–92.
- Guckenberger M, Richter A, Wilbert J, Flentje M, Partridge M. Adaptive radiotherapy for locally advanced non-small-cell lung cancer does not underdose the microscopic disease and has the potential to increase tumor control. Int J Radiat Oncol Biol Phys 2011;81:e275–82.