Dear Editor,
Stereotactic body radiotherapy (SBRT) is a guideline recommended treatment for peripherally located early-stage non-small cell lung cancer (NSCLC), where it has been associated with high rates of local control and a low risk of serious toxicity [Citation1]. However, the large fraction size and short overall treatment time are damaging for many normal tissues [Citation2,Citation3]. This has led to greater caution about applying it to centrally located lung tumors. These are closer to numerous organs at risk (OAR) including the trachea and proximal bronchial tree (PBT) and so the risks are higher, especially for very central tumors [Citation4–10]. To better understand the risks, we evaluated whether the high-dose region delivered to the PBT wall (PBT-Wall) reflected the planned dose distribution when treatment was performed with on-line cone-beam computed tomography (CBCT) and positioning based on the tumor.
Material and methods
We analyzed clinical data from seven patients with some of the most central tumors that we have treated with 8 fractions of 7.5 Gy (Supplementary Figure 1, available online at http://www.informahealthcare.com) [Citation10]. In brief, all treatment plans were generated using a free-breathing, uncoached, 10 phase four-dimensional (4D) CT scan with 2.5 mm slice thickness. The internal target volume (ITV) was delineated on the 4DCT average intensity projection dataset (Ave-IP) using the information from all 10 respiratory phases and the maximum intensity projection. The planning target volume (PTV) was created by a 5 mm isotropic expansion of the ITV. The treatment plan was created using volumetric modulated arc therapy (VMAT) RapidArc® (Varian Medical Systems), with dose calculation performed on the Ave-IP using a heterogeneity corrected algorithm (either Analytical Anisotropic Algorithm or Acuros® XB in the Eclipse™ treatment planning system, Varian Medical Systems). Our standard institutional approach to planning is to allow heterogenous dose in the PTV (maximum point dose up to 140% of the prescribed dose) and to normalize the plans such that the prescribed dose is delivered to 95% of the PTV. Treatment delivery is performed under free-breathing conditions without external immobilization. A CBCT scan is acquired (2.5 mm slice thickness) and matched to the reference Ave-IP dataset using an automated match based on the tumor region, represented by the PTV + 1 cm. Two technologists review the match in three planes and make manual adjustments as needed. Following this, the VMAT treatment is delivered (typically two arcs with avoidance sectors to spare the contralateral lung). If necessary (e.g. if a change in patient position is suspected or if the target is considered susceptible to positional change) the treating team can decide to acquire an additional CBCT scan between the VMAT arcs, or at the end of treatment in order to confirm target position/patient stability [Citation11,Citation12].
The lumen of the central airway was contoured by one observer from 1 cm proximal, to 1 cm below the cranio-caudal extent of the PTV on the Ave-IP dataset in Eclipse, using a combination of mediastinal and lung window level settings. All 4DCT datasets, contours, planned doses and CBCT data were then imported into the Velocity™ AI platform (Version 3.1.0, Varian Medical Systems). All CBCT scans were already DICOM linked to the Ave-IP planning CT in the on-line matched position. The airway lumen was rigidly transferred from the Ave-IP to each available CBCT scan in this position, and then manually edited using visually comparable mediastinal and lung window settings to those that had been used when contouring the airways lumen on the Ave-IP planning CT. The manually edited contours were reviewed by two people. All Ave-IP and CBCT airway lumens were then expanded radially by 2.5 mm to generate a contour of the airway wall (PBT-Wall).
The goal was to estimate the minimum dose (D) delivered to the hottest 0.25 cm3, 0.5 cm3 and 1 cm3 of the PBT-Wall for each fraction. We used the dose to the clinical, on-line, CBCT matched position of the airways as a surrogate for this and compared it with the planned dose. To do this, all contoured CBCT walls were transferred back to the Ave-IP dataset in the DICOM registered on-line match position. The dose-volume histograms (DVH) for each of the PBT-Wall structures (one associated with the planning CT Ave-IP and each of the CBCT scans) were then derived from the clinical treatment plan calculated on the Ave-IP. This represented the total planned dose for the entire 8-fraction treatment. VelocityAI allows a fractional DVH to be created, from which the planned and estimated delivered D0.25 cm3, D0.5 cm3 and D1 cm3 for all PBT-Wall structures could be derived for the original planned position, and for the position at each fraction.
These data provide information about the dose to the hottest parts of PBT-Wall, but do not indicate whether these volumes were located in the same part of the PBT-Wall. Therefore, the spatial locations of the planned and delivered PBT-Wall D0.5 cm3 were compared. To do this, the D0.5 cm3 isodoses for the PBT-Wall of the planning CT and each pretreatment CBCT were converted into a structure. The intersection of this structure with the respective PBT-Wall allowed the location of the hottest regions to be identified. For each patient all of the available PBT-Wall structures were then rigidly registered to each other on the Ave-IP and the displacement of the center of mass of each delivered D0.5 cm3 structure was compared with the center of mass of the planned D0.5 cm3 region, to give an indication of how similar their locations were.
Results
The tumor and treatment characteristics of the seven datasets in this analysis are summarized in Supplementary Figure 1 and Supplementary Table 1 (available online at http://www.informahealthcare.com). The motion of the tumor and the central airway structures were similar on the planning 4DCT, and within 1 mm of each other for each individual patient. and summarize the dosimetric data derived from all pretreatment, on-line CBCT matched positions for all seven patients. This represents a total of 55 CBCT scans. The mean difference for individual patients between the estimated delivered and the planned (pretreatment minus planned) D0.25 cm3, D0.5 cm3 and D1 cm3 was between −0.69 and +0.03 Gy/fraction. When considering all individual results, the absolute differences between estimated delivered and planned doses were within ±0.25 and ±0.50 Gy/fraction in 69% and 89% (D0.25 cm3, n = 55), 58% and 78% (D0.5 cm3, n = 55) and 51% and 80% (D1 cm3, n = 55) of all fractions, respectively. The absolute estimated delivered D0.25 cm3 for each individual fraction (n = 55 observations) exceeded the planned dose by no more than 0.29 Gy. In the difference in the x, y and z displacements of the center of mass between the planned and delivered (pretreatment) positions of the D0.5 cm3 regions for all patients are presented (n = 55 observations in each translational direction). In total 161/165 observations (98%) were within ±2.5 mm; 141/165 (85%) within ±2.0 mm and 131/165 (79%) within ±1.5 mm.
Figure 1. For all seven cases the difference between the proximal bronchial tree wall (PBT-Wall) dose based on the pretreatment CBCT and the planned fractional dose to the hottest 0.25, 0.5 and 1 cm3 of PBT-Wall is shown graphically.
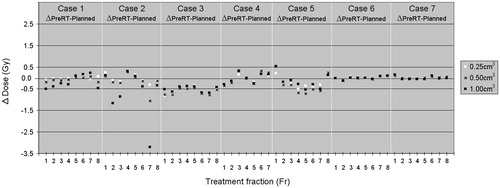
Figure 2. This illustrates the limited difference between the planned and delivered (pretreatment CBCT) position of the center of mass of the D0.5 cm3 PBT-Wall structure. All individual displacements in each translational direction have been combined (n = 165 individual x, y and z measurements combined) and 85% of differences were within ±2.0 mm.
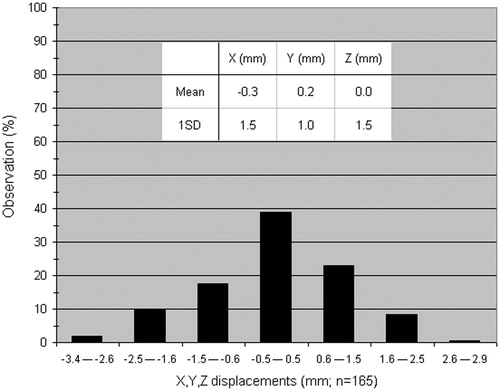
Table I. A summary of the differences between the estimated pretreatment dose and the planned dose for the “hottest” parts of the proximal bronchial tree wall (PBT-wall).
Discussion
A number of papers provide information about target-OAR distance and OAR dose [Citation9,Citation13,Citation14], however, we are not aware of other published data using on-line matched CBCT scans performed prior to treatment to estimate the dose delivered to the PBT during each fraction of central lung SBRT. Using this approach, we saw that in this cohort, there was generally good agreement between planned and estimated delivered doses. This was especially so for the hottest D0.25 and D0.5 cm3 regions, which are most closely related to the target volume and therefore least likely to deviate from the planned position after the on-line match.
Despite the close relationship between the target volume and OAR, we acknowledge that there is some uncertainty in the accuracy of the estimated doses. More accurate/reliable estimates will require additional data during the beam-on period combined with dose recalculation. This may be at least partly addressed by various tracking and imaging solutions designed for conventional treatment platforms as well as new platform designs that incorporate magnetic resonance imaging (MRI) [Citation15–17]. Although we did not have imaging during beam-on, for Case 1 there were six post-treatment CBCT scans available that had not been matched after acquisition. There was little difference in the D0.25 cm3 when subtracting the post-treatment PBT-Wall dose from the pretreatment PBT-Wall dose (mean 0.06 Gy; range of absolute difference for these 6 fractions 0.01–0.15 Gy), suggesting that the position of the hottest region before and after irradiation was relatively stable. The differences in the D0.5 and D1.0 cm3 were slightly larger with a mean of 0.17 and 0.37 Gy, and a range of 0.01–0.63 and 0.00–0.77 Gy, respectively. The use of a planning OAR volume (PRV) and more homogenous dose around the PBT-Wall merit investigation as strategies to reduce the probability of excessive dose to this structure.
In creating the PBT-Wall structures we used manually edited lumen contours instead of simply deforming the original Ave-IP contour over to the CBCT scans. We had intended to use deformable registration alone, but visual inspection of the deformed contours of the airway lumen suggested that it was not sufficiently accurate for this particular piece of work. In three patients, the mean maximum difference between the deformed contour and the manually derived contour was between 2.3 mm (SD 0.9 mm) and 2.5 mm (SD 1.7 mm).
In conclusion, this preliminary study of central lung tumors found generally good agreement between the estimated delivered and planned stereotactic fraction doses to the proximal airways.
Disclosure statement
The Department of Radiation Oncology VU University Medical Center has research collaborations with Varian Medical Systems. MD has received travel support and honoraria from Varian Medical Systems and Brainlab AG. WFV has received travel support and honoraria from Varian Medical Systems. BJS has received travel support and honoraria from Varian Medical Systems and Brainlab AG. SS has received travel support and honoraria from Varian Medical Systems.
Suppl_Table_1_Final.docx
Download MS Word (13 KB)Suppl_Figure_1_Final.tif
Download TIFF Image (4.1 MB)References
- Vansteenkiste J, Crinò L, Dooms C, Douillard JY, Faivre-Finn C, Lim E. Panel Members, et al. 2nd ESMO Consensus Conference on Lung Cancer: early-stage non-small-cell lung cancer consensus on diagnosis, treatment and follow-up. Ann Oncol 2014;25:1462–74.
- Timmerman RD, Herman J, Cho LC. Emergence of stereotactic body radiation therapy and its impact on current and future clinical practice. J Clin Oncol 2014;32:2847–54.
- Roach MC, Videtic GM Bradley JD, Iaslc Advanced Radiation Technology Committee. Treatment of Peripheral Non-Small Cell Lung Carcinoma with Stereotactic Body Radiation Therapy. J Thorac Oncol 2015;10:1261–7.
- Chang JY, Bezjak A Mornex F, Iaslc Advanced Radiation Technology Committee. Stereotactic ablative radiotherapy for centrally located early stage non-small-cell lung cancer: what we have learned. J Thorac Oncol 2015;10:577–85.
- Senthi S, Haasbeek CJ, Slotman BJ, Senan S. Outcomes of stereotactic ablative radiotherapy for central lung tumours: a systematic review. Radiother Oncol 2013;106:276–82. Erratum in: Radiother Oncol 2013; 109:183.
- Timmerman R, McGarry R, Yiannoutsos C, Papiez L, Tudor K, DeLuca J, et al. Excessive toxicity when treating central tumors in a phase II study of stereotactic body radiation therapy for medically inoperable early-stage lung cancer. J Clin Oncol 2006;24:4833–9.
- Dahele M, Tekatli H, Senan S. Stereotactic body radiotherapy for central lung tumours. Br J Radiol 2015;88:20150410.
- Nishimura S, Takeda A, Sanuki N, Ishikura S, Oku Y, Aoki Y, et al. Toxicities of organs at risk in the mediastinal and hilar regions following stereotactic body radiotherapy for centrally located lung tumors. J Thorac Oncol 2014;9:1370–6.
- Haseltine JM, Rimner A, Gelblum DY, Modh A, Rosenzweig KE, Jackson A, et al. Fatal complications after SBRT for central lung tumors abutting the proximal bronchial tree. Pract Radiat Oncol doi: 10.1016/j.prro.2015.09.012.
- Tekatli H, Senan S, Dahele M, Slotman BJ, Verbakel WF. Stereotactic ablative radiotherapy (SABR) for central lung tumors: Plan quality and long-term clinical outcomes. Radiother Oncol 2015;117:64–70.
- Dahele M, Verbakel W, Cuijpers J, Slotman B, Senan S. An analysis of patient positioning during stereotactic lung radiotherapy performed without rigid external immobilization. Radiother Oncol 2012;104:28–32.
- Peguret N, Dahele M, Cuijpers JP, Slotman BJ, Verbakel WF. Frameless high dose rate stereotactic lung radiotherapy: intrafraction tumor position and delivery time. Radiother Oncol 2013;107:419–22.
- Chaudhuri AA, Tang C, Binkley MS, Jin M, Wynne JF, von Eyben R, et al. Stereotactic ablative radiotherapy (SABR) for treatment of central and ultra-central lung tumors. Lung Cancer 2015;89:50–6.
- Chang JY, Li QQ, Xu QY, Allen PK, Rebueno N, Gomez DR, et al. Stereotactic ablative radiation therapy for centrally located early stage or isolated parenchymal recurrences of non-small cell lung cancer: how to fly in a “no fly zone”. Int J Radiat Oncol Biol Phys 2014;88: 1120–8.
- van Sörnsen de Koste JR, Dahele M, Mostafavi H, Sloutsky A, Senan S, Slotman BJ, et al. Markerless tracking of small lung tumors for stereotactic radiotherapy. Med Phys 2015;42:1640–52.
- Mutic S, Dempsey JF. The ViewRay system: magnetic resonance-guided and controlled radiotherapy. Semin Radiat Oncol 2014; 24:196–9.
- Lagendijk JJ, Raaymakers BW, van Vulpen M. The magnetic resonance imaging-linac system. Semin Radiat Oncol 2014;24:207–9.