Abstract
Introdution: Variation in shape, position and treatment response of both tumor and organs at risk are major challenges for accurate dose delivery in radiotherapy. Adaptive radiotherapy (ART) has been proposed to customize the treatment to these motion/response patterns of the individual patients, but increases workload and thereby challenges clinical implementation. This paper reviews strategies and workflows for clinical and in silico implemented ART for prostate, bladder, gynecological (gyne) and ano-rectal cancers. Material and methods: Initial identification of papers was based on searches in PubMed. For each tumor site, the identified papers were screened independently by two researches for selection of studies describing all processes of an ART workflow: treatment monitoring and evaluation, decision and execution of adaptations. Both brachytherapy and external beam studies were eligible for review. Results: The review consisted of 43 clinical studies and 51 in silico studies. For prostate, 1219 patients were treated with offline re-planning, mainly to adapt prostate motion relative to bony anatomy. For gyne 1155 patients were treated with online brachytherapy re-planning while 25 ano-rectal cancer patients were treated with offline re-planning, all to account for tumor regression detected by magnetic resonance imaging (MRI)/computed tomography (CT). For bladder and gyne, 161 and 64 patients, respectively, were treated with library-based online plan selection to account for target volume and shape variations. The studies reported sparing of rectum (prostate and bladder cancer), bladder (ano-rectal cancer) and bowel cavity (gyne and bladder cancer) as compared to non-ART. Conclusion: Implementations of ART were dominated by offline re-planning and online brachytherapy re-planning strategies, although recently online plan selection workflows have increased with the availability of cone-beam CT. Advantageous dosimetric and outcome patterns using ART was documented by the studies of this review. Despite this, clinical implementations were scarce due to challenges in target/organ re-contouring and suboptimal patient selection in the ART workflows.
Organ motion with changes in shape, size and position is one of the major challenges for accurate dose delivery in radiotherapy (RT). The most common approach to compensate for this motion in clinical practice has been to extend the target with population-based margins. For tubular and hollow clinical target volumes (CTV), e.g. rectum, bladder, uterus and cervix, margins well above 1 cm have been suggested to maintain target coverage in the population [Citation1,Citation2]. Moreover, treatment response may result in changes in the functional properties of tissues and/or regression of the target volume, which can cause the planned dose distribution to be sub-optimal for the remaining treatment fractions [Citation3,Citation4]. As a further complication, both the geometrical motion patterns and the treatment response can vary greatly between patients [Citation4–8].
Adaptive radiotherapy (ART) was proposed by Yan et al. as an alternative to tailor margin and dose to the individual patient [Citation9]. In ART, images recorded in connection to treatment fractions are systematically used to determine patient-specific anatomical variations for which the treatment plan can be modified [Citation9,Citation10]. Independent of the clinical intentions, the ART workflow described by Yan constitutes four sub-processes; “feedback of dose from delivered fractions as compared to the planned dose distribution (dose assessment)”, “detection and analysis of variations” (commonly by use of repeat imaging), “decision whether to adapt the plan”, and “execution of the modified treatment plan” [Citation9,Citation10]. As the fractionated treatment progresses, new measurements can continuously be used to verify and re-adapt the treatment plan [Citation9,Citation10]. In this paper we present a literature review of clinical and in silico implemented adaptive strategies and workflows in the context of these four sub-processes. In particular, we considered prostate, gynecological, bladder, and ano-rectal tumors. In line with Yan et al., an ART workflow was defined as a process where an adaptive intervention was used as a systematic strategy on the basis of images acquired on- or offline. The adaptive intervention was required to involve more than one treatment plan (including calculated dose distribution), e.g. plan selection from a plan library, re-optimization/re-planning or multileaf-collimator (MLC)/treatment field alterations. Both external beam radiotherapy (EBRT) and brachytherapy (BT) publications were eligible. Papers describing systematic plan adaptations based on functional/biological imaging were also allowed, as an extension of ART to account for variations in organ motion.
Material and methods
A protocol specifying review questions and definitions of the study objective, participants, interventions, as well as outcome measures was composed prior to the start of the searches to avoid bias in the review.
Paper selection
Systematic searches in PubMed were the basis for identification of papers to review. The searches included the following mesh terms applied to titles and abstracts of full length papers: “(adapt* AND (radiotherapy OR radiation therapy OR radiation treatment))”, and one of the site-specific keywords: “prostate” for prostate cancer, “bladder” for bladder cancer, “cervi*”, “gyn*”, “ovar*”, “vagi*” and “vulv*” for gynecological cancers, or “rect*” and “anal” for ano-rectal tumors. The searches were restricted to English written papers. For each site, the search and the following paper selection (below) was performed independently by two researchers. The last Pubmed search update for all sites was conducted on January 26, 2015.
For each tumor site, papers identified in the Pubmed searches were first screened on title and abstract, in order to exclude studies lacking an ART workflow (as defined in the Introduction section), e.g. no application of RT, reports on target definitions, outcome and prediction analysis, or comparison of different RT delivery techniques. For the remaining papers, a full paper screening was performed to verify that the topic was indeed an ART workflow as defined above. Disagreements in paper selection between the two researchers evaluating each tumor site were discussed before final selection. If needed, an additional senior researcher was consulted. Finally, papers referred to by the selected studies were screened as well using the review inclusion and exclusion criteria.
Data extraction
For all selected papers, data from each sub-process in the ART workflow [Citation10] was collected. This data included the type and frequency of imaging used for identification of treatment variations, the type of decision criteria used to trigger adaptations, any dose prescription modifications, if the adaptations were performed online or offline, as well as the type of modification executed (e.g. plan selection or re-planning/re-optimization). For the clinical papers, additional data on the number of patients treated with the ART protocol, the clinical intentions behind the trials, the recorded results/gain, and any reports on additional workload and/or quality assurance measures for ART were also gathered.
Results
Eligible studies
The results of the literature search and screening process for all sites are shown in . The Pubmed searches resulted in 897 hits. Seventy-four of these papers passed both the title and abstract screening and the full paper screening, i.e. they contained a description of a full adaptive workflow either in a clinical (33 papers), or in silico (41 papers) implementation. These studies referred to additional 10 clinical and 10 simulation papers that were added to the review. In total, the review included 43 clinical studies and 51 in silico studies ().
Table 1. Results from the PubMed searches and paper selection.
Study characteristics
Clinical implementations of ART were found to increase around year 2004 for all but ano-rectal cancers (). For prostate cancer, a relatively low number of ART workflows had reached clinical implementations since the year 2005, in comparison to the vast number of in silico studies that had been published. The opposite trend was seen for gynecological cancers, where published clinical implementations outnumbered the in silico studies. This was largely due to the imbalance between BT and EBRT, with EBRT contributing with only one study to the clinical implementations but dominating the silico studies. For both bladder and ano-rectal cancers, the number of clinical and in silico implementations were more balanced (, ). Of the 43 publications involving clinically implemented ART, three were multi-center studies and the others single institutional studies, conducted at 24 different clinics in 12 countries ( and ).
Figure 1. Accumulation of clinical implementation and simulation studies for prostate (dark gray), gynecological (light gray), bladder (gray) and ano-rectal (black) cancer. Date of enrollment of the first patient were denoted for the clinical implementations (solid lines) and date of acceptance for publication of the simulation studies (dotted lines).
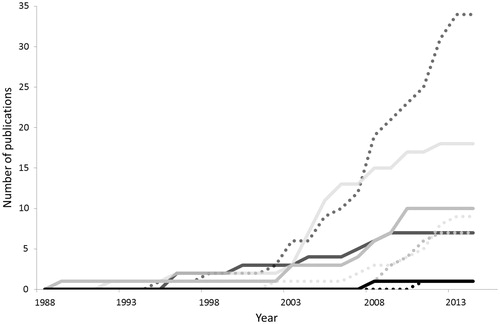
Table 2. Applied plan adaptations and reported dosimetric gains in the clinically implemented studies for external beam radiotherapy.
Table 3. Applied plan adaptations and reported dosimetric gains for adaptation in the clinically implemented studies for a combination of brachy- and external beam radiotherapy.
For the published clinical implementations of ART, 36 different workflows had been introduced, almost half of which were applied to the combined treatment of EBRT and BT for gynecological cancers ( and ). These workflows could be grouped into different strategies based on timing and type of plan adaptations: 1) on- or offline re-planning/re-optimization; 2) online plan selection; and 3) on- or offline MLC/field adjustments ( and ). Due to the rapid increase in the number of clinical implementations in BT since 2005 (), the majority of the patients included in this review had been treated with online re-planning/re-optimization ( and , see Gynecological cancer – brachytherapy). For EBRT only, most patients had been treated with offline re-planning/re-optimization ( and ), largely related to early implementations for prostate cancer. The new plan in EBRT was presented as a geometric correction of target motion for 98% of the patients while for 2% it was a biological adaptation to tumor regression magnetic resonance imaging (MRI) (see Prostate cancer, Bladder cancer, Ano-rectal cancers). For online re-planning/re-optimization with BT, tumor regression from MRI was used for >58% of the patients. However, no implementations were found using functional properties as feedback to ART in this review. For the online library-based plan selection strategy in EBRT, 71% of the patients had been treated for bladder cancer, 28% patients had been treated for cervical cancer and only one patient had been treated for prostate cancer. For all but the prostate cancer patient, the library of plans had been constructed from detection of variations in bladder filling. Details of the published clinically implemented ART workflows have been summarized in specific sections for each site along with and , Supplementary Tables S1 and S2 (available online at http://www.informahealthcare.com), and and .
Figure 2. The number of prostate (dark gray), gynecological (light gray), bladder (gray) and ano-rectal (black) patients treated with the different categories of ART workflows. Patients treated with brachytherapy additionally marked with striped pattern.
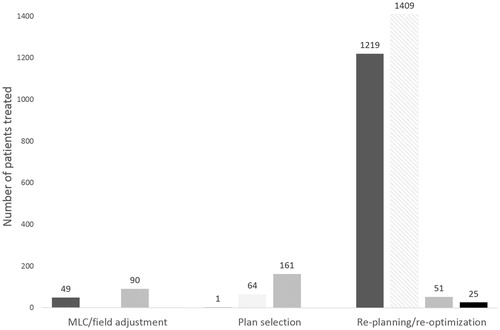
Figure 3. Different categories of the implemented and the simulated ART workflows: online re-planning (blue), offline re-planning (red), online plan selection (turquoise), online MLC/field alteration (green), offline MLC/field alteration (violet). The workflows are plotted as percentage of the total number of either implementation or simulation workflows. Studies concerning brachytherapy additionally marked with striped pattern. clin: clinical; sim: simulation; gyne: gynecological.
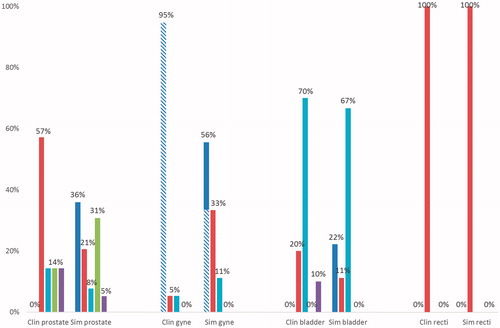
Comparisons between ART and conventional practice – an overview
Comparison of ART to the conventional practice (e.g. population-based margins and image-guided patient positioning) was available in a larger fraction of the published implementations for EBRT, 63% than for BT, 18% of the reviewed workflows ( and ). Comparisons with the conventional method was more frequently included the in silico studies with 83% presenting such data. However, only 5% of the in silico studies involved BT. Overall, the evaluations had large variations both in regard to included number of patients: ranging from 1 to 201, as well as the number of treatment fractions: ranging from two to a complete course of treatment having daily cone-beam computed tomography (CBCT). The results for prostate EBRT showed a greater gain from adaptations (positive values in ) in minimum dose to the CTV, dose to 95% and 99% of the prostate when compared to set-up based on bony anatomy than when compared to set-up on gold markers. The overall largest improvement from ART of the prostate (dose to 95% of the prostate) was from online MLC-adaptations, though the results were influenced by the target margins applied with others ambiguously reporting decreased coverage from this ART strategy. Two of the in silico studies applying online MLC-adaptation also reported an increase in bladder dose as compared to gold marker guided set-up (). In general, for ART in prostate cancer, sparing of the rectum was reported in all but two ART studies and rectum sparing was generally greater than bladder sparing (). For online plan selection, when applied to gynecological and bladder cancers, improved coverage but the greatest impact of these adaptations were sparing of the bowel and normal tissue for doses higher than 45 Gy in addition to reduced irradiation of the rectum (). Sparing of the bladder and bowel were benefits of offline re-planning adaptations for ano-rectal cancers ().
Figure 4. Changes in evaluation points with ART as compared to the conventional strategy for prostate (dark gray diamonds), gynecological (light gray triangles), bladder (gray circles) and ano-rectal (black squares). Changes in target coverage is displayed in top left (A), to the rectum top right (B), bladder lower left (C) and the bowel cavity and other normal tissues lower right (D). Studies concerning brachytherapy additionally marked with striped pattern. BA: bony anatomy; GM: gold markers; BT: brachytherapy; fxs: fractions.
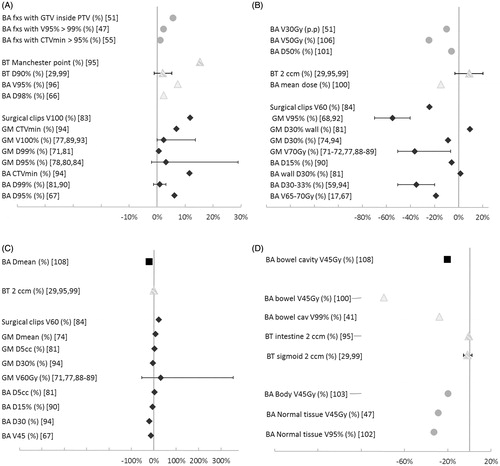
For BT, a large improvement in target coverage from ART as compared to the conventional/old method (the Manchester point) was reported (). The improved coverage with online re-planning increased the dose to rectum whereas smaller changes were reported for the bladder ().
Prostate cancer
ART had been used clinically for prostate cancer since 1997, with a total of 1269 patients reported as treated at six different institutions [Citation11,Citation12,Citation13–21]. For the reviewed studies reporting CTV extension, the volume included prostate only in 405 patients, prostate and parts of the seminal vesicles in 649 patients and prostate, seminal vesicles and pelvic lymph nodes in one patient. ART for prostate had been clinically implemented with all three strategies of adaptation (MLC/field adjustments, re-plan/re-optimization and plan selection), through seven different clinical workflows (, ). Only three of these workflows enrolled patients after year 2010 ().
Offline field adjustments or re-plan to correct for prostate motion relative to bony anatomy
The adaptation strategy and timing applied to the majority (1186) of the prostate patients was offline re-plan/re-optimization after the initial 1–2 weeks of treatment to adjust to patient-specific prostate motion relative to bony anatomy (, and ) [Citation11–18]. This ART strategy was used in three single institutional studies. The earliest implementations, and the pioneers of ART for prostate cancer, were conducted at Beaumont Hospital, US, where 962 patients were treated between the years 1997–2006 [Citation9–16,Citation22]. The target treated was prostate only for 42% of the patients with the remaining 58% receiving irradiation of both prostate and seminal vesicles. Their initial implementation, applied to 10 patients, was based on field adjustments for adaptation to the patient-specific set-up errors detected by mega-voltage (MV) radiographs [Citation13]. Subsequently, the workflow was expanded to adaptation of target shape changes through construction of a patient-specific planning target volume (PTV) and finally – for the majority of the patients – this PTV was used for individualized dose escalations until reaching the pre-set criteria for rectum and bladder constraints () [Citation11–16,Citation22]. The patient-specific PTV, adapted target motion in regard to bony anatomy and was composed of a union formed from four delineated target volumes in the initial CTs to account for shape – and positional changes. The union was expanded with a patient-specific PTV margin calculated set-up errors depicted with radiographs (Table S1) [Citation11–12,Citation22]. The new conformal RT (CRT) or intensity modulated RT (IMRT) plan was made offline using the patient-specific PTV and used for the remainder of the treatment course [Citation13,Citation14,Citation22].
Offline re-plan with a patient-specific PTV correcting prostate motion in treatments with set-up on bony anatomy was also used at NKI-AVL, Amsterdam in 2005 as well as at Pardubice hospital, Pardubice in 2008–2012 [Citation17,Citation18]. At NKI-AVL, the majority of the 23 patients (75%) had irradiation of the prostate and seminal vesicles, the remaining prostate only [Citation17] whereas seminal vesicle irradiation was not stated for the Pardubice patients [Citation18]. As the Beaumont group, the group at NKI-AVL accounted for target position and shape variations but detected with CBCT of the first week of treatment. The average target and rectum were calculated from variations of the corresponding points of the prostate and rectum shapes in the repeat CBCTs and the prostate and rectum of the planning CT, after bony anatomy based co-registrations [Citation17]. As the NKI-AVL group aimed for toxicity reduction of the rectum, the new IMRT plan was optimized using the prescription of the initial plan. The group allowed for re-activation of the initial plan (with larger margins) if indicated by the weekly CBCT used for verification of the treatment. This occurred in 4% of the follow-up CBCTs [Citation17]. The group at Pardubice, corrected positional variations of the target in relation to the bony anatomy, analyzed from CBCTs of the initial 10 fractions [Citation18]. Patient-specific PTV margins were selected based on the standard deviation of the position of the prostate in regard to bony anatomy while the average position was applied as a shift of the isocenter. These adaptations were use when optimizing the new IMRT plan used for the remaining fraction [Citation18].
Outcome and/or toxicity data has only been reported by the Beaumont group, with 96% bio-chemical control after five years for the low-risk (prostate only) and 83% for the higher risk groups [Citation16]. Acute rectal toxicity ≥ Grade 2 [Common Terminology Criteria (CTC) 2.0] was 34%, presented for a sub-group of 331 patients [Citation15]. Late rectal toxicity after five-year follow-up was 21% for CTC Grade ≥2 and 3% for CTC Grade ≥3. Late effect for the bladder was reported for 16% as CTC Grade ≥2 and for 4% as CTC Grade ≥3 [Citation16].
Comparisons of ART to conventional margins showed that a larger dose escalation could be achieved through ART [Citation11]. For the NKI-AVL patients, the adapted PTV was on average 29% smaller than the initial PTV leading to sparing of the rectum (, ).
Offline re-plan and online MLC adjustments to adapt to intra-fractional motion
Two studies used intra-prostatic gold markers in the prostate to adapt to intra-fractional motion but with substantially different workflows: one using online MLC adjustments and the other offline re-planning. The latter was applied in the study from Toronto-Sunnybrook Regional Cancer Center, Toronto for irradiation of the prostate and parts of the seminal vesicles [Citation19]. ART was employed for 33 patients to shrink margins in order to deliver a hypo-fractionated prostate boost. Intra-fractional motion of the gold markers was detected with kilovoltage (kV) radiographs collected pre- and post- each of the initial 9 fractions, in addition to respiratory motion detected with fluoroscopic imaging in connection to the planning CT. With the gathered data the random errors were quantified in order to calculate individualized PTVs, used for offline planning of the hypo-fractionated IMRT boost. The boost was delivered in the last 10 fractions with gold marker-based set-up. No dosimetric data or comparisons with non-ART were presented. Toxicity reports were limited to the bladder with an incidence of acute CTC Grade 3 of 9% [Citation19]. The other study reporting ART for intra-fractional motion was conducted in 2009 in Salzburg [Citation20]. As for the Toronto patients, the prostate and parts of the seminal vesicles were treated. MV and kV radiographs detected the location of intra-prostatic gold markers and rigidly aligned these to the markers in the planning CT. The obtained transformation was used to online re-position the MLC segments of the original IMRT plan (monitor units unchanged). The adapted fractions were verified with MV radiographs during delivery and re-adaptation of segments was triggered if a marker translation >3 mm was detected. The verification MV images revealed the adapted PTV to account for target motion in the majority of the patients, but some patients needed a PTV enlargement (). Comparison to non-ART was made for a selected patient, showing a considerable PTV reduction from online ART () [Citation20]. No outcome or toxicity data was reported.
Plan selection for adaptation of uncorrelated prostate and lymph node motion
A case study from University of San Francisco and the Cleveland Clinic in 2009 used online plan selection for a high-risk (HR) prostate cancer patient with nodal involvement in the proximity of an abdominal kidney [Citation21]. The switch to ART in the last 17 of the 25 fractions was triggered by observed acute rectal complications. The plan library consisted of five IMRT plans generated by shifts of the prostate contour (0.5–1.0 cm) in posterior-superior direction relative to the lymph node target in the planning CT. The plan-of-the-day was selected from the position of the implanted gold markers relative to the bony anatomy, as quantified by daily pretreatment MV-CBCTs. The adaptations resulted in reduced prostate but increased lymph node coverage as compared to non-ART as well as kidney sparing () [Citation21].
Gynecological cancer
For gynecological tumor sites ART implementations had been used since 1993 for treatment of in total of 1409 patients at 10 institutions and one multi-center study () [Citation23–41]. All but four studies focused on cervical cancer, most commonly staged as FIGO IIB. One study focused on locally advanced vaginal cancer with patients mainly in FIGO stages II and III, and one on confined endometrial cancers mainly with FIGO stage IB. Two studies investigated recurrent endometrial or gynecological cancer. The majority of the studies dealt with BT alone or in combination with EBRT. The majority of the reported ART studies used workflows based on the Gynecological GEC-ESTRO guidelines published in 2005 () [Citation42,Citation43]. Only two studies reported on ART implementations for EBRT of cervical cancer.
Gynecological cancer – brachytherapy
Early implementations of online brachytherapy re-planning
ART for gynecological BT was first implemented at the Vienna General Hospital, Vienna, with the motivation to detect incorrect applicator placements and thus improve target coverage and/or organs at risk (OAR) sparing. Online applicator adaptations were performed for 181 cervix cancer patients based on visual evaluation of the applicator placement, with the BT dose distributions superimposed on CTs [Citation23] (). Gradually, the Vienna group applied similar ART strategies for locally advanced cervix cancer, vaginal cancer, as well as endometrial cancer, while replacing the CT images with MRI and moving from 2D- to 3D-based treatment planning () [Citation24]. Starting in 2001, MRI-based ART using re-defined gross tumor volume (GTV), OARs as well as a HR-CTV for re-planning of every BT fraction was applied systematically for cervical cancer treatment () [Citation26,Citation27]. The new HR-CTV considered tumor regression occurring between the MR images at time of diagnosis, and the MR images of each BT fraction [Citation26]. The re-defined structures were used for online re-planning with an individual dose escalation limited by OAR constraints [Citation26,Citation27]. With the introduction of MRI-based planning interstitial needles were available to compliment intracavitary BT for coverage of large tumors [Citation24,Citation26]. Later, in the years 2005–2007, the updated Vienna workflow was implemented at Addenbrooke’s Hospital in Cambridge, UK, treating 28 locally advanced cervical patients [Citation28]. Due to the inaccessibility of MRI, this group used CTs, which led to a slight change of target definitions as compared to the Vienna workflow.
The results from the earliest implementation in Vienna revealed a wrongly placed applicator in 7% of the instances where a CT was acquired, and dose adaptation was performed in 30% of the treatments, mainly to modify the rectal dose [Citation23]. With approximately three-year follow-up, pelvic control of the cervical cancer was achieved in 78% of the patients, and rates for LENT SOMA Grade ≥3 toxicity were 10% for gastro-intestinal (GI), 3% for bladder, and 31% for the vagina [Citation23]. In comparison, three-year pelvic control for the 156 cervix patients treated with the updated workflow from 2001 was improved to 91%, and LENT SOMA Grade ≥3 toxicity was reduced to 4% for GI, 2% for the bladder and 1% for the vagina [Citation27]. For these patients, the average dose to 90% (D90) of the HR-CTV was 93 Gy and the local control at three years was 95%. For patients with endometrial cancer, the ART workflow was feasible but only 19% of the patients received CTV D90 ≥ the prescribed dose of 60 Gy [Citation24]. However, 92% of the patients with curative intent and who completed treatment had local control with no reports of toxicity LENT SOMA (GI or GU) >Grade 2 [Citation24]. For locally advanced vaginal cancer, the average D90 of the HR-CTV was 86 Gy with three-year actuarial local control of 92% and no late side effects higher than LENT SOMA Grade 2 [Citation25]. For the cervical cancer patients treated at Addenbrooke’s Hospital, 86% had coverage of HR-CTV D90 of a dose equal to or higher than the prescribed (74 Gy EQD2), the deviations caused by OAR constraints. With an average follow-up of 23 months, both the pelvic control and the toxicity was higher than that reported by the Vienna group with 96% control and 11% RTOG Grade ≥3 toxicity (abdominal pains and fistula) [Citation28].
Online re-plan using the GEC-ESTRO recommendations for adaptation to target response
The recommendations from the gynecological GEC-ESTRO working group, published in 2005, served to unify the BT community through a set of target definitions and reporting concepts facilitating implementation of image-based BT. Relating this to an ART workflow, the GEC-ESTRO recommended imaging at the time of BT for assessment of residual tumor after response to EBRT. Subsequently three target volumes were adapted to a differential risk: GTV seen at time of BT, a HR-CTV and an intermediate-risk CTV (IR-CTV) obtained by adding a margin determined by the amount of regression of the HR-CTV. The BT was adapted through online re-planning prescribing different dose levels to each adapted risk volume and allowing an individual dose escalation bounded by the recommended OAR doses [Citation42–44]. Twelve studies have so far reported their experience and outcomes after clinical implementation according to these recommendations () [Citation29–40], 10 of which focused solely on cervical cancer [Citation29–31,Citation34–40]. The majority (674/871) of the cervical cancer patients underwent EBRT combined with 1–3 fractions of pulsed-dose-rate (PDR) BT, 151 patients received EBRT and high-dose-rate (HDR) BT and 46 patients EBRT in addition to a combination of PDR and HDR () [Citation29–31,Citation34–40]. Across these studies, the aim was to deliver at least 80 Gy to the HR-CTV and/or at least 60 Gy to the IR-CTV. Most patients were treated with MR-guided BT, except for those included in the French multi-institutional study where the limited availability of MRI caused the regression analysis and adaptations to be based on CTs [Citation37]. In two studies MR images were only used in the first BT fraction and CTs used for the remaining fractions [Citation35,Citation39]. In addition to the adaptations recommended by the Gynecological GEC-ESTRO, a group at Aarhus University Hospital, Aarhus, sculptured a supplementary stereotactic boost to the BT dose distribution. In their workflow, a CT was acquired for planning of the stereotactic dose distribution additional to the MR images at each BT fraction [Citation40]. Following intracavitary BT complimented by interstitial needles, the IMRT boost was planned offline with the applicator still in place. This workflow was applied to three patients with either large tumors or challenging anatomy for delivery of a high dose (>70 Gy) to the entire HR-CTV plus 3 mm margin, with a greater contribution of the dose from the boost to the distal regions of the target. The boost was delivered at the linear accelerator, following CBCT-guided set-up based on applicator position. The workflow greatly improved dose coverage of the HR-CTV and with 1–3 years of follow-up, none of the patients had recurrences and only one patient experienced CTC Grade 3 toxicity (pelvic pain) [Citation40].
The studies of cervical treatment following the gynecological GEC-ESTRO guidelines reported the planned D90 for both the HR- and IR-CTV in 42% while D90 for only HR-CTV was provided in 33% of the studies. The average D90 to the cervical HR-CTV was 80 Gy (range: 67; 92 Gy) while D90 of IR-CTV was 64 Gy (range: 59; 68 Gy). Actuarial local control at 2–3 years in the studies ranged from 88% to 95%. Actuarial toxicity scored as Grade 3 or worse for any normal tissue ranged from 0% to 9% at 2 or 3 years. In comparison to the standard technique (point A) Charra-Brunaud et al. and Lindegaard et al. both reported superior outcome and toxicity profiles through the adaptations in the GEC-ESTRO guidelines. In the French multi-center study reported by Charra-Brunaud et al. the increase in local relapse-free survival at two years from the adaptations as compared to the standard BT varied between the risk groups. For the IR group the local relapse-free survival increased from 85% to 93% whereas the highest risk group had a smaller increase, from 74% to 79% [Citation37]. Toxicity reported as CTC Grade ≥3 was decreased from 13% to 9% and from 23% to 3% for the IR and HR group, respectively [Citation37]. Lindegaard et al. reported actuarial pelvic control at three years being increased from 76% to 85%, while toxicity RTOG Grade ≥3 decreased from 10% to 3% with adaptive BT as compared to standard BT [Citation29].
Extrapolating the GEC-ESTRO guidelines to other gynecological cancers, the Aarhus group applied the adaptations in BT to 28 patients with advanced vaginal, endometrial or cervix cancers treated with laparoscopic guided PDR BT as well as 43 recurrent endometrial patients treated with intracavitary BT [Citation32,Citation33]. Both studies used a prescription dose of 80 Gy and 60 Gy to the HR- and IR-CTV, respectively. All patients in these studies completed treatment and both studies reported an actuarial local control at two years of 92% with no acute CTC Grade 3 toxicity. GI complications were the most common morbidity, with a higher risk for Grade 3 complication in the latter study with patients treated for recurrent endometrial cancer [Citation32,Citation33].
Gynecological cancer – EBRT
Online plan selection adapting for shape and positional changes of the cervix-uterus
An ART workflow based on daily plan selection from a plan library to accommodate for variations in position and shape of cervix-uterus had been applied to 64 cervical cancer patients since 2001 at the Erasmus Medical Center, Rotterdam [Citation41]. All patients were treated in prone position with a belly-board and had markers implanted in the lower part of cervix for the purpose of image guidance. The plan libraries consisting of motion-adapted IMRT plans and a 3DCRT back-up plan were generated prior to the fractionated treatment. For planning, individualized cervix-uterus internal target volumes (ITVs) were produced from patient-specific motion models using the correlation in bladder filling and cervix-uterus shape. This correlation was derived with deformable registration of the delineated target in two planning CTs, with an empty and a full bladder [Citation7]. Initially, the individualized libraries contained one IMRT plan, based on an ITV capturing cervix-uterus shapes for all bladder fillings but later patients exhibiting large cervix motion (pre-set criterion) had two IMRT plans, each covering a subset of possible bladder fillings, in their library. All patients had markers implanted in the lower part of the cervix, to aid the daily plan selection based on CBCT. Implementations were successful, with the back-up plan used in less than 20% of the fractions. In the later implementations, 11 of 29 patients were selected as needing two ITVs to account for their extensive cervix-uterus motion. For those patients dosimetric comparisons showed ART to reduce high dose to bowel cavity [Citation41] (, ).
Bladder cancer
ART was introduced for bladder cancer already in 1989 and so far 302 patients have been enrolled in clinical ART from seven different institutions and three multi-center studies [Citation45,Citation56]. The majority of the patients had been treated with a uniform dose to the whole bladder [Citation45–53], while 46 patients had received a boost of the bladder tumor [Citation52–56] and nine had received partial bladder treatment [Citation52,Citation56]. In total 36 patients had the pelvic lymph nodes included the treatment [Citation51,Citation55,Citation56]. Three different ART strategies had been used in the ART implementations ( and ).
Offline field adjustments and re-plan for varying bladder shape and position
Offline field adjustments were used in the two earliest studies. The first study was conducted in 1989–1990 at Yorkshire Regional Center for Cancer Treatments, Leeds including 90 patients while the second study was conducted in 1993 at Christie Hospital, Manchester with 30 patients (, S1) [Citation45,Citation46]. Both studies used CTs to detect deviations and to decide upon adaptations. From visually comparing the bladder shape mid-treatment and the treated volume, Sur et al. found 20% of the patients with a change in bladder shape, and 40% of these patients subsequently had the treatment fields of the CRT plan adjusted. No difference in outcome between the adapted and the non-adapted patients was noted [Citation45]. Turner et al. applied an adaptation criterion for the bladder wall at mid-plane extending beyond the 95%-isodose in the weekly CTs. This triggered a new CRT plan in 7% of the patients [Citation46].
One of the implementations for bladder cancer used offline re-planning based on an ITV derived from the CTs acquired in the first week of treatment [Citation56]. Similar to their workflow for prostate cancer (see section Prostate cancer), 21 patients were treated in a joined feasibility study from the Academic Medical Center, University of Amsterdam and NKI-AVL Hospital, Amsterdam in 2003–2004 [Citation56]. The adapted 3DCRT plan used an ITV accounting for target motion relative to the bony anatomy by forming a union of all partial bladder GTV contours delineated in the daily CTs. The new plan was used from the third week, continuously evaluated by repeat CTs and allowing re-activation of the initial plan in case of sub-optimal coverage in two successive CTs [Citation56]. The authors reported a smaller PTV but improved target coverage with ART as compared to the initial plan (, ).
Online plan selection based on a single or multiple plan CTs
The remaining studies on plan adaptations in bladder cancer used online plan selection (, ). At Tata Memorial Hospital, Mumbai in 2008, several PTVs were generated by expanding the CTV in the planning CT by isotropic margins of 5–30 mm in 5 mm steps, yielding a library consisting of six IMRT plans. The plans of the library were generated either with a simultaneous integrated boost to the suspicious tumor area or with homogenous irradiation of the bladder. The plan-of-the-day was selected after registering the bony anatomy and bladder (manually) of daily MVCTs to the planning CT. In the post-treatment MVCT scans used to verify the plan selection, the authors observed a substantial risk of geographical miss due to intra-fractional bladder filling but no dosimetric evaluation was provided [Citation53].
The most common approach for plan library creation in bladder cancer was to use 2–5 planning CTs with varying bladder fillings, which was employed at Helsinki University Central Hospital, Helsinki, Royal Marsden Hospital, Sutton and Catharina Hospital Eindhoven, Eindhoven [Citation52,Citation54,Citation55,Citation59]. The enrolled patients received a uniform bladder dose with either conventional or hypo-fractionated regimen, simultaneous integrated tumor dose or a hypo-fractionated partial bladder treatment. Two planning CTs, one with full and one with empty bladder were acquired for library creation in the studies from the Royal Marsden and Eindhoven whereas the group in Helsinki used 4–5 successive planning CTs acquired over 15–30 minute intervals. The number of plans, 3DCRT [Citation49], IMRT [Citation54,Citation55] or volumetric modulated arc therapy (VMAT) [Citation52], in the libraries varied from three to six as did the motion models to create the different ITVs. The groups in Helsinki and Sutton both created 3–4 ITVs by expanding the bladder volumes seen in the multiple CTs by 5–20 mm whereas the group at Eindhoven applied a deformable motion model to derive the ITVs. The motion model used vector fields obtained from deformable registration of bladder contours and GTV of the empty- and full bladder CTs, after bony anatomy-based alignment. The vector fields were inter-and extrapolated to create six bladder ITVs with associated GTVs. In all studies, plan selection guided by daily CBCTs started from the first fraction. The plan-of-the-day was chosen such that the PTV covered the visible bladder, often with a margin for intra-fractional bladder filling. This selection was aided by rigidly aligning the daily CBCTs to the planning CT in addition to Lipiodol markers for patients receiving partial bladder- or integrated boost to the GTV [Citation52,Citation54]. The results from target coverage evaluation in 25 patients with post-treatment CBCT, revealed the 95%-isodose encompassing the CTV in 88% of the treatment fractions [Citation49]. ART lead to PTV reduction or sparing of normal tissue as compared to conventional delivery () [Citation49,Citation55]. Acute toxicity was reported by Meijer et al. with no patient having CTCAE Grade ≥3.
Online plan selection based multiple plan CBCTs
A third alternative for building a plan library was to create multiple ITVs from the bladders observed in daily CBCTs acquired in the first 4–5 fractions of treatment. This method had been employed in one single- and two multi-institutional studies in Australia/New Zeeland and Denmark [Citation47,Citation48,Citation50,Citation51]. The first implementation was a pilot study involving 27 patients in 2008–2009 at Peter MacCallum Cancer Center, Melbourne. The study was later expanded to a multi-institutional trial involving 12 RT centers in Australia and New Zealand in 2010–2013 [Citation47,Citation48,Citation50]. The Danish multicentre trial enrolled patients during 2012–2013 [Citation51]. All these studies used manual segmentation of the bladder CTV in the repeat CBCTs to generate adapted ITVs. The Melbourne group defined three ITVs: 1) small as the CTV with least filling or the union of the two smallest CTVs; 2) large as the union of all CTVs; and 3) medium as the mean of the large and small CTVs. 3DCRT plans were generated for all these ITVs. The group in Denmark defined the three ITVs slightly different, with 1) small as the union of at least two bladder CTVs; 2) large as the ITV of the initial plan; and 3) medium as the union of all bladders in the CBCTs. For each of these ITVs, a VMAT plan was optimized. Plan selection started in the second week of treatment and was based on daily CBCTs in all studies. The PTV best suited for the daily bladder volume was selected after aligning the isocenter or bony anatomy of the CBCTs to the planning CT. The reported comparisons to a non-adapted treatment revealed ART to greatly spare normal tissue (, ) [Citation47,Citation48,Citation51]. Acute toxicity incidence of CTCAE Grade ≥3 was reported for 15% and 18% of the patients in the Australian single- and multi-institutional studies, respectively [Citation47,Citation50].
Ano-rectal cancers
Offline re-plan/re-optimization to adapt to tumor shrinkage
The only clinical implementation found for rectal cancer used offline plan re-optimization for adaptation to treatment response. The study from San Raffaele Scientific Institute, Milan included 25 patients treated in 2009–2012. The treatment was delivered in two phases; an initial phase of hypo-fractionation to the CTV plus margin in 12 fractions followed by the adapted plan with an additional integrated boost to the adapted GTV for the final 6 fractions. The adapted GTV was defined on a mid-therapy MRI (ninth fraction) and transferred through bony anatomy registration to the mid-therapy planning CT for PTV creation and optimization of the new tomotherapy plan. Throughout the treatment, set-up was based on bony anatomy with manual adjustments to correct for rotations and deformations. The study reported ART to enable dose escalation (). Result of tumor response in regard to surgical findings was promising with 7 patients showing no residual viable cells and 12 patients having less than 10% residual viable cells after RT. Only acute complication scoring was available with three patients reported CTCAE Grade 3 toxicity [Citation57].
Discussion
This systematic review of adaptive strategies for pelvic tumor sites included treatment of in total of 3069 patients from clinical ART implementations in 12 countries. In addition to these studies, 51 studies in silico implementations of a full ART workflow were found. The clinically and in silico implemented workflows reflects the tumor sites well, where the faster responding tumors of cervix and rectum were adapted based on treatment response whereas tumors of the bladder and prostate have been adapted to variations in motion. The clinical implementations of ART were dominated by three strategies and timing of ART workflows: online re-planning/re-optimization applied to 1409 patients all receiving BT, offline re-planning/re-optimization applied to 1358 patients and online plan selection applied to 226 patients (). This pattern was also seen in the in silico workflows where most studies used online re-planning/re-optimization followed by offline re-planning/re-optimization but plan selection was equally common to online MLC/beam alterations (). However, online re-planning/re-optimization presented by the in silico studies was dominated by EBRT studies whereas in silico studies applied to BT were rare ().
Mature clinical outcome on recurrence and toxicity was reported by the gynecological cancers treated with a combination of EBRT and BT [Citation23,Citation25–30,Citation32–39] as well as from the large prostate trial conducted at Beaumont [Citation12,Citation14–16]. For the gynecological cancers and most pronounced for the advanced stages, the improved target coverage obtained through ART has improved local control [Citation37]. In addition, a decrease in complications has been reported by patients receiving BT with ART in comparison with the old method of treatment delivery [Citation27,Citation29,Citation37,Citation38]. These encouraging results from following the Gynecological GEC-ESTRO recommendations [Citation42,Citation43] has also led to patients previously appointed to hysterectomy now being considered for BT [Citation30]. Regarding the Beaumont trial, outcome was favorable or similar to the large non-ART trials using similar prescription delivered with 3DCRT [Citation16,Citation108,Citation109,Citation110] or IMRT [Citation16,Citation111,Citation110] but toxicity was generally higher in the Beaumont trial [Citation16,Citation111,Citation110]. Non-ART with gold marker-based set-up has indicated further improvement in toxicity to be possible [Citation112,Citation113]. For the more recent implementations of adaptations in prostate as well as bladder cancer, longer follow-up is needed to assess the potential clinical gain of ART. Until such outcome data has matured, comparison of ART to the conventional RT with population-based margins can be used to assess the dosimetric benefit of ART. Such data was mainly provided by in silico studies. The comparisons revealed the target coverage to improve with ART for the vast majority of the studies, but the potential for OAR sparing was the most evident benefit from the applied ART workflows ().
The comparisons of ART with a non-adaptive treatment were strongly dependant on differences in size of the applied margins. In several studies, ART allowed for margin reduction but both clinical as well as in silico studies also reported decreased coverage (, ) [Citation21,Citation49,Citation50,Citation78,Citation90]. Prolonged fraction times could reduce the benefits of ART, requiring larger margins for intra-fractional motion. This could be a concern for plan selection strategies based on differential bladder fillings [Citation49,Citation50]. Here, the different ITVs of the library were commonly expanded with identical PTV margins to account for both intra-fractional and delineation uncertainties. The additional time needed for plan selection based on CBCTs in bladder cancer was reported to 2–8 min (excluding CBCT uptake [Citation44,Citation54,Citation55]). This corresponded well to that using non-adaptive image guidance [Citation49,Citation55]. Reasons for the rare occasion when plan selection was unfeasible, besides intra-fractional motion, were to poor CBCT image quality and technical malfunction of the CBCT [Citation44,Citation50,Citation55]. To mitigate the limited CBCT image quality for plan selection in EBRT of cervical cancer, Heijkoop et al. used cervical markers while in plan selection for bladder cancer, Tuomikoski et al. and Meijer et al. used Lipiodol injections to visualize bladder tumor. Further improvements of the plan selection process could also be attained through feedback and training of the staff responsible for the selections [Citation47,Citation50].
Another factor that influence the results form ART alone or when compared to the conventional practice, is the patients used for comparison. ART is still a resource intensive correction strategy and patients with the most benefit from the corrections should be prioritized. However, the majority of the studies in this review adapted the treatment for all patients with only about 17% of the clinically applied ART and 18% of the in silico studies followed a workflow allowing for selective use of ART. Examples of patient selection in the reviewed workflows were, applied alone or in combination: exerting a threshold criteria on the detected motion for initiation of modifications [Citation20,Citation45,Citation46,Citation61–64,Citation69,Citation72] or shrinking the PTV margins to less than the common 90% of the population and correct for dose degradation of outliers during/at the end of treatment [Citation63,Citation65,Citation66,Citation94]. As an alternative, differentiating the size of the plan library based on a criterion for large and small cervix-uterus [Citation41] or discriminating the adaptations based on greater and smaller response to treatment as in the gynecological GEC-ESTRO recommendations has been applied clinically [Citation42].
For paper selection in the review, we used as inclusion criteria the application of ART as a systematic procedure to modify the applied (and calculated) dose distribution based on findings in imaging data. This led to inclusion of EBRT implementations from the year 1989 and BT studies starting in 1993 [Citation23,Citation45], even before the ART concept was introduced [Citation9]. The inclusion criteria of an updated dose distribution to select studies in this review lead to exclusion of papers solely focusing on geometric interventions, such as tumor set-up correction strategies etc. The pioneering paper in plan selection of bladder cancer from Burridge et al. was consequently excluded [Citation114]. Adaptation of the treatment plan could have benefited BT studies, since BT has a long tradition of involving both physicists and oncologists simultaneously in the more interventional treatment process as compared to EBRT. The effort in composing common and systematic guidelines involving diagnostic quality imaging (MRI) was likely to have facilitated introduction of online BT ART further, reflected by the rapid increase of clinical implementations ().
The motivation for including in silico studies in the review was to detect new strategies soon to be implemented as well as identifying possible bottlenecks hindering clinical implementation. Notable differences in the applied ART strategies between in silico and clinical application were obtained. Whereas online re-planning for prostate, gynecological and bladder cancer EBRT was applied in silico, the strategy was only used clinically for gynecological cancer treated with BT () [Citation58,Citation59,Citation61,Citation65–67,Citation70–72,Citation74,Citation78,Citation80,Citation82,Citation83,Citation88–91,Citation94,Citation100, Citation101,Citation103]. An ART workflow presented in the in silico studies but not in the clinical workflows was offline re-plan/re-optimization, applied during or at the end of EBRT, to correct degradation of the dose distribution from previously delivered fraction [Citation60,Citation63,Citation69,Citation75,Citation76]. In addition to one in silico implementation of online compensation [Citation58], these were the only studies where feedback from delivered dose triggered adaptations. Contributing to differences in applied strategies and workflows could be the additional manual contouring, which was a pre-requisite in over 70% of the simulated ART workflows [Citation22,Citation59,Citation60,Citation62–66,Citation68–70,Citation72,Citation74,Citation78,Citation79,Citation81–83,Citation85,Citation86,Citation88,Citation89,Citation91,Citation93,Citation95–98,Citation100–107]. To reduce the need of manual contours, the segmentation could be propagated using deformable image registration. Deformable registration was utilized when generating ITVs in one offline re-planning/re-optimization and in two online plan selection workflows applied clinically [Citation17,Citation41,Citation54]. Deformable image registration was more frequently applied in the in silico studies, mostly for online re-planning/re-optimization [Citation59,Citation61,Citation67,Citation71,Citation86,Citation87,Citation94] and offline re-planning/re-optimization strategies [Citation60,Citation63,Citation66,Citation69,Citation75,Citation107] but also applied for online MLC/field adjustments [Citation77,Citation89]. To facilitate adaptations, especially for online execution, modifications of the planning system or automatic activation/selection of plans was applied in ∼50% of the in silico studies [Citation58–61,Citation67–69,Citation71,Citation72,Citation74–80,Citation82–88,Citation92–94]. In view of the increasing speed of optimization algorithms, limited in-room imaging quality together with manual (re-)contouring remains the biggest bottlenecks for clinical implementation of many of the ART strategies and certainly limiting online applications.
The number of images or inputs needed for adaptations of the treatment is a tradeoff between an accurate prediction, cost, imaging dose and the benefit of the adjustments. The majority of the clinical implementations and almost 40% of the in silico studies with an offline re-planning strategy adapted to motion using images from the initial 4–9 fractions (Table S1). Images acquired in the first week of treatment were also used for creating plan libraries in bladder cancer. Another method was to construct the library from multiple planning CTs, which besides bladder was applied to account for cervix-uterus motion. The former strategy has been applied to 94 patients and the latter to 114 patients, with the library reported as a poor model for only four patients, respectively [Citation44,Citation50,Citation51,Citation54,Citation55]. Consequently, although the approach of creating a plan library based on multiple planning CTs and initiating plan selection at first fraction might be more time efficient, the first week of images also seem to provide a good representation of the motion in later stages.
In conclusion, clinical implementations of ART were dominated by offline re-planning/re-optimization and online BT re-planning/re-optimization, although recently online plan selection workflows have increased with the availability of CBCT. Advantageous dosimetric and outcome-related patterns has been documented by the studies on ART included in this review. Despite this, clinical implementations have been scarce, especially regarding prostate and the vast amount of in silico studies available. Identified challenges, hindering successful clinical implementations, were re-contouring of target/OARs in addition to patient selection in the workflows to guide the resources towards the most challenging patients.
SONC-2015-0809.R1_-_Supplementary_material_revised.docx
Download MS Word (27.1 KB)Acknowledgments
The author would like to acknowledge Acta Oncologica for initiating this review. This first author of this work has been supported by research grants from the Norwegian Cancer Society.
Disclosure statement
The authors report no conflict of interest.
References
- Meijer G, Rasch C, Remeijer P, Lebesque J. Three-dimensional analysis of delineation errors, setup errors, and organ motion during radiotherapy of bladder cancer. Int J Radiat Oncol Biol Phys 2003;55:1277–87.
- Van de Bunt L, Jürgenliemk-Schulz I, de Kort G, Roesink J, Tersteeg R, van der Heide UA. Motion and deformation of the target volumes during IMRT for cervical cancer: what margins do we need? Radiother Oncol 2008;88:233–40.
- Schmid MP, Mansmann B, Fredrico M, Dimopoulous JC, Georg P, Fidarova E, et al. Residual tumour volumes and grey zones after external beam radiotherapy (with or without chemotherapy) in cervical cancer patients. A low-field MRI study. Strahlenther Onkol 2013;189:238–44.
- Lim K, Chan P, Dinniwell R, Fyles A, Haider M, Cho YB, et al. Cervical cancer regression measured using weekly magnetic resonance imaging during fractionated radiotherapy: radiobiologic modelling and correlation with tumor hypoxia. Int J Radiat Oncol Biol Phys 2008;70:126–33.
- Zahra MA, Tan LT, Priest AN, Graves MJ, Arends M, Crawford RAF. Semiquantitative and quantitative dynamic contrast-enhanced magnetic resonance imaging measurements predict radiation response in cervix cancer. Int J Radiat Oncol Biol Phys 2009;74:766–73.
- Thörnqvist S, Hysing LB, Zolnay AG, Söhn M, Hoogeman MS, Muren LP, et al. Treatment simulations with a statistical deformable motion model to evaluate margins for multiple targets in radiotherapy for high-risk prostate cancer. Radiother Oncol 2013;109:344–9.
- Bondar ML, Hoogeman MS, Mens JW, Quint S, Ahmad R, Dhawtal G, et al. Individualized nonadaptive and online-adaptive intensity-modulated radiotherapy treatment strategies for cervical cancer patients based on pretreatment acquired variable bladder filling computed tomography scans. Int J Radiat Oncol Biol Phys 2011;83:1617–23.
- Muren LP, Smaaland R, Dahl O. Organ motion, set-up variation and treatment margins in radical radiotherapy of urinary bladder cancer. Radiother Oncol 2003;69:297–304.
- Yan D, Vicini F, Wong J, Martinez A. Adaptive radiation therapy. Phys Med Biol 1997;42:123–32.
- Yan D. Adaptive radiotherapy: merging principle into clinical practice. Semin Radiat Oncol 2010;20:79–83.
- Martinez A, Yan D, Lockman D, Brabbins D, Kota K, Sharpe M, et al. Improvement in dose escalation using the process of adaptive radiotherapy combined with three-dimensional conformal or intensity-modulated beams for prostate cancer. Int J Radiat Oncol Biol Phys 2001;50:1226–34.
- Brabbins D, Martinez A, Yan D, Lockman D, Wallace M, Gustafson G, et al. A dose-escalation trial with the adaptive radiotherapy process as a delivery system in localized prostate cancer: analysis of chronic toxicity. Int J Radiat Oncol Biol Phys 2005;61:400–8.
- Yan D, Ziaja E, Jaffray D, Wong J, Brabbins D, Vicini F, et al. The use of adaptive radiation therapy to reduce setup error: a prospective clinical study. Int J Radiat Oncol Biol Phys 1998;41:715–20.
- Vargas C, Yan D, Kestin L, Krauss D, Lockman D, Brabbins D, et al. Phase II dose escalation study of image-guided adaptive radiotherapy for prostate cancer: use of dose-volume constraints to achieve rectal isotoxicity. Int J Radiat Oncol Biol Phys 2005;63:141–9.
- Vargas C, Martinez A, Kestin L, Yan D, Grills I, Brabbins D, et al. Dose-volume analysis of predictors for chronic rectal toxicity after treatment of prostate cancer with adaptive image-guided radiotherapy. Int J Radiat Oncol Biol Phys 2005;62:1297–308.
- Park S, Yan D, McGrath S, Dilworth J, Liang J, Ye H, et al. Adaptive image-guided radiotherapy (IGRT) eliminates the risk of biochemical failure caused by the bias of rectal distension in prostate cancer treatment planning: clinical evidence. Int J Radiat Oncol Biol Phys 2012;83:947–52.
- Nijkmap J, Pos F, Nuver T, de Jong R, Remeijer P, Sonke J-J, et al. Adaptive radiotherapy for prostate cancer using kilovoltage cone-beam computed tomography: first clinical results. Int J Radiat Oncol Biol Phys 2008;70:75–82.
- Vanasek J, Odrazka K, Dolezel M, Dusek L, Jarkovsky J, Hlavka A, et al. Searching for an appropriate image-guided radiotherapy method in prostate cancer-implications for safety margin. Tumori 2014;100:518–23.
- Cheung P, Sixel K, Morton G, Loblaw A, Tirona R, Pang G, et al. Individualized planning target volumes for intrafraction motion during hypofractionated intensity-modulated radiotherapy boost for prostate cancer. Int J Radiat Oncol Biol Phys 2005;62:418–25.
- Deutschmann H, Kametriser G, Steininger P, Scherer P, Schöller H, Gaisberger C, et al. First clinical release of an online, adaptive, aperture-based image-guided radiotherapy strategy in intensity-modulated radiotherapy to correct for inter- and intrafractional rotations of the prostate. Int J Radiat Oncol Biol Phys 2012;83:1624–32.
- Xia P, Qi P, Hwang A, Kinsey E, Pouliot J, Roach M. Comparison of three strategies in management of independent movement of the prostate and pelvic lymph nodes. Med Phys 2010;37:5006–13.
- Yan D, Lockman D, Brabbins D, Tyburski L, Martinez A. An off-line strategy for constructing a patient-specific planning target volume in adaptive treatment process for prostate cancer. Int J Radiat Oncol Biol Phys 2000;48:289–302.
- Pötter R, Knocke RH, Fellner C, Baldass M, Reinthaller A, Kucera H. Definitive radiotherapy with iridium 192 in uterine cervix carcinoma: report on the Vienna University Hospital findings (1193-1997) compared to the preceding period in the context of ICRU 38 recommendations. Cancer Radiother 2000;4:159–72.
- Weitman J, Pötter R, Waldhäusl C, Nechvile E, Kirisits C, Knocke T. Pilot study in the treatment of endometrial carcinoma with 3D image-based high-dose brachytherapy using modified Heyman packing: clinical experience and dose-volume histogram analysis. Int J Radiat Oncol Biol Phys 2005;62:468–78.
- Dimopoulos J, Schmid M, Fidarova E, Berger D, Kirisits C, Pötter R. Treatment of locally advanced vaginal cancer with radiochemotherapy and magnetic resonance image-guided adaptive brachytherapy: dose-volume parameters and first clinical results. Int J Radiat Oncol Biol Phys 2012;82:1180–8.
- Pötter R, Dimopoulos J, Georg P, Lang S, Waldhäusl C, Wachter-Gerstner N, et al. Clinical impact of MRI assisted dose volume adaptation and dose escalation in brachytherapy of locally advanced cervix cancer. Radiother Oncol 2007;83:14–155.
- Pötter R, Georg P, Dimopoulos J, Grimm M, Berger D, Nesvacil N, et al. Clinical outcome of protocol based image (MRI) guided adaptive brachytherapy combined with 3D conformal radiotherapy with or without chemotherapy in patients with locally advanced cervical cancer. Radiother Oncol 2011;100:116–23.
- Tan L, Coles C, Hart C, Tait E. Clinical impact of computed tomography-based image-guided brachytherapy for cervix cancer using the tandem-ring applicator - the Addenbrooke's experience. Clin Oncol (R Coll Radiol)2009;21:175–82.
- Lindegaard J, Fokdal L, Kynde S, Juul-Christensen J, Tanderup K. MRI-guided adaptive radiotherapy in locally advanced cervical cancer from a Nordic perspective. Acta Oncol 2013;53:1510–9.
- Mazeron R, Glimore J, Dumas I, Champoudry J, Goulart J, Vanneste B, et al. Adaptive 3D image-guided brachytherapy: a strong argument in the debate on systematic radical hysterectomy for locally advanced cervical cancer. Oncologist 2013;18:15–22.
- Morgia M, Cuartero J, Walsh L, Lezioranski J, Keeler K, Xie J, et al. Tumor and normal tissue dosimetry changes during MR-guided pulsed-dose-rate (PDR) brachytherapy for cervical cancer. Radiother Oncol 2013;107:46–51.
- Fokdal L, Tanderup K, Kynde Nielsen S, Kidmose Christensen H, Røhl L, Morre Pedersen E, et al. Image and laparoscopic guided interstitial brachytherapy for locally advanced primary or recurrent gynaecological cancer using the adaptive GEC-ESTRO target concept. Radiother Oncol 2011;100:473–9.
- Fokdal L, Ørtoft G, Hansen ES, Røhl L, Pedersen EM, Tanderup K, et al. Toward four-dimensional image-guided adaptive brachytherapy in locally recurrent endometrial cancer. Brachytherapy 2014;13:554–61.
- Nomden C, de Leeuw A, Roesink J, Tersteeg R, Moerland M, Witteveen P, et al. Clinical outcome and dosimetric parameters of chemo-radiation including MRI guided adaptive brachytherapy with tandem-ovoid applicators for cervical cancer patients: a single institution experience. Radiother Oncol 2013;107:69–74.
- Mahantshetty U, Swamidas J, Khanna N, Engineer R, Merchant N, Deshpande D, et al. Reporting and validation of gynaecological groupe euopeen de curietherapie European society for therapeutic radiology and oncology (ESTRO) brachytherapy recommendations for MR image-based dose volume parameters and clinical outcome with high dose-rate brachytherapy in cervical cancer. Clin Oncol 2011;21:1110–6.
- Chargari C, Magné N, Dumas I, Messai T, Vicenzi L, Gillion N, et al. Physics contribution and clinical outcome with 3D-MRI-based pulsed-dose-rate intracavitary brachytherapy in cervical cancer patients. Int J Radiat Oncol Biol Phys 2009;74:133–9.
- Charra-Brunaud C, Harter V, Delannes M, Haie-Meder C, Quetin P, Kerr C, et al. Impact of 3D image-based PDR brachytherapy on outcome of patients treated for cervix carcinoma in France: results of the French STIC prospective study. Radiother Oncol 2012;103:305–13.
- Rijkmans EC, Nout RA, Rutten IH, Ketelaars M, Neelis KJ, Laman MS, et al. Improved survival of patients with cervical cancer treated with image-guided brachytherapy compared with conventional brachytherapy. Gynecol Oncol 2014;135:231–8.
- Beriwal S, Kannan N, Kim H, Houser C, Mogus R, Sukumvanich P, et al. Three-dimensional high dose rate intracavitary image-guided brachytherapy for the treatment of cervical cancer using a hybrid magnetic resonance imaging/computed tomography approach: feasibility and early results. Clin Oncol 2011;23:685–90.
- Assenholt MS, Vestergaard A, Kallehauge JF, Mohanmed S, Nielsen SK, Petersen JB, et al. Proof of principle: applicator-guided stereotactic IMRT boost in combination with 3D MRI-based brachytherapy in locally advanced cervical cancer. Brachytherapy 2014;13:361–8.
- Heijkoop ST, Langerak TR, Quint S, Bondar L, Mens J, Heijmen BJ, et al. Clinical implementation of an online adaptive plan-of-the-day protocol for nonrigid motion management in locally advanced cervical cancer IMR. Int J Radiat Oncol Biol Phys 2014;90:673–9.
- Haie-Meder C, Pötter R, van Limbergen E, Briot E, de Brabandere M, Dimopoulos J, et al. Recommendations from Gynaecological (GYN) GEC ESTRO Working Group (I): concepts and terms in 3D image-based 3D treatment planning in cervix cancer brachytherapy with emphasis on MRI assessment of GTV and CTV. Radiother Oncol 2005;74:235–45.
- Pötter R, Haie-Meder C, van Limbergen E, Barillot I, de Brabandere M, Dimopoulos J, et al. Recommendations from gynaecological (GYN) GEC ESTRO working group (II): Concepts and terms in 3D image-based treatment planning in cervix cancer brachytherapy-3D dose volume parameters and aspects of 3D image-based anatomy, radiation physics, radiobiology. Radiother Oncol 2006;78:67–77.
- International Commission on Radiation Units and Measurements (ICRU) Report 38. Dose and volume specification for reporting intracavitary brachytherapy in gynecology. 1985
- Sur R, Clinkard J, Jones W, Taylor R, Close H, Chaturvedi A, et al. Changes in target volume during radiotherapy treatment of invasive bladder carcinoma. Clin Oncol 1993;5:30–3.
- Turner S, Swindell R, Bowl N, Marrs J, Brookes B, Read G, et al. Bladder movement during radiation therapy for bladder cancer: implications for treatment planning. Int J Radiat Oncol Biol Phys 1997;39:355–60.
- Foroudi F, Wong J, Kron T, Rolfo A, Haworth A, Roxby P, et al. Online adaptive radiotherapy for muscle-invasive bladder cancer: Results of a pilot study. Int J Radiat Oncol Biol Phys 2011;81:765–71.
- Kron T, Wong J, Rolfo A, Pham D, Cramb J, Foroudi F. Adaptive radiotherapy for bladder cancer reduces integral dose despite daily volumetric imaging. Radiother Oncol 2010;97:485–7.
- McDonald F, Lalondrelle S, Taylor H, Warren-Oseni K, Khoo V, McNair HA, et al. Clinical implementation of adaptive hypofractionated bladder radiotherapy for improvement in normal tissue irradiation. Clin Oncol (R Coll Radiol) 2013;25:549–56.
- Foroudi F, Pham D, Rolfo A, Bressel M, Tang CI, Tan A, et al. The outcome of a multi-centre feasibility study of online adaptive radiotherapy for muscle-invasive bladder cancer TROG 10.01 BOLART. Radiother Oncol 2014;111:316–20.
- Vestergaard A, Muren LP, Lindberg H, Jakobsen KL, Petersen JB, Elstrøm UV, et al. Normal tissue sparing in a phase II trial on daily adaptive plan selection in radiotherapy for urinary bladder cancer. Acta Oncol 2014;53:997–1004.
- Tuomikoski L, Korhonen J, Collan J, Keyriläinen J, Visapää H, Sairanen J, et al. Implementation of adaptive radiation therapy for urinary bladder carcinoma: imaging, planning and image guidance. Acta Oncol 2013;52:1451–7.
- Murthy V, Master Z, Adurkar P, Mallick I, Mahantshetty U, Bakshi G, et al. 'Plan of the day' adaptive radiotherapy for bladder cancer using helical tomotherapy. Radiother Oncol 2011;99:55–60.
- Meijer GJ, van der Toorn PP, Bal M, Schuring D, Weterings J, de Wildt M. High precision bladder cancer irradiation by integrating a library planning procedure of 6 prospectively generated SIB IMRT plans with image guidance using lipiodol markers. Radiother Oncol 2012;105:174–9.
- Tuomikoski L, Collan J, Keyriläinen J, Visapää H, Saarilahti K, Tenhunen M. Adaptive radiotherapy in muscle invasive urinary bladder cancer–an effective method to reduce the irradiated bowel volume. Radiother Oncol 2011;99:61–6.
- Pos FJ, Hulshof M, Lebesque J, Lotz H, van Tienhoven G, Moonen L, et al. Adaptive radiotherapy for invasive bladder cancer: a feasibility study. Int J Oncol Biol Phys 2006;64:862–8.
- Passoni P, Fiorino C, Slim N, Ronzoni M, Ricci V, di Palo S, et al. Feasibility of an adaptive strategy in preoperative radiochemotherapy for rectal cancer with image-guided tomotherapy: boosting the dose to the shrinking tumor. Int J Radiat Oncol Biol Phys 2013;87:67–72.
- Lu W, Chen M, Ruchala KJ, Chen Q, Langen KM, Kupelian PA, et al. Real-time motion-adaptive-optimization (MAO) in TomoTherapy. Phys Med Biol 2009;54:4373–98.
- Wu QJ, Thongphiew D, Wang Z, Mathayomchan B, Chankong V, Yoo S, et al. On-line re-optimization of prostate IMRT plans for adaptive radiation therapy. Phys Med Biol 2008;53:673–91.
- Wu Q, Liang J, Yan D. Application of dose compensation in image-guided radiotherapy of prostate cancer. Phys Med Biol 2006;51:1405–19.
- Li T, Zhu X, Thongphiew D, Lee WR, Vujaskovic Z, Wu Q, et al. On-line adaptive radiation therapy: feasibility and clinical study. J Oncol 2010;2010:407236.
- Qi P, Pouliot J, Roach M 3rd, Xia P. Offline multiple adaptive planning strategy for concurrent irradiation of the prostate and pelvic lymph nodes. Med Phys 2014;41:021704–1-7.
- Lim K, Stewart J, Kelly V, Xie J, Brock KK, Moseley J, et al. Dosimetrically triggered adaptive intensity modulated radiation therapy for cervical cancer. Int J Radiat Oncol Biol Phys 2014;90:147–54.
- Lalondrelle S, Huddart R, Warren-Oseni K, Nordmark Hansen V, McNair H, Thomas K, et al. Adaptive-predictive organ localization using cone-beam computed tomography for improved accuracy in external beam radiotherapy for bladder cancer. Int J Oncol Biol Phys 2011;79:705–12.
- Oh S, Stewart J, Moseley J, Kelly V, Lim K, Xie J, et al. Hybrid adaptive radiotherapy with on-line MRI in cervix cancer IMRT. Radiother Oncol 2014;110:323–8.
- Stewart J, Lim K, Kelly V, Vie J, Brock K, Moseley J, et al. Automated weekly replanning for intensity-modulated radiotherapy of cervix cancer. Int J Radiat Oncol Biol Phys 2010;78:350–8.
- Yang C, Liu F, Ahunbay E, Chang Y-E, Lawton C, Schultz C, et al. Combined online and offline adaptive radiation therapy: a dosimetric feasibility study. Pract Radiat Oncol 2014;4:e75–83.
- Holubyev K, Bratengeier K, Gainey M, Polat B, Flentje M. Towards automated on-line adaptation of 2-step IMRT plans: QUASIMODO phantom and prostate cancer cases. Radiat Oncol 2013;8:263
- Liu H, Wu Q. Evaluation of an adaptive planning technique incorporating dose feedback in image-guided radiotherapy of prostate cancer. Med Phys 2011;28:6362–70.
- Battista JJ, Johnson C, Turnbull D, Kempe J, Bzdusek K, Van Dyk J, et al. Dosimetric and radiobiological consequences of computed tomography-guided adaptive strategies for intensity modulated radiation therapy of the prostate. Int J Radiat Oncol Biol Phys 2013;87:874–80.
- Li X, Quan EM, Li Y, Pan X, Zhou Y, Wang X, et al. A fully automated method for CT-on-rails-guided online adaptive planning for prostate cancer intensity modulated radiation therapy. Int J Radiat Oncol Biol Phys 2013;86:835–41.
- Li T, Thongphiew D, Zhu X, Lee WR, Vujaskovic Z, Yin FF, et al. Adaptive prostate IGRT combining online re-optimization and re-positioning: a feasibility study. Phys Med Biol 2011;56:1243–58.
- Yan D, Wong J, Michalski J, Pan C, Frazier A, Horwitz E, et al. Adaptive modification of treatment planning to minimize the deleterious effects of treatment set-up errors. Int J Radiat Oncol Biol Phys 1997;38:197–206.
- Boggula R, Lorenz F, Abo-Madyan Y, Lohr F, Wolff D, Boda-Heggemann J, et al. A new strategy for online adaptive prostate radiotherapy based on cone-beam CT. Z Med Phys 2009;19:264–76.
- Birkner M, Yan D, Alber M, Liang J, Nüsslin F. Adapting inverse planning to patient and organ geometrical variation: algorithm and implementation. Med Phys 2003;30:2822–31.
- Rehbinder H, Forsgren C, Löf J. Adaptive radiation therapy for compensation of errors in patient setup and treatment delivery. Med Phys 2004;31:22633371.
- Court L, Dong L, Lee A, Cheung R, Bonnen M, O'Daniel J, et al. An automatic CT-guided adaptive radiation therapy technique by online modification of multileaf collimator leaf positions for prostate cancer. Int J Radiat Oncol Biol Phys 2005;62:154–63.
- Ahunbay EE, Peng C, Holmes S, Godley A, Lawton C, Li XA. Online adaptive replanning method for prostate radiotherapy. Int J Radiat Oncol Biol Phys 2010;77:1561–72.
- Liu H, Wu Q. Dosimetric and geometric evaluation of a hybrid strategy of offline adaptive planning and online image guidance for prostate cancer radiotherapy. Phys Med Biol 2011;56:5045–62.
- Hwang AB, Chen J, Nguyen TB, Gottschalk AG, Roach MR, Pouliot J. Irradiation of the prostate and pelvic lymph nodes with an adaptive algorithm. Med Phys 2013;39:1119–24.
- Haverkort MA, van de Kamer JB, Pieters BR, Koning CC, van Herk M, van Tienhoven G. Adaptive margin radiotherapy for patients with prostate carcinoma: what's the benefit?. Radiother Oncol 2012;105:203–6.
- Li T, Wu Q, Zhang Y, Vergalasova I, Lee R, Fang-Fang L, et al. Strategies for automatic online treatment plan reoptimization using clinical treatment planning system: a planning parameters study. Med Phys 2013;40:1–14.
- Liu F, Ahunbay E, Lawton C, Li A. Assessment and management of interfractional variations in daily diagnostic-quality-CT guided prostate-bed irradiation after prostatectomy. Med Phys 2014;41:031710–1-14.
- Crijns W, Van Herck H, Defraence G, Van den Bergh L, Slagmolen P, Haustermans K, et al. Dosimetric adaptive IMRT driven by fiducial points. Med Phys 2014;41:061716–1-11.
- Stanley K, Eade T, Kneebone A, Booth JT. Investigation of an adaptive treatment regime for prostate radiation therapy. Pract Radiat Oncol 2015;5:e23–9.
- Song W, Wong E, Bauman S, Battisata J, van Dyk J. Dosimetric evaluation of daily rigid and nonrigid geometric correction strategies during on-line image-guided radiation therapy (IGRT) of prostate cancer. Med Phys 2007;34:352–65.
- Feng Y, Castro-Pareja C, Shekhar R, Yu C. Direct aperture deformation: an interfraction image guidance strategy. Med Phys 2006;33:4490–8.
- Ahnubay E, Peng C, Chen G-P, Yu C, Lawton C, Li A. An on-line replanning scheme for interfractional variations. Med Phys 2008;35:3607–15.
- Mohan R, Zhang X, Wang H, Kang Y, Wang X, Liu H, et al. Use of deformed intensity distributions for on-line modification of image-guided IMRT to account for interfractional anatomic changes. Int J Radiat Oncol Biol Phys 2005;61:1258–66.
- Beldjoudi G, Yartsev S, Bauman G, Battista J, van Dyk J. Schedule for CT image guidance in treating prostate cancer with helical tomotherapy. Br J Radiol 2010;83:241–51.
- Schulze D, Liang J, Yan D, Zhang T. Comparison of various online IGRT strategies: The benefit of online treatment plan re-optimisation. Radiother Oncol 2009;90:367–76.
- Bratengeier K, Polat B, Gainey M, Grewenig P, Meyer J, Flentje M. Is ad-hoc plan adaptation based on 2-step IMRT feasible? Radiother Oncol 2009;93:266–72.
- Fu W, Yang Y, Yue NJ, Heron DE, Hug MS. A cone beam CT-guided online plan modification technique to correct interfractional anatomic changes for prostate cancer IMRT treatment. Phys Med Biol 2009;54:1691–703.
- Thongphiew D, Wu J, Lee R, Chankong V, Yoo S, McMahon, et al. Comparison of online IGRT technique for prostate IMRT treatment: adaptive vs repositioning correction. Med Phys 2009;36:1651–62.
- Wachter-Gerstner N, Wachter S, Reinstadler E, Fellner C, Knocke T, Pötter R. The impact of sectional imaging on dose escalation in endocavitary HDR-brachytherapy of cervical cancer: results of a prospective comparative trial. Radiother Oncol 2003;68:51–9.
- Ahmad R, Bondar L, Voet P, Mens J-W, Quint S, Dhawtal G, et al. A margin-of-the-day online adaptive intensity-modulated radiotherapy strategy for cervical cancer provides superior treatment accuracy compared to clinically recommended margins. a dosimetric evaluation. Acta Oncol 2013;52:1430–6.
- Nagano A, Minohara S, Kato S, Kiyohara H, Ando K. Adaptive radiotherapy based on the daily regression of a tumor in carbon-ion irradiation. Phys Med Biol 2012;57:8343–56.
- Ohkubo Y, Ohno T, Noda S, Kubo S, Nakagawa A, Kawahara M, et al. Interfractional change of high-risk CTV D90 during image-guided brachytherapy for uterine cervical cancer. J Radiat Res 2013;54:1138–45.
- Wanderås A, Sundset M, Langdal I, Danielsen S, Frykholm G, Marthinsen A. Adaptive brachytherapy of cervical cancer, comparison of conventional point A and CT based individual treatment planning. Acta Oncol 2012;51:345–54.
- Kerkhof E, Raaymakers B, van der Heide U, van de Blunt L, Jürgenliemk-Schultz I, Lagendijk J. Online MRI guidance for healthy tissue sparing in patients with cervical cancer: an IMRT planning study. Radiother Oncol 2008;88:241–9.
- Nielson CE, Yartsev S. A case study for online plan adaptation using helical tomotherapy. J Med Phys 2012;37:97–101.
- Vestergaard A, Søndergaard J, Petersen JB, Høyer M, Muren LP. A comparison of three adaptive strategies in image-guided radiotherapy of bladder cancer. Acta Oncol 2010;49:1069–76.
- Vestergaard A, Muren LP, Søndergaard J, Elstrøm UV, Høyer M, Petersen JB. Adaptive plan selection vs. re-optimisation in radiotherapy for bladder cancer: a dose accumulation comparison. Radiother Oncol 2013;109:457–62.
- Wright P, Muren LP, Høyer M, Malinen E. Evaluation of adaptive radiotherapy of bladder cancer by image-based tumour control probability modelling. Acta Oncol 2010;49:1045–51.
- Webster GJ, Stratford J, Rodgers J, Livsey JE, Macintosh D, Choudhury A. Comparison of adaptive radiotherapy techniques for the treatment of bladder cancer. Br J Radiol 2013;86:20120433.
- Foroudi F, Wong J, Haworth A, Baille A, McAlpine J, Rolfo A, et al. Offline adaptive radiotherapy for bladder cancer using cone beam computed tomography. J Med Imaging Radiat Oncol 2009;53:226–33.
- Nijkmap J, Marijnen C, van Herk M, van Triest Sonke J-J. Adaptive radiotherapy for long course neo-adjuvant treatment of rectal cancer. Radiother Oncol 2012;103:353–9.
- Michalski J, Winter K, Roach M, Markoe A, Sandler HM, Ryu J, et al. Clinical outcome of patients treated with 3D conformal radiation therapy (3D-CRT) for prostate cancer on RTOG 9406. Int J Radiat Oncol Biol Phys 2012;83:e363–70.
- Peeters ST, Heemsbergen WD, Koper PC, van Putten WL, Slot A, Dielwart MF, et al. Dose-response in radiotherapy for localized prostate cancer: results of the Dutch multicenter randomized phase III trial comparing 68 Gy of radiotherapy with 78 Gy. J Clin Oncol 2006;24:1990–6.
- Zelefsky MJ, Fuks Z, Hunt M, Lee HJ, Lombardi D, Ling CC, et al. High dose radiation delivery by intensity modulated conformal radiotherapy improves outcome of localized prostate cancer. J Urol 2001;166:876–81.
- Beckendorf V, Guerif S, Le Prisé E, Cosset JM, Bougnoux A, Chauvet B, et al. 70 Gy versus 80 Gy in localized prostate cancer: 5-year results of GETUG 06 randomized trial. Int J Radiat Oncol Biol Phys 2011;80:1056–63.
- Leborgne F, Fowler J. Late outcomes following hypofractionated conformal radiotherapy vs. Standard fractionation for localized prostate cancer: a nonrandomized contemporary comparison. Int J Radiat Oncol Biol Phys 2009;74:1441–6.
- Martin JM, Bayley A, Bristow R, Chung P, Gospodarowicz M, Menard C, et al. Image guided dose escalated prostate radiotherapy: still room to improve. Radiat Oncol 2009;4:50.
- Burridge N, Amer A, Marchant T, Sykes J, Stratford J, Henry A, et al. Online adaptive radiotherapy of the bladder: small bowel irradiated-volume reduction. Int J Radiat Oncol Biol Phys 2006;66:892–7.