Abstract
Background: Adjuvant radiotherapy (RT) of left-sided breast cancer (LSBC) with voluntary deep inspiration breath hold (vDIBH) technique reduces the cardiac dose. In this study, the effect of marker block position and the efficacy of breath hold level (BHL) correction based on lateral kV setup images are evaluated to improve the daily reproducibility. Material and methods: A total of 148 consecutive LSBC patients treated with vDIBH RT were included in this study. The real-time position management (RPM) marker block was placed on the abdominal wall in 63 patients (group A) and on the sternum in 85 patients (group S). Acquired 900 (group A) + 1040 (group S) orthogonal image pairs were retrospectively analyzed. The actual BHL was determined from the lateral kV images. The height of the BHL gating window in RPM was corrected if errors of the actual BHL exceeded 4 mm. Setup margins were calculated for the chest wall and for bony surrogates of the lymph node regions. Results: The sternal marker block reduced the random residual errors in the actual BHL (p < 0.05). The BHL correction was required for 26/63 patients in group A and for 26/85 patients in group S. Correction of the BHL window significantly reduced both the systematic and the random residual error in both groups. In patients with lymph node irradiation, the effect of both marker placement and BHL window correction was significant in the superior-inferior direction. Correction of the BHL reduced the mean cardiac dose by 0.5 Gy (p < 0.01) in group A and 0.6 Gy (p < 0.05) in group S. Conclusions: Reproducibility of the BHL can be improved by placing the marker block on the sternum and correcting the height of the BHL window based on lateral kV setup images. Acquisition of lateral kV images in the first 3 fractions and once a week during RT is recommended.
Adjuvant radiotherapy (RT) of left-sided breast cancer (LSBC) increases long-term cardiac morbidity and mortality [Citation1–3]. This excess risk has been shown to be dose-dependent [Citation2] and the heart should be spared from radiation as much as possible. Deep inspiration breath hold (DIBH) techniques decrease the dose to the heart and coronary arteries significantly in LSBC RT compared with free breathing (FB) [Citation4–6]. This dose sparing in the heart reduces the later cardiac complication probabilities in NCTP-models [Citation7]. The voluntary deep inspiration breath hold (vDIBH) method can be used for RT of the breast only as well as in the treatment of axillary and supraclavicular lymph node areas [Citation8]. The daily in-room time in breast cancer RT is only a few minutes more with vDIBH compared with FB, which has further resulted in adopting this technique in routine use.
Intra- and inter-fraction motion and subsequent displacement errors must be validated while deploying new techniques in daily use. Breath hold levels (BHL) may differ between the planning computer tomography (CT) scan and the start of the RT due to patient-derived reasons (improved or decreased inspiration). Utilizing patient-based monitors for the visual guidance of vDIBH increases the daily reproducibility of this technique [Citation6]. However, large inter-fraction setup errors have been found [Citation9–11].
In our unit, the BHL is detected using the real-time position management (RPM) system, which tracks the movement of an external marker block. The marker block used can be placed either on the abdominal wall at the level of the diaphragm [Citation5,Citation6,Citation12] or on bony sternum [Citation8,Citation13]. RPM measures the BHL only indirectly and in anterior-posterior (AP) dimension – thus the actual BHL must be re-verified in the treatment situation [Citation9,Citation10]. Changes in the actual BHL can be measured as position errors between the sternum and vertebra in the lateral (LAT) kV images [Citation12]. Tangential kV/MV or AP kV images are essential in verifying the target coverage and optimal patient positioning, but are insufficient alone to estimate the BHL.
In our previous work with the RPM system [Citation14], we estimated the actual BHL from the distance between the vertebrae and the sternum in the LAT images. Systematic errors in AP and superior-inferior (SI) directions were 3.4 mm and 5.0 mm, respectively. In addition, we observed large inter-fraction random position errors and therefore adopted strict daily image guidance for vDIBH patients.
Our previous findings encouraged us to further improve the reproducibility of vDIBH. First, we aimed to evaluate the effect of marker block positioning between the upper abdomen and sternum. Second, we verified whether the BHL observed in RPM corresponded to the true BHL measured from the LAT kV images and whether its correction results in improved delivery accuracy in the target regions as well as diminished cardiac doses during adjuvant LSBC RT.
Material and methods
Patient group and the planning CT
In this retrospective study, we included 148 consecutive LSBC patients receiving adjuvant RT with the vDIBH technique following breast conserving surgery or mastectomy. The mean age of the patients was 59 years (range 34–74 years).
The planning CT was done in supine position with both arms lifted above the head. The Varian RPM respiratory gating system (Varian Medical Systems, Palo Alto, CA) was used to verify the BHL. A marker block with two infrared reflectors was placed on the abdominal wall between the lower sternum and umbilicus on the average of 4 cm from the xyphoid process for the first 63 patients constituting group A. The second group of 85 patients (group S) had the marker block placed on the bony sternum 4 cm cranially from the xyphoid process. Before the planning CT, patients were audio coached to perform reproducible and stable breath holds for periods of 12–15 s at least 4–5 times and one breath hold exceeding 20 s. The gating window was set to a maximum of ±5 mm around the average BHL and individually further narrowed based on the stability of at least three breath holds before the acquisition of the actual planning CT in DIBH. All patients had monitors to visually guide breathing levels.
For the breast only, 50 Gy at 25 fractions or 42.56 Gy at 16 fractions with tangential field technique were used. For patients requiring lymph node region RT (in group A n = 25, in group S n = 30), only conventional 50/2 Gy fractionation was used and two or three additional fields to cover the axillary and supraclavicular lymph node regions were included.
Image guidance protocol
In our institutional vDIBH image guidance protocol, the treatment couch corrections are based on anterior and LAT setup images acquired using an on-board imaging system (OBI, Varian Medical Systems) at least in the first 3 fractions for all patients. The BHL is verified from the LAT image once a week thereafter. Tangential breast images are acquired daily. Anterior image is acquired once a week for the patients with lymph nodes involved to correct the potential patient rotation. Couch corrections are required if position errors of the bony structures in the tangential breast images and/or residual errors of the bony landmarks for the lymph node areas exceed 4 mm, and/or the BHL deviates more than 4 mm from the planned. In this case, additional anterior and LAT orthogonal images are acquired at the next 2 fractions after the corrections to ensure the validity of corrections.
For these 148 patients, 900 (group A) + 1040 (group S) orthogonal setup images were matched online by experienced radiation therapists. Image matching was performed to the midway compromise of the ribs and the sternum as presented in our previous study [Citation14].
Estimation and correction of BHL
The reference BHL was recorded in the AP direction using the RPM system during the planning CT. The breath hold at the start of RT was advised on the basis of these data and by using a patient-based monitor for visual guidance. The actual BHL was determined from the distance between the sternum and the middle part of the vertebra, taking into account the movement of bony structures both in AP- and SI direction, as presented in . If the measured BHL differed by more than 4 mm from that in the LAT reference image (reconstructed from planning CT) in 3 successive fractions, the height of the BHL window in the RPM was adjusted to obtain an optimal match with the reference image. The amount of correction was the average error of the BHL determined by offline analysis. The correction was further confirmed by a designated group of radiation therapists and physicists. For patients with BHL correction, the BHL values before the first correction and after the successful correction were called “uncorrected” and “corrected”, respectively.
Figure 1. The lateral setup image applied for the image guidance. The black solid line represents the target volume including the breast and lymph node regions. Bony landmarks (white lines) were chosen in the vertebrae and sternum to determine the actual BHL. BHL error was defined as the difference between optimal matches of these structures in both AP- and SI-directions (white arrows).
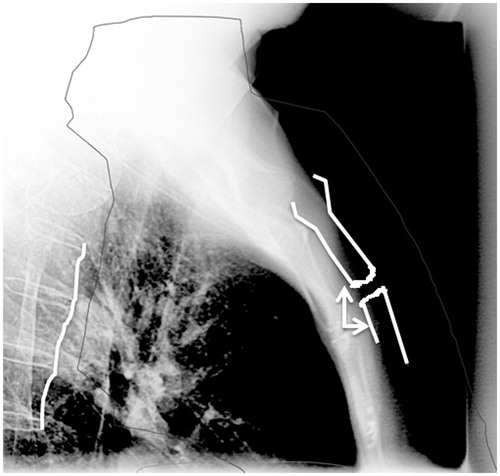
Estimation of the setup errors and margins for the lymph node surrogates and chest wall
The upper vertebrae and clavicula were used as bony surrogates for the lymph node regions in AP images and the chest wall for the breast tissue in tangential images as previously described [Citation14] (Supplementary Figure 1, available online at http://www.informahealthcare.com). Residual errors of these structures were defined as maximal errors determined offline by matching the reference and OBI images after the couch corrections. Setup margins for the lymph node region surrogates and for the chest wall were calculated using van Herk’s formula m = 2.5Σ + 0.7σ [Citation15], where Σ denoted systematic error and σ random error, as presented by Greener [Citation16].
Effect of the BHL correction on the displacement of the heart
The potential error in distance of the heart surface inside the treatment field was retrospectively analyzed from the tangential images (). The effect of the BHL correction on the heart position inside the treatment field and the subsequent cardiac dose were analyzed. Investigation of the heart displacement during treatment was also done for patients without the need of BHL corrections due to stable and reproducible BHLs (within ±2 mm in the LAT image).
Figure 2. The error in distance of the heart surface inside the tangential treatment field was retrospectively analyzed from the tangential images. A) tangential image of the planning CT. B) and C) daily tangential images of the same patient in treatment position before and after the BHL correction, respectively. The heart surface is pointed by arrows.
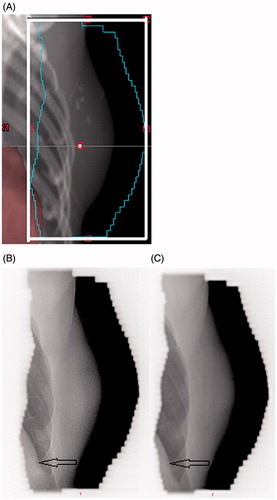
Statistical analysis
Statistical tests were used to evaluate whether the difference of position errors is statistically significant before and after the BHL correction. As the systematic errors were normally distributed, the two-tailed F-test was applied for systematic errors (test for equality of variances). The Wilcoxon rank sum test was applied for random errors (test for equality of means). The study groups were compared using the t-test for paired or independent samples. A p-value ≤0.05 was considered statistically significant.
Results
Errors in BHL and required corrections
The BHL correction was required for 26/63 (41%) patients in group A, and for 26/85 (31%) patients in group S (p = 0.18) as the systematic error of the actual BHL in LAT images exceeded the predefined 4 mm tolerance. For these patients, the mean BHL errors before the correction were 3.1 mm (range −2.6–7.7 mm) in group A and 1.4 mm (−4.2–6.5 mm) in group S in the AP direction and −3.7 mm (−12.8–5.6 mm) versus −1.4 mm (−10–9 mm) in the SI direction, respectively.
The gating window width for the BHL was individually determined during the planning CT with an average of ±3.6 mm (min ±2 mm, max ±5 mm) in group A and ±2.6 mm (±1.5, ±4.5) in group S. The mean BHL window height, which is defined at the center of the RPM gating window, was 2.7 cm (range 1.4–4.3 cm) in group A and 1.8 cm (1.2–3.2 cm) in group S. To correct the actual BHL, the level of gating window in RPM was lifted in 22 patients and lowered in four patients in group A. In group S, the window level was lifted in 20 and lowered in six patients. The average window level correction was 3.0 mm (1.4–10.0 mm) in group A and 2.7 mm (1.9–7.0 mm) in group S. There was no statistical difference between the horizontal angle of the marker block between the groups A and S nor between those requiring BHL correction.
Effect of the BHL correction on position errors and setup margins
The effect of the BHL correction in the RPM on the treatment reproducibility is shown in . With the BHL correction, both the systematic error Σ and the random error σ were significantly reduced in both groups. In group A, the residual errors were nearly halved with the correction. In group S, the decrease in Σ was more moderate, but still statistically significant. A similar effect was observed in lymph node-positive patients with respect to errors in the upper vertebrae in the SI direction (). The setup margins could be reduced even by 5 mm in the SI direction in group A and 3.4 mm in group S.
Table 1. Residual errors (mm) in the actual breath hold level (BHL) measured as a distance between the upper sternum and middle part of the vertebrae during the treatment course. The difference between the abdominal marker group A and the sternal marker group S is shown. Actual treatment position after the on-line matching with bony structures is presented. In addition, the effect on residual errors after the BHL window correction is evaluated.
Table 2. For patients with lymph node region radiation, the residual location errors and setup margins (mm) of the upper vertebra as a surrogate for the lymph node region are presented. The comparison between the abdominal marker group A and the sternal marker block group S is shown as well as the effect of the breath hold level (BHL) correction. The setup margins are calculated with van Herks’ formula.
Residual errors for the clavicula before and after the BHL correction are presented in Supplementary Table S1 (available online at http://www.informahealthcare.com). Significant changes were not observed. The results in and Supplementary Table S1 demonstrate that the BHL correction had only a minor effect on position errors in the LAT direction for the surrogates of the lymph node region. The random residual errors and setup margins required for the chest wall are presented in Supplementary Table S2 (available online at http://www.informahealthcare.com). The BHL correction decreased the errors in the SI direction (p < 0.05) but the effect on the calculated margins was modest: from 7.0 mm to 5.0 mm in group A and from 7.6 mm to 5.5 mm in group S.
Effect of the marker block position
Random residual errors in the actual BHL were significantly decreased by placing the marker block on the sternum instead of on the abdominal wall. σ reduced from 2.3 mm to 1.8 mm (p < 0.05) in the AP direction and from 3.0 mm to 2.5 mm (p < 0.05) in the SI direction. Σ decreased from 3.1 mm to 2.6 mm (p = 0.13) in AP direction and from 4.2 mm to 3.7 mm (p = 0.13) in SI direction with the use of the sternal marker placement ().
For patients with lymph node region irradiation (n = 25 in group A, n = 30 in group S), the sternal marker block decreased significantly both the Σ (from 3.9 mm to 2.6 mm) and σ (from 2.8 mm to 2.1 mm) of the residual errors in the upper vertebra in the SI direction (p < 0.05), but the errors in the LAT direction were similar with both marker positions ().
Displacement of the heart before and after BHL correction
From BHL corrected patients, 8/26 in group A and 9/26 in group S had the heart near or inside the LAT beam and had evaluable heart surface in the tangential images. The mean signed residual error of the heart location was significantly reduced from 5.5 mm to 2.0 mm after the BHL correction in group A (n = 8, p = 0.02) and from 3.3 mm to 0.8 mm in group S (n = 9, p < 0.01). As a reference, 30 evaluable patients with reproducible BHLs had the mean signed displacement of the heart 2.9 mm (n = 15 group A) and 2.0 mm (n = 15 group S) anteriorly.
The effect of the BHL correction was evaluated from planning CT. The average mean cardiac dose in group A would have been 2.6 Gy if uncorrected and 2.1 Gy after the correction (p < 0.01), and in group S 2.9 Gy and 2.3 Gy (p < 0.05), respectively. The volume of the heart receiving 10 Gy (V10Gy) decreased from 3.8% to 2.5% (p < 0.05) in group A and from 4.5% to 2.8% (p < 0.05) in group S.
Discussion
The effect of cardiac dose sparing with DIBH has been widely studied [Citation4,Citation5,Citation10] and is a preferable method in adjuvant supine RT of LSBC patients. vDIBH and active breathing control (ABC) are comparable techniques in terms of positional reproducibility and normal tissue sparing [Citation10]. However, both of these methods are recognized to have inter-fraction variability in treatment position.
In this study, we investigated the reproducibility vDIBH for 148 LSBC patients receiving adjuvant RT. vDIBH was guided by the RPM and the true BHL was verified using the LAT OBI images. Two different marker block positions were reviewed and the effect of active BHL correction was evaluated. The setup margins were calculated for the clavicula and upper vertebra as a surrogate of lymph node regions and the chest wall before and after corrections of the BHL. We also investigated the effect of the correction for the position of the heart in the treatment field.
The RPM system uses the infrared reflective marker block for the tracking of breath movements. In previous studies, the marker block has been placed differently with various gating window levels. Hjelstuen et al. [Citation8] placed the marker block on the xiphoid process with a gating window of ±1 mm. Damkjaer et al. placed the block on bony structures close to the sternum and restricted the gating window to ±1–1.5 mm. In studies by Hayden et al. [Citation5], Sung et al. [Citation6] and Bruzzatini et al. [Citation4], the marker block was placed below the xiphoid process on soft abdominal wall with gating windows of ±2.5 mm, ±1.5 mm and ±5 mm, respectively. Lutz et al. [Citation11] used two different marker block positions in their study: one on the inferior part of the sternum and the other laterally from the xiphoid process with a reported gating window from 1.6 mm to 3.6 mm.
The breath movement in thoracic bony structures is naturally smaller than in diaphragmatic area. In our patients, the average BHL in the RPM window was 1.8 cm in group S and 2.7 cm in group A. The gating window levels and widths were individually adjusted both in the planning CT and in the treatment phase, such that the actual treatment window was on average ±2.6 mm in group S and ±3.6 mm in group A. The random errors in the actual BHL and both the systematic and random errors in the upper vertebrae in the SI direction were significantly smaller in group S than in group A. The magnitude of the BHL correction was similar in both groups (3 mm and 2.7 mm). Our results favor the sternal marker block placement to minimize the inter-fraction setup errors.
Visual guidance with goggles or monitors improves the reproducibility of breath hold, as presented by Damkjaer et al. [Citation13,Citation17]. Rong et al. found that the RPM system alone is not sufficient to verify the BHL [Citation18] and used three-dimensional (3D) surface imaging as a second method. Likewise, 3D surface imaging was used by Betgen et al. [Citation9] to quantify the intra- and inter-fraction differences and found that the inter-fraction variability was particularly significant. The same observation was made by Fassi et al. [Citation19] using a spirometer-based DIBH with external skin markers. Lutz et al. [Citation11] used continuous portal imaging to evaluate the setup error and motion during vDIBH and found that despite a small gating window, large errors occurred in the chest wall position in some patients. In a study by McIntosh et al. [Citation12], the BHL was measured from LAT kV images. In these 10 patients, the reproducibility of the BHL relative to bony structures was good (in the AP direction 1 mm given as 1SD). However, these studies demonstrated that the RPM system alone has limitations and further image guidance is required.
In our previous study, using an abdominal marker, we found the Σ in the BHL was 5.0 mm in the SI direction and 3.4 mm in the AP direction [Citation14]. In this present study, even without any corrections, the Σ was slightly better: 4.2 mm in the SI direction and 3.1 mm in the AP direction in group A. Visual guidance was used for all patients in this study with in-room monitors, which might explain the improved results. However, BHL corrections in the RPM window were necessary for 4/10 in group A and 3/10 in group S.
The values presented in indicated that systematic displacement errors are present and the actual BHL changed between the planning CT and start of treatment. In 90% of patients, the systematic errors were already present in the first 3 fractions, which advocates the daily image guidance protocol at the start of the treatment. Prominent errors were found in orthogonal images in the SI direction, which is unevaluable in the RPM system. The BHL correction significantly improved both residual Σ and σ with respect to bony structures in both groups and decreased the estimated setup margins by 5–7 mm for the lymph node region and 2.0–2.1 mm for the chest wall in the SI direction. The BHL correction did not diminish marginal errors in the clavicula, which suggests that the clavicula moves independently from other landmarks and is not strongly related to the BHL. In addition, the results in and Supplementary Table S1 demonstrate that the BHL correction has only a minor effect on position errors in the LAT direction for the lymph node region surrogates and chest wall.
For the majority of patient needing the BHL correction (22 vs. 4 in group A and 20 vs. 6 in group S), there was a need to lift the gating window, even though the patient’s breath hold was within the predefined window in RPM. For these patients, the RPM and monitor guided the breath hold to be less deep than in the planning CT. One possible explanation for this phenomenon is a slightly different angle of the in-room infrared camera compared with the one used in the planning CT – even though the BHLs in both RPM systems were within 1 mm when measured with a phantom. Yet with the BHL correction and patient guidance, all patients were able to increase the breath hold volume to the desired level. However, 10 patients increased their breath hold volume in the treatment phase compared with the planning CT. This error does not lead to increased cardiac doses, but has to be corrected to ensure optimal target coverage.
Altogether, the smallest errors were obtained with the combination of sternal marker placement, correction of the BHL and individual adjustment of the window width.
The heart position variability during vDIBH was previously evaluated by McIntosh et al. [Citation12] with average heart position shifts with respect to bony anatomy by 2 ± 3 mm in the SI-, 1 ± 2 mm in the LAT- and 1 ± 3 mm in the AP direction. The authors recognized that the heart position variability required further analyses to ensure optimal cardiac protection. Similarly, Alderliesten et al. [Citation20] found moderate heart position variability with respect to the breast surface. Likewise, in our study, the patients with good BHL reproducibility and without any corrections had the mean signed displacement of the heart 2.0 mm (group S) and 2.9 mm (group A) to the anterior direction. With active BHL correction, we were able to reduce the residual errors in heart position even more, and in addition, decrease the mean cardiac doses by 0.5 Gy (group A) and 0.6 Gy (group S). The average absorbed dose from one planar kV image is 1–3 mGy [Citation21]. In a study by Alvarado et al, the cardiac dose from AP + LAT kV imaging pair was 1.6 ± 0.3 mGy [Citation22]. Regarding the tangential imaging, the mean cardiac dose with MV was measured to be 0.3 ± 0.8 mGy by Bahig et al. [Citation23]. To calculate the maximal cardiac dose from tangential kV imaging, an estimation of 1 mGy dose/fraction could be made. Therefore, the total additional dose to the heart from daily IGRT is maximally 72.5 mGy in our protocol (orthogonal setup kV image pair and one tangential kV image per session, 25 sessions).
On the basis of our experience, we now routinely place the marker block on the sternum. Both orthogonal and tangential images are acquired at 3 first fractions to confirm the matching position and the BHL. Tangential images are acquired daily. Online corrections are made based on bony structures. If errors in the BHL occur, then the BHL correction is determined based on kV images acquired at 3 consecutive fractions and after the shift verified by two consecutive days and once or twice a week thereafter with LAT images. In addition, AP images are acquired once a week with lymph node irradiation to confirm the position of the upper vertebrae and clavicula.
Conclusions
The sternal marker block placement diminished random errors in the BHL and decreased residual errors in the lymph node region surrogates compared to an abdominal marker block. The BHL correction based on LAT kV images significantly improved the reproducibility of the vDIBH. The best results were obtained with individually verified BHL and a corrected gating window level with the sternal marker block. Image guidance in the treatment phase is mandatory to maintain the target coverage and to protect the heart.
Funding information
This study was supported by the Pirkanmaa Hospital District, Elna Kaarina Savolainen fund (Grant No. R12536).
Supplementary_table_2.DIBH.doc
Download MS Word (39 KB)Supplementary_table_1_DIBH.doc
Download MS Word (46 KB)Supplementary_figure_1_DIBH.jpg
Download JPEG Image (40 KB)Disclosure statement
The authors report no conflicts of interest. The authors alone are responsible for the content and writing of the paper.
References
- Bouillon K, Haddy N, Delaloge S, Garbay JR, Garsi JP, Brindel P, et al. Long-term cardiovascular mortality after radiotherapy for breast cancer. J Am Coll Cardiol 2011;57:445–52.
- Darby SC, Ewertz M, McGale P, Bennet AM, Blom-Goldman U, Bronnum D, et al. Risk of ischemic heart disease in women after radiotherapy for breast cancer. N Engl J Med 2013;368:987–98.
- Darby S, McGale P, Correa C, Taylor C, Arriagada R. Early Breast Cancer Trialists' Collaborative Group (EBCTCG), et al. Effect of radiotherapy after breast-conserving surgery on 10-year recurrence and 15-year breast cancer death: meta-analysis of individual patient data for 10,801 women in 17 randomised trials. Lancet 2011;378:1707–16.
- Bruzzaniti V, Abate A, Pinnaro P, D'andrea M, Infusino E, Landoni V, et al. Dosimetric and clinical advantages of deep inspiration breath-hold (DIBH) during radiotherapy of breast cancer. J Exp Clin Cancer Res 2013;32:88–95.
- Hayden AJ, Rains M, Tiver K. Deep inspiration breath hold technique reduces heart dose from radiotherapy for left-sided breast cancer. J Med Imaging Radiat Oncol 2012;56:464–72.
- Sung K, Lee KC, Lee SH, Ahn SH, Lee SH, Choi J. Cardiac dose reduction with breathing adapted radiotherapy using self respiration monitoring system for left-sided breast cancer. Radiat Oncol J 2014;32:84–94.
- Korreman SS, Pedersen AN, Aarup LR, Nottrup TJ, Specht L, Nystrom H. Reduction of cardiac and pulmonary complication probabilities after breathing adapted radiotherapy for breast cancer. Int J Radiat Oncol Biol Phys 2006;65:1375–80.
- Hjelstuen MH, Mjaaland I, Vikstrom J, Dybvik KI. Radiation during deep inspiration allows loco-regional treatment of left breast and axillary-, supraclavicular- and internal mammary lymph nodes without compromising target coverage or dose restrictions to organs at risk. Acta Oncol 2012;51:333–44.
- Betgen A, Alderliesten T, Sonke JJ, van Vliet-Vroegindeweij C, Bartelink H, Remeijer P. Assessment of set-up variability during deep inspiration breath hold radiotherapy for breast cancer patients by 3D-surface imaging. Radiother Oncol 2013;106:225–30.
- Bartlett FR, Colgan RM, Carr K, Donovan EM, McNair HA, Locke I, et al. The UK HeartSpare Study: randomised evaluation of voluntary deep-inspiratory breath-hold in women undergoing breast radiotherapy. Radiother Oncol 2013;108:242–7.
- Lutz CM, Poulsen PR, Fledelius W, Offersen BV, Thomsen MS. Setup error and motion during deep inspiration breath-hold breast radiotherapy measured with continuous portal imaging. Acta Oncol 2016;55:193–200.
- McIntosh A, Shoushtari AN, Benedict SH, Read PW, Wijesooriya K. Quantifying the reproducibility of heart position during treatment and corresponding delivered heart dose in voluntary deep inhalation breath hold for left breast cancer patients treated with external beam radiotherapy. Int J Radiat Oncol Biol Phys 2011;81:e569–76.
- Damkjaer SM, Aznar MC, Pedersen AN, Vogelius IR, Bangsgaard JP, Josipovic M. Reduced lung dose and improved inspiration level reproducibility in visually guided DIBH compared to audio coached EIG radiotherapy for breast cancer patients. Acta Oncol 2013;52:1458–63.
- Laaksomaa M, Kapanen M, Haltamo M, Skyttä T, Peltola S, Hyödynmaa S, et al. Determination of the optimal matching position for setup images and minimal setup margins in adjuvant radiotherapy of breast and lymph nodes treated in voluntary deep inhalation breath-hold. Radiat Oncol 2015;10:76–85.
- van Herk M, Remeijer P, Rasch C, Lebesque JV. The probability of correct target dosage: dose-population histograms for deriving treatment margins in radiotherapy. Int J Radiat Oncol Biol Phys 2000;47:1121–35.
- Greener T. Practical determination of systematic and random set-up errors, Σ set-up and σ set-up using portal imaging. The British Institute of Radiology: Geometric Uncertainties in Radiotherapy. London, UK; 2003:36–43.
- Cervino LI, Gupta S, Rose MA, Yashar C, Jiang SB. Using surface imaging and visual coaching to improve the reproducibility and stability of deep-inspiration breath hold for left-breast-cancer radiotherapy. Phys Med Biol 2009;54:6853–65.
- Rong Y, Walston S, Welliver MX, Chakravarti A, Quick AM. Improving intra-fractional target position accuracy using a 3D surface surrogate for left breast irradiation using the respiratory-gated deep-inspiration breath-hold technique. PLoS One 2014;9:e97933.
- Fassi A, Ivaldi GB, Meaglia I, Porcu P, Tabarelli de Fatis P, Liotta M, et al. Reproducibility of the external surface position in left-breast DIBH radiotherapy with spirometer-based monitoring. J Appl Clin Med Phys 2014;15:130–40.
- Alderliesten T, Betgen A, Elkhuizen PH, van Vliet-Vroegindeweij C, Remeijer P. Estimation of heart-position variability in 3D-surface-image-guided deep-inspiration breath-hold radiation therapy for left-sided breast cancer. Radiother Oncol 2013;109:442–7.
- Goyal S, Kataria T. Image guidance in radiation therapy: techniques and applications. Radiol Res Pract 2014;2014:705604
- Alvarado R, Booth JT, Bromley RM, Gustafsson HB. An investigation of image guidance dose for breast radiotherapy. J Appl Clin Med Phys 2013;14:25–38.
- Bahig H, Roussin E, Yassa M, Vavassis P, Lemaire C, Archambault L, et al. Partial kilovoltage cone beam computed tomography, complete kilovoltage cone beam computed tomography, and electronic portal images for breast radiation therapy: A dose-comparison study. Pract Radiat Oncol 2015;5:e521–9.