Abstract
Objectives. Active inflammatory responses play an important role in the pathogenesis of depression. We hypothesized that the rapid antidepressant effect of ketamine is associated with the down-regulation of pro-inflammatory mediators.
Methods. Forty-eight rats were equally randomized into six groups (a control and five chronic unpredictable mild stress (CUMS) groups) and given either saline or 10 mg/kg ketamine, respectively. The forced swimming test was performed, and the hippocampus was subsequently harvested for the determination of levels of interleukin (IL)-1β, IL-6, tumour necrosis factor-α (TNF-α), indoleamine 2,3-dioxygenase (IDO), kynurenine (KYN), and tryptophan (TRP).
Results. CUMS induced depression-like behaviours and up-regulated the hippocampal levels of IL-1β, IL-6, TNF-α, IDO, and the KYN/TRP ratio, which were attenuated by a sub-anaesthetic dose of ketamine.
Conclusion. CUMS-induced depression-like behaviours are associated with a reduction in hippocampal inflammatory mediators, whereas ketamine’s antidepressant effect is associated with a down-regulation of pro-inflammatory cytokines in the rat hippocampus.
Introduction
Depression is a common, serious, recurrent illness. In American adults, the 12-month prevalence rate of a major depressive episode is 8.3%, and the lifetime prevalence rate is 19.2% (Citation1). Unfortunately, the pathogenesis of depression remains insufficiently understood, and currently available monoamine-based therapies are associated with delayed onset times and low remission rates (Citation2). These limitations raise an urgent and unmet need for more efficacious and fast-acting antidepressant agents, particularly for depressed subjects with high suicide rates.
Recently, much attention has been paid to the hypothesis that inflammation contributes to depression. A growing body of evidence has shown that depression is associated with increased concentrations of inflammatory cytokines in the serum and cerebrospinal fluid (CSF), which is correlated with the severity of depression (Citation2-4). Recent studies and our previous results have shown that the peripheral or intra-cerebral administration of the cytokine-inducer lipopolysaccharide (LPS) and intra-cerebral injections of interleukin (IL)-6 induced a depressive behaviour in rats (Citation5-7). Furthermore, inhibition of pro-inflammatory responses improves depressive symptoms and facilitates the therapeutic efficacy of conventional antidepressants (Citation2,3). Preclinical and clinical data suggest that depression may be an inflammation-related disorder, and inhibition of pro-inflammatory responses is probably implicated in the treatment of depression. This implies that pro-inflammatory cytokines may be used as biomarkers to predict the development of depression and the therapeutic efficacy of antidepressants.
One pathway by which inflammatory stimuli contribute to depression is indoleamine 2,3-dioxygenase (IDO), which is activated by pro-inflammatory cytokines, such as IL-1, IL-6, and tumour necrosis factor-α (TNF-α) (Citation8). If activated, IDO depletes tryptophan (TRP), reduces 5-hydroxytryptamine (5-HT) in several regions of the brain, and ultimately elicits depression symptoms (Citation9). IDO activity, as evaluated by the levels of metabolites in the kynurenine (KYN) pathway, is associated with the severity of inflammation as well as depressive symptoms (Citation3). Conversely, the inhibition of IDO abrogates depression-like behaviours induced by acute or chronic pro-inflammatory stimuli (Citation10,11). Thus, IDO plays a key role in the induction of depression-like behaviours in response to inflammation.
Ketamine is a commonly used anaesthetic. The administration of a sub-anaesthetic dose of ketamine has been shown to produce rapid, robust, and long-lasting antidepressant properties (Citation12-14) and to exert anti-inflammatory reactions in both humans and animals (Citation15-17). These anti-inflammatory effects can be linked to ketamine’s antidepressant activity mainly via a direct inhibition of inflammatory cytokines and regulators and an indirect influence on the kynurenine signal pathway (Citation18). However, little attention has been paid to the anti-inflammatory signalling pathway that mediates ketamine’s antidepressant effect. We hypothesized that down-regulation of pro-inflammatory cytokines may be one of the possible mechanisms underlying ketamine’s antidepressant properties. Thus, we investigated whether ketamine alleviating depressive behaviours is related with inhibition of inflammatory responses in chronic unpredictable mild stress (CUMS) in a rat model.
Materials and methods
Animals and drug administration
The animal experiments were approved by the Ethics Committee of Jinling Hospital and were performed in accordance with the Guide for the Care and Use of Laboratory Animals from the National Institutes of Health, USA. Forty-eight male adult Wistar rats weighing 200–250 g were purchased from the Shanghai Animal Centre, Shanghai, China. They were housed five per cage with food and water available ad libitum and were maintained on a 12-h light/dark cycle (lights on at 7.00 a.m.). After 7 days of acclimatization, the rats were randomly divided into six groups of eight rats each. The control group received an intraperitoneal injection of 0.9% saline. Rats in the five CUMS groups were given an intraperitoneal injection of 0.9% saline or a sub-anaesthetic dose of ketamine (10 mg/kg) in the same volume of 1 mL. Behavioural tests were performed 0.5 h after saline administration and 0.5, 1, 2, and 4 h after ketamine administration in the CUMS groups.
CUMS
The CUMS procedure was performed as previously described (Citation19) with a slight modification. Briefly, the rats were subjected to different stressors: cage tilting for 24 h; wet bedding for 24 h (200 mL of water per individual cage, which is enough to wet the sawdust bedding); cold swimming for 5 min (at 4°C); swimming in water for 5 min (at 45°C); fasting for 48 h; water deprivation for 24 h; level shaking for 10 min; tail nip for 1 min (1 cm from the end of the tail); and inversion of the light/dark cycle for 24 h. These nine stressors were applied for 21 days, during which each stressor was applied 2–3 times. Rats in the CUMS groups received one of these stressors at a different time each day, and the same stressor was never applied consecutively so that the animals could not predict the stimulation. Rats in the control group were undisturbed except for necessary procedures, such as routine cage cleaning.
Forced swimming test (FST)
The swimming session was conducted by placing the rats in cylinders that were 65 cm tall, 30 cm in diameter, and filled with water (22–23°C) to a depth of 40 cm. All the procedures were conducted between 09.00 and 15.00. The FST was conducted for 6 min, and the immobility time (in seconds) was recorded during the last 5 min of the 6-min test by two observers blinded to the group assignment. Immobility was defined as floating in the water without struggling and only making necessary movements to keep the head above water. The water in the tank was changed after every test.
Novelty-suppressed feeding test (NSFT)
NSFT was performed as previously described (Citation20). Before testing, rats were food-deprived overnight. Rats were placed in an open field (75 cm × 75 cm × 40 cm) with a small amount of food in the centre. Animals were allowed to explore the open field for 8 min. The latency to feed, specifically the time it took for the animal to approach and take the first bite of the food, was recorded with a stopwatch. Home cage food intake was measured right after the test as a control value.
Open field test (OFT)
Before the test session of the FST, OFT was used to test whether locomotor activities were affected in the experimental rats. Locomotor activity of rats was evaluated by the OFT at 0.5 h after the administration of saline or ketamine. The apparatus consisted of an arena of 75 cm × 75 cm square floor surrounded by a 40 cm high wall. The floor of the arena was divided into 25 equal squares. Rats were placed in the centre of the square, and the numbers of crossings and rearings were subsequently recorded by the same two observers in a blinded manner. The open field was cleaned entirely after each test.
Stereotype rating
To study psychosis-like behaviours, stereotype rating was performed at 0.5 h after the last administration of saline or ketamine as in the study of Zuo and co-workers (Citation21). This activity was observed for 5 min every time. For the test, the same two observers rated the stereotyped behaviour every minute in this procedure in a blinded manner. Score 1, lying down, eyes closed (asleep); Score 2, lying down, eyes open (inactive); Score 3, normal grooming or chewing cage litter (in place activities); Score 4, moving around in the cage, sniffing, rearing (normal, alert, active); Score 5, running movement (hyperactive); Score 6, repetitive exploration of the cage at a normal level of activity (slow patterned); Score 7, repetitive exploration of the cage with hyperactivity (fast patterned); Score 8, remaining in same place in cage with fast repetitive head and/or foreleg movement (restricted); Score 9, backing up, jumping, seizures, abnormally maintained postures, dyskinetic movements (dyskinetic-reactive).
IL-1β, IL-6, and TNF-α determinations
Rat brain was removed, and the hippocampus was dissected and stored at −80°C until assayed. IL-1β, IL-6, and TNF-α levels were measured using a radioimmunoassay (RIA) (North China Institute of Bio-technology, Beijing, China). All analytical steps were performed in accordance with the manufacturer’s recommendations. The assays used can detect the biologically active form of the protein. Concentrations of IL-1β, IL-6, and TNF-α were calculated by referring to a standard curve, according to the manufacturer’s instructions.
IDO determination
IDO levels were determined using a commercially available enzyme-linked immunosorbent assay (ELISA) (R&D, Minneapolis, MN, USA) following the manufacturer’s instructions. In this procedure, flat-bottom 96-well plates were coated with anti-IDO monoclonal antibodies to bind IDO, and the plates were incubated overnight at 4°C. The plates were washed, and a second specific anti-IDO polyclonal antibody was added and the incubation went on for 60 min at 37°C so that the captured IDO bound the polyclonal antibody. After washing, the amount of specifically bound polyclonal antibody was then determined using species-specific antibody conjugated to horseradish peroxidase as a tertiary reactant. Unbound conjugate was removed by washing. The conjugates were revealed by incubating with a chromogenic substrate. The absorbency was measured using an automatic ELISA microplate reader.
TRP and KYN determinations
TRP and KYN levels were analysed by means of a high-performance liquid chromatography (HPLC) system. Rat brains were first weighed and then homogenized in 500 μL of a 0.1 N HClO4/10 μM ascorbate solution using a 1.5 mL centrifuge tube and a disposable pestle. Upon complete homogenization, the brain samples were centrifuged at 12,000g for 5 min at 4°C. After homogenization, the supernatant was extracted and loaded into a Costar Spin-X tube filter and centrifuged at 12,000g for 5 min at 4°C. For the current study, sample extracts were then diluted at 1:10 (v:v) in 0.02 N HClO4 and analysed for TRP and KYN. A standard curve was generated on each day from concentrated (2 μM) TRP and KYN made up in 0.02 N HClO4 and held at 4°C until a volume of 20 μL was injected into the system. A standard curve was created using the system software, and samples were not analysed unless a linear standard curve with r2 greater than 0.995 was achieved. The KYN/TRP ratio was calculated as an estimate of IDO activity.
Statistical analysis
Data are expressed as the mean ± SEM or n (%) and were calculated by the Statistical Product for Social Sciences (SPSS; Version 16.0, IL, USA). Comparisons between groups were performed by one-way analysis of variance followed by the Bonferroni test for post-hoc comparisons. P < 0.05 was considered statistically significant.
Results
Effects of CUMS exposure and ketamine on the locomotor activity and stereotypic movements
Compared with the controls, rats subjected to the CUMS exposure did not present any changes in the crossings and rearings (P > 0.05) of the locomotor activity and the stereotypic movements (P > 0.05). To investigate the effects of ketamine on the locomotor activity and psychosis-like behaviour of the rats, we injected 10 mg/kg ketamine into the rats subjected to CUMS exposure. It was found that rats given ketamine at 0.5, 1, 2, and 4 h had unaffected locomotor activities and stereotypic movements (all P > 0.05).
Effects of CUMS exposure and ketamine on the immobility time during FST and the latency to feed during NSFT
Compared with the controls, rats subjected to CUMS exposure presented a significantly increased immobility time (P < 0.001, ) and latency to feed (P < 0.05, ). To investigate the effects of ketamine on the depressive-like behaviours of the rats, we injected ketamine to the rats subjected to CUMS exposure. It was found that ketamine administration prevented the CUMS-induced increase in immobility time and latency to feed at 0.5, 1, 2, and 4 h (all P < 0.05) ().
Figure 1. Effects of CUMS exposure and ketamine administration on immobility time during FST and latency to feed during NSFT of rats. Data are presented as the mean ± SEM of 8 rats per group. *P < 0.05, ***P < 0.001, versus control, # P < 0.05, ## P < 0.01, ### P < 0.001, versus CUMS (saline). Ket = ketamine.
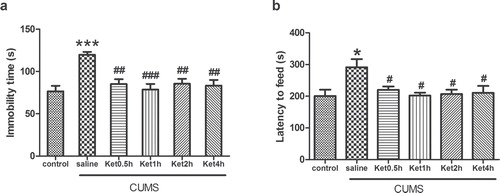
Levels of IL-1β, IL-6, and TNF-α in the hippocampus
Compared with the control group, the levels of IL-1β, IL-6, and TNF-α measured by RIA were significantly increased in the rat hippocampus after CUMS exposure (P < 0.05). To determine the effects of ketamine on the pro-inflammatory cytokines, we injected ketamine to rats subjected to CUMS exposure. RIA analysis revealed that ketamine administration prevented the CUMS-induced increases in IL-1β at 0.5 and 1 h, IL-6 at 0.5, 1, 2, and 4 h, and TNF-α at 2 and 4 h in the hippocampus (P < 0.05) ().
Figure 2. Effects of CUMS exposure and ketamine administration on the levels of IL-1β (A), IL-6 (B), and TNF-α (C) in the rat hippocampus. Levels of IL-1β at 0.5 and 1 h (A), IL-6 at 0.5, 1, 2, and 4 h (B), and TNF-α at 2 and 4 h (C) were reduced after ketamine treatment. Data are presented as the mean ± SEM of 8 rats per group. *P < 0.05, versus control, # P < 0.05, versus CUMS (saline). Ket = Ketamine.
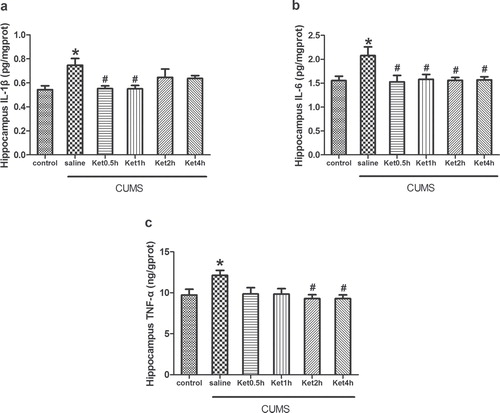
IDO levels and the KYN/TRP ratio in the hippocampus
Compared with the control group, the levels of IDO and the KYN/TRP ratio measured by ELISA and HPLC were significantly increased in the hippocampus after CUMS exposure (P < 0.05). To determine the effects of ketamine on the downstream signalling of pro-inflammatory cytokines, we injected ketamine to the rats subjected to CUMS exposure. ELISA and HPLC analysis revealed that ketamine administration prevented the CUMS-induced increase in IDO levels and the KYN/TRP ratio at 0.5, 1, 2, and 4 h in the hippocampus (P < 0.05) ().
Figure 3. Effects of CUMS exposure and ketamine administration on IDO levels (A) and the KYN/TRP ratio (B) in the rat hippocampus. IDO levels (A) and the KYN/TRP ratio (B) were reduced at 0.5, 1, 2, and 4 h after ketamine treatment. Data are presented as the mean ± SEM of 8 rats per group. *P < 0.05, versus control, ## P < 0.01, # P < 0.05, versus CUMS (saline). Ket = Ketamine.
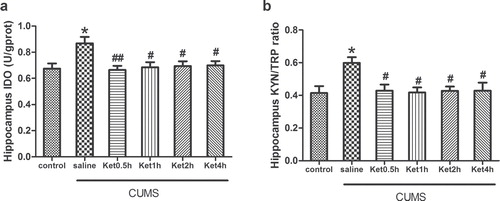
Discussion
We found that CUMS exposure induced depression-like behaviours and up-regulated the levels of IL-1β, IL-6, TNF-α, IDO, and the KYN/TRP ratio in the rat hippocampus, which were attenuated by a sub-anaesthetic dose of ketamine. This suggests that the rapid antidepressant effect of ketamine is related to the down-regulation of the inflammatory response to CUMS in the hippocampus.
Previous studies (Citation22,23) have suggested that depression is associated with increased levels of inflammatory cytokines, such as IL-1β, IL-2, IL-6, and TNF-α. Two recent meta-analyses also demonstrated higher serum IL-6 concentrations in depressed patients compared with control patients, but there were no differences in IL-10 concentrations (Citation24,25). Recently, a population-based longitudinal study demonstrated that higher levels of the systemic inflammatory marker IL-6 in childhood are associated with an increased risk of developing depression and psychosis in young adulthood (Citation26). Moreover, several lines of evidence indicate that both acute and chronic use of cytokines or cytokine inducers may lead to depressive symptoms. For example, the administration of LPS to normal mice effected acute increases in depressive symptoms (Citation27), and the injection of a Salmonella typhi vaccine to healthy controls elicited depressed moods (Citation28). In the present study, we found that the hippocampal levels of IL-1β, IL-6, and TNF-α were significantly increased after CUMS. This model is generally thought to be the most valuable and promising model of depression and is widely used to study the pathogenesis of depression and its therapeutic interventions.
It has been widely acknowledged that ketamine down-regulates inflammatory responses, and it has been recommended for use in surgery of sepsis patients due to its anti-inflammatory effects (Citation15-17). Previous studies in both animals and humans have shown that ketamine produces immunomodulatory effects and facilitates 5-HT production in the hippocampus, partly contributing to the beneficial effect of ketamine on depressive symptoms, which is consistent with our data (Citation17,29). Our previous clinical study also suggested that serum IL-6 is a predictive biomarker for the antidepressant effect of ketamine in treatment-resistant patients (Citation30).
IDO is an enzyme that regulates the first step of the KYN pathway, which can be induced by the increased production of pro-inflammatory cytokines. Recent evidence has suggested IDO as a potential link between inflammatory cytokines and the neurochemical or neuroendocrine alterations that may be responsible for the pathogenesis of depression (Citation5,8,9). The activation of IDO will promote the conversion of TRP to KYN rather than 5-HT. Two hypotheses have been put forward for the deteriorated effect of elevated KYN formation in the pathogenesis of depression. First, the up-regulation of KYN will reduce the bioavailability of TRP for the synthesis of 5-HT (Citation31). Second, the metabolism of KYN can lead to 3-hydroxykynurenine (3-HK) and the end-product of quinolinic acid (QUIN), increasing the formation of reactive oxygen species involved in neuronal apoptosis and inducing the overstimulation of N-methyl-D-aspartate (NMDA) receptors, respectively, leading to hippocampal damage and the development of depression (Citation32,33). Alternatively, the metabolism of KYN will lead to kynurenic acid (KYNA), an NMDA receptor antagonist thought to be neuroprotective. Recent studies have demonstrated decreased levels of hippocampal KYNA in depressive patients (Citation34) and decreased KYNA as well as increased QUIN in the CSF of suicidal individuals (Citation35). Furthermore, there were reduced serum KYNA to QUIN ratios in patients with major depressive disorder (Citation36,37) associated with decreased hippocampal and amygdala volumes (Citation37).
A recent study showed that IL-6 induces IDO expression through the JAK/STAT pathway (Citation38). In addition, IDO may participate in hyperactivity of the hypothalamic-pituitary-adrenal (HPA) axis during the development of depression (Citation39). Taken together, IDO plays a pivotal role in mediating depression-like behaviours in response to immune activation. In the present study, we examined the value of the KYN/TRP ratio as an estimate of IDO activity. Our study demonstrated that CUMS exposure elevated the IDO levels and activities in the hippocampus. That effect was blocked by ketamine, supporting the hypothesis that IDO activated by pro-inflammatory cytokines may contribute to the pathogenesis of depression. On the contrary, Walker et al. (Citation40) have recently reported that ketamine abrogates LPS-induced depression-like behaviours by targeting QUIN, but not by affecting inflammatory responses in the brain of C57BL/6J mice.
At the molecular level, there are scientific data available that may implicate a possible role for NMDA, a-amino-3-hydroxy-5-methyl-4-isoxazolepropionic acid, brain-derived neurotrophic factor (BDNF), mammalian target of rapamycin, and glycogen synthase kinase-3 in the rapid antidepressant action of ketamine (Citation41-43). Nevertheless, the neurotransmitters underlying the molecular and cellular activity of ketamine have not been fully elucidated. Additionally, there is evidence to suggest that ketamine is indeed changing glutamate transmission. Interestingly, inflammatory cytokines have been shown to interact with the glutamate pathways in several important ways, including increasing the release of glutamate from astrocytes and decreasing the expression of glutamate transporters on relevant glial elements. Glutamate released from astrocytes has preferential access to extra-synaptic NMDA receptors, which have been shown to decrease BDNF and increase excitotoxicity (Citation3). In addition, inflammatory cytokines, including TNF-α, have been shown to reduce glutamine synthetase (Citation44), which converts glutamate to glutamine, potentially leading to a build-up of intracellular and extracellular glutamate concentrations. Along with cytokine-mediated induction of nitrogen and oxygen free radicals, this build-up can lead to astrocyte death. Cytokines, such as TNF-α, can also reduce glutamate transporter expression on oligodendrocytes and, in excess, are directly toxic to these cells. These effects of inflammatory cytokines on glutamate metabolism, reuptake, and release by astrocytes and oligodendrocytes, as well as the fundamental integrity and survival of these glial elements, provide an intriguing intersection of the inflammation and glutamate hypotheses of depression and their relationship to treatment resistance.
In conclusion, our results suggest that depression is associated with increased inflammatory mediators and the antidepressant effect of ketamine may be associated with the down-regulation of pro-inflammatory mediators in the hippocampus. The anti-inflammatory effect of ketamine is not likely to be the only one but seems to be a major cause of its antidepressant actions.
Funding: The present study was supported by National Natural Science Foundation of China (No. 81271216).
Declaration of interest: The authors have no potential conflicts of interest to disclose.
References
- Hirschfeld RM. The epidemiology of depression and the evolution of treatment. J Clin Psychiatry. 2012;73:5–9.
- Tyring S, Gottlieb A, Papp K, Gordon K, Leonardi C, Wang A, et al. Etanercept and clinical outcomes, fatigue, and depression in psoriasis: double-blind placebo-controlled randomised phase III trial. Lancet. 2006;367:29–35.
- Miller AH, Maletic V, Raison CL. Inflammation and its discontents: the role of cytokines in the pathophysiology of major depression. Biol Psychiatry. 2009;65:732–41.
- Zunszain PA, Hepgul N, Pariante CM. Inflammation and depression. Curr Top Behav Neurosci. 2013;14:135–51.
- Dobos N, de Vries EF, Kema IP, Patas K, Prins M, Nijholt IM, et al. The role of indoleamine 2,3-dioxygenase in a mouse model of neuroinflammation-induced depression. J Alzheimers Dis. 2012;28:905–15.
- Sukoff Rizzo SJ, Neal SJ, Hughes ZA, Beyna M, Rosenzweig-Lipson S, Moss SJ, et al. Evidence for sustained elevation of IL-6 in the CNS as a key contributor of depressive-like phenotypes. Transl Psychiatry. 2012;2:e199.
- Yang C, Shen J, Hong T, Hu TT, Li ZJ, Zhang HT, et al. Effects of ketamine on lipopolysaccharide-induced depressive-like behavior and the expression of inflammatory cytokines in the rat prefrontal cortex. Mol Med Rep. 2013;8:887–90.
- Maes M. Depression is an inflammatory disease, but cell-mediated immune activation is the key component of depression. Prog Neuropsychopharmacol Biol Psychiatry. 2011;35:664–75.
- Sublette ME, Postolache TT. Neuroinflammation and depression: the role of indoleamine 2,3-dioxygenase (IDO) as a molecular pathway. Psychosom Med. 2012;74:668–72.
- O’Connor JC, Lawson MA, André C, Briley EM, Szegedi SS, Lestage J, et al. Induction of IDO by Bacille Calmette-Guérin is responsible for development of murine depressive-like behavior. J Immunol. 2009;182:3202–12.
- O’Connor JC, Lawson MA, André C, Moreau M, Lestage J, Castanon N, et al. Lipopolysaccharide-induced depressive-like behavior is mediated by indoleamine 2,3-dioxygenase activation in mice. Mol Psychiatry. 2009;14:511–22.
- Réus GZ, Carlessi AS, Titus SE, Abelaira HM, Ignácio ZM, da Luz JR, et al. A single dose of s-ketamine induces long-term antidepressant effects and decreases oxidative stress in adulthood rats following maternal deprivation. Dev Neurobiol. 2015. [Epub ahead of print].
- Ionescu DF, Luckenbaugh DA, Niciu MJ, Richards EM, Zarate CAJr. A single infusion of ketamine improves depression scores in patients with anxious bipolar depression. Bipolar Disord. 2015;17:438–43.
- Zarate CAJr, Brutsche NE, Ibrahim L, Franco-Chaves J, Diazgranados N, Cravchik A, et al. Replication of ketamine’s antidepressant efficacy in bipolar depression: a randomized controlled add-on trial. Biol Psychiatry. 2012;71:939–46.
- Yang C, Hong T, Shen J, Ding J, Dai XW, Zhou ZQ, et al. Ketamine exerts antidepressant effects and reduces IL-1β and IL-6 levels in rat prefrontal cortex and hippocampus. Exp Ther Med. 2013;5:1093–6.
- Ward JL, Harting MT, Cox CSJr, Mercer DW. Effects of ketamine on endotoxin and traumatic brain injury induced cytokine production in the rat. J Trauma. 2011;70:1471–9.
- Dale O, Somogyi AA, Li Y, Sullivan T, Shavit Y. Does intraoperative ketamine attenuate inflammatory reactivity following surgery? A systematic review and meta-analysis. Anesth Analg. 2012;115:934–43.
- Zunszain PA, Horowitz MA, Cattaneo A, Lupi MM, Pariante CM. Ketamine: synaptogenesis, immunomodulation and glycogen synthase kinase-3 as underlying mechanisms of its antidepressant properties. Mol Psychiatry. 2013;18:1236–41.
- Surget A, Wang Y, Leman S, Ibarguen-Vargas Y, Edgar N, Griebel G, et al. Corticolimbic transcriptome changes are state-dependent and region-specific in a rodent model of depression and of antidepressant reversal. Neuropsychopharmacology. 2009;34:1363–80.
- Warner-Schmidt JL, Duman RS. VEGF is an essential mediator of the neurogenic and behavioral actions of antidepressants. Proc Natl Acad Sci USA. 2007;104:4647–52.
- Zuo DY, Wu YL, Yao WX, Cao Y, Wu CF, Tanaka M. Effect of MK-801 and ketamine on hydroxyl radical generation in the posterior cingulate and retrosplenial cortex of free-moving mice, as determined by in vivo microdialysis. Pharmacol Biochem Behav. 2007;86:1–7.
- Kim JW, Szigethy EM, Melhem NM, Saghafi EM, Brent DA. Inflammatory markers and the pathogenesis of pediatric depression and suicide: a systematic review of the literature. J Clin Psychiatry. 2014;75:1242–53.
- Raison CL, Capuron L, Miller AH. Cytokines sing the blues: inflammation and the pathogenesis of depression. Trends Immunol. 2006;27:24–31.
- Dowlati Y, Herrmann N, Swardfager W, Liu H, Sham L, Reim EK, et al. A meta-analysis of cytokines in major depression. Biol Psychiatry. 2010;67:446–57.
- Hiles SA, Baker AL, de Malmanche T, Attia J. A meta-analysis of differences in IL-6 and IL-10 between people with and without depression: exploring the causes of heterogeneity. Brain Behav Immun. 2012;26:1180–8.
- Khandaker GM, Pearson RM, Zammit S, Lewis G, Jones PB. Association of serum interleukin 6 and C-reactive protein in childhood with depression and psychosis in young adult life: a population-based longitudinal study. JAMA Psychiatry. 2014;71:1121–8.
- Ma M, Ren Q, Zhang JC, Hashimoto K. Effects of brilliant blue G on serum tumor necrosis factor-α levels and depression-like behavior in mice after lipopolysaccharide administration. Clin Psychopharmacol Neurosci. 2014;12:31–6.
- Brydon L, Harrison NA, Walker C, Steptoe A, Critchley HD. Peripheral inflammation is associated with altered substantia nigra activity and psychomotor slowing in humans. Biol Psychiatry. 2008;63:1022–9.
- Rofael HZ, Turkall RM, Abdel-Rahman MS. Effect of ketamine on cocaine-induced immunotoxicity in rats. Int J Toxicol. 2003;22:343–58.
- Yang JJ, Wang N, Yang C, Shi JY, Yu HY, Hashimoto K. Serum interleukin-6 is a predictive biomarker for ketamine’s antidepressant effect in treatment-resistant patients with major depression. Biol Psychiatry. 2015;77:e19–20.
- Capuron L, Ravaud A, Neveu PJ, Miller AH, Maes M, Dantzer R. Association between decreased serum tryptophan concentrations and depressive symptoms in cancer patients undergoing cytokine therapy. Mol Psychiatry. 2002;7:468–73.
- Dantzer R, O’Connor JC, Lawson MA, Kelley KW. Inflammation-associated depression: from serotonin to kynurenine. Psychoneuroendocrinology. 2011;36:426–36.
- Maes M, Leonard BE, Myint AM, Kubera M, Verkerk R. The new ‘5-HT’ hypothesis of depression: cell-mediated immune activation induces indoleamine 2,3-dioxygenase, which leads to lower plasma tryptophan and an increased synthesis of detrimental tryptophan catabolites (TRYCATs), both of which contribute to the onset of depression. Prog Neuropsychopharmacol Biol Psychiatry. 2011;35:702–21.
- Busse M, Busse S, Myint AM, Gos T, Dobrowolny H, Müller UJ, et al. Decreased quinolinic acid in the hippocampus of depressive patients: evidence for local anti-inflammatory and neuroprotective responses? Eur Arch Psychiatry Clin Neurosci. 2015;265:321–9.
- Bay-Richter C, Linderholm KR, Lim CK, Samuelsson M, Träskman-Bendz L, Guillemin GJ, et al. A role for inflammatory metabolites as modulators of the glutamate N-methyl-D-aspartate receptor in depression and suicidality. Brain Behav Immun. 2015;43:110–17.
- Savitz J, Drevets WC, Wurfel BE, Ford BN, Bellgowan PS, Victor TA, et al. Reduction of kynurenic acid to quinolinic acid ratio in both the depressed and remitted phases of major depressive disorder. Brain Behav Immun. 2015;46:55–9.
- Savitz J, Drevets WC, Smith CM, Victor TA, Wurfel BE, Bellgowan PS. Putative neuroprotective and neurotoxic kynurenine pathway metabolites are associated with hippocampal and amygdalar volumes in subjects with major depressive. Neuropsychopharmacology. 2015;40:463–71.
- Kim H, Chen L, Lim G, Sung B, Wang S, McCabe MF, et al. Brain indoleamine 2,3-dioxygenase contributes to the comorbidity of pain and depression. J Clin Invest. 2012;122:2940–54.
- Schiepers OJ, Wichers MC, Maes M. Cytokines and major depression. Prog Neuropsychopharmacol Biol Psychiatry. 2005;29:201–17.
- Walker AK, Budac DP, Bisulco S, Lee AW, Smith RA, Beenders B, et al. NMDA receptor blockade by ketamine abrogates lipopolysaccharide-induced depressive-Like behavior in C57BL/6J mice. Neuropsychopharmacology. 2013;38:1609–16.
- Lepack AE, Fuchikami M, Dwyer JM, Banasr M, Duman RS. BDNF release is required for the behavioral actions of ketamine. Int J Neuropsychopharmacol. 2014;18.
- Yang C, Hu YM, Zhou ZQ, Zhang GF, Yang JJ. Acute administration of ketamine in rats increases hippocampal BDNF and mTOR levels during forced swimming test. Ups J Med Sci. 2013;118:3–8.
- Koike H, Chaki S. Requirement of AMPA receptor stimulation for the sustained antidepressant activity of ketamine and LY341495 during the forced swim test in rats. Behav Brain Res. 2014;271:111–15.
- Huang TL, O’Banion MK. Interleukin-1 beta and tumor necrosis factor-alpha suppress dexamethasone induction ofglutamine synthetase in primary mouse astrocytes. J Neurochem. 1998;71:1436–42.