Abstract
Background. Intraventricular hemorrhage (IVH) in very preterm infants is a common disease associated with long-term consequences. Risk factors of IVH remain to be further defined.
Aims. To determine whether specific immunoproteins at birth predict the risk of IVH and whether their receptors are localized at the bleeding site.
Methods. A prospective cohort consisted of 163 infants born before 32 weeks of gestation. Altogether 107 cord blood immunoproteins and 12 cytokines from peripheral blood obtained 1 and 7 days after birth were analyzed. Serial brain ultrasounds were assessed. Immunohistochemistry of a chemokine receptor from 14 autopsies was studied.
Results. Low levels of cord chemokine CCL18 (chemokine (C-C motif) ligand 18) robustly predicted the risk of IVH grade II–IV when ante- and neonatal risk factors were considered. Cord CCL18 increased from 32 weeks to term. During the first week after very preterm birth CCL18 increased as the risk of new IVH cases decreased. CCL18 receptor, CCR3, was detectable in choroid plexus, periventricular capillary endothelium, ependymal cells, and in germinal matrix.
Conclusion. Low cord blood CCL18 is an independent risk factor of IVH. CCL18 may inhibit signal transduction of its receptor in periventricular cells. Defining the function and regulation of CCL18 may help to decrease the risk of IVH.
Abbreviations | ||
BP | = | blood pressure |
CART | = | classification and regression trees |
CCL18 | = | chemokine (C-C motif) ligand 18 |
CCR3 | = | chemokine (C-C motif) receptor 3 |
CRIB | = | clinical risk index for babies |
ELGAN | = | extremely low gestational age new-born (<28 weeks) |
GA | = | gestational age |
G-CSF | = | granulocyte colony-stimulating factor |
GM | = | germinal matrix |
GM-CSF | = | granulocyte-macrophage colony-stimulating factor |
HCA | = | histologic chorioamnionitis |
IL | = | interleukin |
IVH | = | intraventricular hemorrhage |
LR | = | logistic regression |
OR | = | odds ratio |
PDA | = | patent ductus arteriosus |
RDS | = | respiratory distress syndrome |
ROC | = | receiver operating characteristic |
TNF-α | = | tumor necrosis factor-α |
VLGA | = | very low gestational age (<32 weeks) |
Key messages
Low concentration of chemokine CCL18 (chemokine (C-C motif) ligand 18) in cord blood is an independent risk factor of intraventricular hemorrhage in very preterm infants.
CCL18 is an inhibitory ligand of CCR3 receptor that was detectable at the bleeding site. Understanding the function and factors that control the ontogeny of CCL18 may contribute towards prevention of this serious disorder.
Introduction
Intraventricular hemorrhage (IVH) is a major brain injury in very preterm infants linked to subsequent neurodevelopmental disabilities (Citation1). The pathogenesis of IVH is multifactorial. Fragility of germinal matrix (GM) vasculature and deficient autoregulation of cerebral blood-flow are among the proposed factors leading to GM hemorrhage (IVH grade I) (Citation2,Citation3). Cerebral hypoxia-ischemia/reperfusion can additionally perturb cerebrovascular blood-flow injuring GM vessels (Citation2,Citation4). Hemorrhage may rupture through the ependyma into one or both lateral ventricles (IVH grade II) and cause ventricular dilatation (IVH grade III). Finally, intraventricular hemorrhage may obstruct the blood-flow in the terminal veins coursing through the GM, resulting in hemorrhagic venous infarction (IVH grade IV) (Citation1,Citation3). In most cases, IVH is evident by 72 hours after birth, and the extent of the injury is detected within the first postnatal week (Citation2). Higher grades (II–IV) of IVH are associated with poor neurodevelopmental outcome, whereas follow-up results of IVH grade I are inconclusive (Citation2,Citation5–8).
Risk factors for IVH in preterm infants include very low gestational age, vaginal birth, and intrauterine infection (Citation1). Extension of histologic chorioamnionitis (HCA) to the fetal compartment may be detrimental to the fetus. Indeed, according to several studies fetal inflammatory response syndrome, defined by umbilical vasculitis (Citation9) or increased cord serum proinflammatory cytokines (Citation10), predicted the risk of IVH (Citation11,Citation12). However, association between fetal inflammation and IVH has not been confirmed in all studies (Citation13,Citation14).
Among postnatal factors, severity of clinical illness, fluctuation of arterial blood pressure (BP), deficient autoregulation of cerebral blood-flow, hypercapnia, respiratory distress syndrome (RDS), positive pressure ventilation, pneumothorax, and coagulation disorders have been associated with IVH (Citation1,Citation5). On the other hand, intrauterine growth restriction and antenatal corticosteroids have been identified to be protective against IVH (Citation15,Citation16).
Besides structural immaturity and functional deficits in the cerebral hemodynamics, cytokines, hormones, and growth factors may influence defense against IVH (Citation17). Specific receptors and their ligands appear to influence normal brain development and neuropathology depending on the maturity and environmental stresses (Citation18). To that end, the aim of the current study was to determine whether cord serum immunoproteins would predict risk of IVH among a cohort of very preterm infants. In addition, brain tissue was investigated to localize a corresponding specific signaling receptor in periventricular cells. Here we describe a novel chemokine restricted to primates with potential of influencing the risk of IVH.
Materials and methods
Study population
The study population consisted of infants born alive before 32 weeks of gestation at Oulu University Hospital from November 1998 to November 2002. The data set of the prospective cohort has been previously described (Citation19). The study protocol was approved by the ethics committee of Oulu University Hospital. Written informed consent was obtained from the parents.
Very low gestational age (VLGA, <32 weeks) infants who died after birth (n = 18) or had serious congenital disease or malformation syndrome (n = 0) were excluded. Additionally, infants were excluded if the umbilical cord blood sample was not available (n = 42), parents refused to participate in the study (n = 6), or no follow-up data were available (n = 3). A total of 163 infants out of 232 were enrolled. Gestational age (GA) was confirmed by ultrasound before 20 weeks of gestation. In addition, cord blood was analyzed from 33 term-born infants. The sera from these low-risk infants were obtained without identification of the individuals or obtaining a consent. Supplementary Table I lists the prospectively collected antenatal and early neonatal data selected for the analysis of the factors influencing the risk of IVH.
Brain imaging
Cranial ultrasound screening was performed using HDI 5000 (Advanced Technology Laboratories Ultrasound, Botwell, WA) with a curved-array 5–8 MHz transducer. According to the protocol, the infants underwent serial brain ultrasound assessments at the following ages: 1–3 days, 1, 2, and 4 weeks, and thereafter every 4 weeks until discharge or at term. Examinations were performed by a pediatric radiologist, and the results were reviewed afterwards by a research radiologist (M.P.) without knowledge of the clinical data. Intraventricular hemorrhage was classified using standard criteria (Citation3), and the most severe grade was included in the present analysis. Early IVH was defined as IVH diagnosed within 3 days of life.
Analysis of immunoproteins
Cord blood. For measurement of the 107 proteins presented in Supplementary Table II, cord serum was separated by centrifugation and stored at −70°C. Concentrations of the cytokines were analyzed using antibody-based protein microarrays with DNA amplification (Citation20) and expressed as fluorescence units (FU).
Blood sample after birth. Two peripheral samples of arterial blood were collected on day 1 and 7 after birth. Ethylenediaminetetraacetic acid (EDTA) plasma specimens were separated and stored at −70°C. The analysis of the following cytokines was performed using the Cytometric Bead Array Kit (BD Biosciences, San Diego, CA): interleukin (IL)-1β, IL-2, IL-3, IL-4, IL-6, IL-8, IL-10, IL-12p70, eotaxin, granulocyte colony-stimulating factor (G-CSF), granulocyte-macrophage colony-stimulating factor (GM-CSF), and tumor necrosis factor (TNF)-α (125 patients). Distinct fluorescence intensities for the specific proteins were measured with flow cytometry together with the BD™ CBA Software (BD Biosciences).
Quantitative analysis. Concentrations of chemokine (C-C motif) ligand 18 (CCL18) in cord blood and in plasma on day 1 and 7 after very preterm birth (23 patients with all available specimens) and in cord blood at term (4 specimens) were measured using Duoset ELISA (R&D Systems Inc., Minneapolis, MN) according to the manufacturer's instructions. The concentrations measured by enzyme-linked immunosorbent assay (ELISA) correlated with the values obtained by antibody-based protein microarray with DNA amplification (r = 0.704).
Placental pathology
Placentas (n = 159) were fixed in 10% neutral buffered formalin immediately after birth. The rim of the membranes was taken from the site of membrane rupture. The umbilical cord specimens were obtained from the fetal and placental sides and from midway between the sides of insertion. A full-thickness specimen of placental parenchyma was taken from midway between the umbilical cord insertion and the placental margin. For histologic examination, paraffin blocks were cut into 5-μm slices and stained with hematoxylin-eosin. Histologic chorioamnionitis was defined according to the criteria of Salafia et al. (Citation21). The placentas were evaluated by a single pathologist (R.H.) without any knowledge of clinical data.
Immunohistochemistry of the brain
Nine preterm and five term infants who died between 1998 and 2008 were included in the study to detect the cellular localization of chemokine (C-C motif) receptor 3 (CCR3). Seven of the preterm infants were born between 23 and 27 weeks of gestation and two infants between 31 and 32 weeks. The primary causes of death were IVH (n = 3), RDS (n = 2), septicemia (n = 2), severe asphyxia due to placental abruption (n = 1), and pericardium tamponade caused by hydrops fetalis (n = 1). Causes of death of the full-term infants included septicemia (n = 1) and perinatal asphyxia (n = 4).
The formalin-fixed, paraffin-embedded blocks of representative brain tissue were cut into 4-μm slices, deparaffinized, and rehydrated. The antigen retrieval was done in a microwave oven in Tris-EDTA buffer, pH 9. Endogenous peroxidase activity was inhibited with peroxidase-blocking solution (DAKO, Glostrup, Denmark). The sections were incubated with 1:100 dilution of rabbit anti-human CCR3 polyclonal antibody (AHP1010, manufactured by AbD Serotec, Dusseldorf, Germany). CCR3 detection was done using the DakoCytomation kit (DAKO), and the samples were stained with DAB substrate (DAKO). The specificity of the antibody was confirmed by optimization of the immunohistochemical staining conditions on positive control tissues (liver and spleen).
Statistical analysis
Classification and regression trees (CART) was used as a primary evaluation to identify the major risk factor(s) for IVH grade II–IV. We used a conservative application of CART that is not affected by problems of multiple testing. The details of the test were explained previously (Citation19). Further statistical analyses were performed using the SPSS 15.0 program (SPSS Inc., Chicago, IL). Spearman's rank correlation test was used in order to find out the associations between independent variables and IVH. Receiver operating characteristic (ROC) was used to evaluate the cut-off point of the best sensitivity and specificity for the predicting variable. Categorical variables were assessed using the chi-square test or Fisher's exact test. Differences in continuous variables between two outcome groups (IVH grade II–IV versus no IVH or IVH grade I) were studied using the Mann-Whitney U test. Stepwise logistic regression (LR) was used as a secondary analysis to evaluate covariates as independent predictors for dependent variable. All tests were two-tailed. Statistical significance was set at P < 0.05.
Results
Clinical characteristics
The clinical characteristics of the study population are presented in . Differences in clinical factors between infants with IVH grade II–IV compared to infants with no IVH or IVH grade I are shown in . Among the cohort, 23 (14%) infants had IVH grade II–IV. Intraventricular hemorrhage grade II–IV was more common in extremely preterm infants (gestation <28 weeks) (14/49; 29%) compared to infants born ≥28 weeks of gestation (9/114; 8%) (P = 0.001). A total of 12 infants (52%) of the 23 who developed IVH grade II–IV did so within the first 3 days of life (early IVH).
Table I. Characteristics of the study group (n = 163).
Table II. Differences of clinical factors in univariate analyses between two outcome groups.
Chemokine (C-C motif) ligand 18 and intraventricular hemorrhage
Of the 107 cord serum proteins, chemokine CCL18 emerged as the only cytokine associating with IVH grade II–IV in CART. Infants with IVH grade II–IV had a lower median level of cord serum CCL18 compared to infants with no IVH or IVH grade I (4060 FU versus 6471 FU, P = 0.002). There was no difference in CCL18 concentration between infants without IVH and those with IVH grade I (median 6470 FU versus 6472 FU, P = 0.666). Term-born infants had a higher median level of CCL18 compared to very preterm infants (10097 FU versus 6053 FU, P < 0.0001). However, in very preterm infants gestational age did not associate with CCL18 (P = 0.667). There was no detectable association between CCL18 and HCA or funisitis.
As CCL18 expression was previously shown to be induced by GM-CSF and IL-4 in monocyte-derived dendritic cells (Citation22), the possible associations between these three cytokines were further studied. Correlations between CCL18 and IL-4 (P = 0.02, Spearman's correlation with Bonferroni correction) and between IL-4 and GM-CSF levels (P < 0.0001) were evident. Similar to CCL18, the cord serum concentrations of IL-4 and GM-CSF were lower in very preterm infants than in infants born at term (P < 0.0001). Neither these two cytokines, common proinflammatory cytokines (IL-1α, IL-1β, IL-6, IL-8, TNF-α), nor any of the other cord blood proteins analyzed showed association with IVH grade II–IV (Spearman correlation, ROC analysis), whereas CCL18 (cut-off value 4969 FU) predicted the risk of IVH grade II–IV with a specificity of 73% and a sensitivity of 74% (area under ROC-curve 0.72, P = 0.002) ().
Figure 1. Receiver operating characteristic (ROC) curve showing the cord blood concentration of CCL18 in prediction of the risk of intraventricular hemorrhage grade II–IV. Low level of CCL18 in cord blood (cut-off value 4969 fluorescence units) predicted IVH in very preterm infants with a sensitivity of 74% and a specificity of 73% (area under ROC curve 0.72, P=0.002).
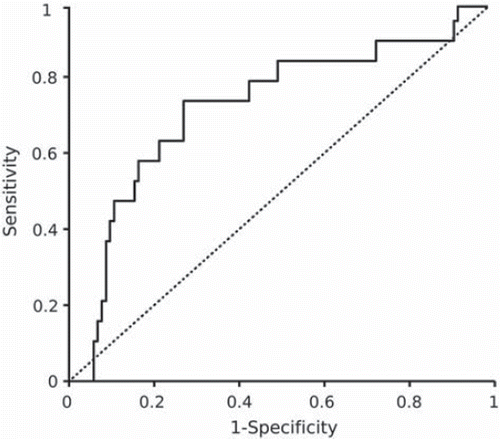
In the LR model (covariates: CCL18 <4969 FU and GA <28 wk) CCL18 independently predicted IVH grade II–IV (OR 7.6; 95% confidence interval (CI) 2.5–23.0, P < 0.0001). Gestational age had no significant effect. Further, CCL18 remained an independent risk factor for IVH grade II–IV (P = 0.001) when ante- and neonatal risk factors associating with IVH grade II–IV in univariate analyses were considered ( and ). Together with CCL18, the lowest mean BP during the first day of life additively predicted IVH grade II–IV. In an additional comparison, cases with IVH grade I were excluded. In this LR model (CCL18 <4969 FU, GA <28 wk, RDS, PDA, 5 min Apgar <7, CRIB score >4, and lowest mean BP <25 mmHg), CCL18 remained a significant, independent risk factor for IVH (OR 8.1; 95% CI 2.1–31.5, P = 0.002).
Table III. A multiple logistic regression model shows independent risk factors of IVH grade II–IV.
Subgroup analysis
Infants born before 28 weeks of gestation. In this population, HCA associated with IVH grade II–IV (P = 0.005). In the LR model (CCL18 <4969 FU, HCA, and GA <27.1 wk), HCA (OR 54.4; 95% CI 3.1–954.2, P = 0.006) and CCL18 (OR 24.0; 95% CI 2.1–270.0, P = 0.01) independently predicted IVH grade II–IV. Histologic chorioamnionitis and CCL18 remained significant, independent risk factors when other clinical variables associating with IVH grade II–IV (mode of delivery, CRIB score, the lowest mean BP, and PDA) were included in the analysis (data not shown).
Early intraventricular hemorrhage. Infants with early IVH grade II–IV had lower median levels of cord serum CCL18 (4099 FU versus 6203 FU, P = 0.026) and GM-CSF (554 FU versus 663 FU, P = 0.034) and a higher level of IL-8 (7199 FU versus 3686 FU, P = 0.042), compared to infants without early IVH or with early IVH grade I. In the LR model (CCL18 <4969 FU, GM-CSF <596 FU, IL-8 >5847 FU, GA <28 wk, and HCA), CCL18 (OR 13.9; 95% CI 2.3–83.5, P = 0.004), GM-CSF (OR 9.7; 95% CI 1.8–50.6, P = 0.007), and HCA (OR 6.1; 95% CI 1.1–32.9, P = 0.036) independently predicted early IVH grade II–IV.
Infants with HCA had a higher level of IL-8 compared to infants without HCA (6419 FU versus 2873 FU, P < 0.0001), whereas neither CCL18 (P = 0.349) nor GM-CSF (P = 0.916) associated with HCA. After including clinical variables associating with early IVH grade II–IV in the LR analysis, CCL18 remained an independent risk factor. Additional independent risk factors were GM-CSF and the lowest mean BP ().
Table IV. A multiple logistic regression model shows independent risk factors of early IVH grade II–IV.
Infants with cytokine analysis after birth. The cytokines analyzed from arterial blood of 125 VLGA infants taken on day 1 or on day 7 did not associate with IVH grade II–IV in univariate analyses. IL-6 concentrations tended to be higher in infants with IVH grade II–IV compared to infants with no IVH or IVH grade I (53.9 FU versus 24.9 FU, P = 0.05) on day 1.
Quantitative analysis. To further evaluate cord CCL18 concentration as a risk factor of IVH, the available specimens from 23 infants were submitted to quantitative analysis (). Low cord CCL18 in VLGA infants developing IVH grade II–IV was confirmed (median 4181 pg/mL versus median 5684 pg/mL in controls without grade II–IV IVH). CCL18 increased within the first 24 hours. The increase in CCL18 between day 1 and 7 was not significant. After birth CCL18 levels tended to be lower in infants with IVH grade II–IV than in those without IVH or IVH grade I. However, these differences were not significant. By the age of 7 days the levels of CCL18 in VLGA infants (median 9165 pg/mL) had approached the cord blood levels of CCL18 at term birth (median 14619 pg/mL).
Table V. Analysis of plasma concentrations of CCL18 (pg/mL) from very preterm infants with IVH grade II–IV and no IVH or IVH grade I using enzyme-linked immunosorbent assay.
Immunohistochemistry for localization of chemokine (C-C motif) receptor 3
As CCR3 is the only known CC-chemokine receptor for CCL18 (Citation23), immunohistochemistry of the brain was performed to detect the cellular localization of CCR3. We found CCR3 to be detectable already at 23 weeks of gestation. Immunopositivity was detected in the ependymal cells, the choroid plexus, the germinal matrix, the endothelium of the capillaries, and in the neurons of the hippocampus of all seven infants born before 28 weeks of gestation. The localization of CCR3 in two infants born between 31 and 32 weeks of gestation resembled those born between 23 and 27 weeks of gestation; however, immunostaining was no longer detectable in the germinal matrix ().
Figure 2. Immunohistochemical localization of CCR3 in the brain. Representative brain samples from preterm (A–F) and term (G–J) infants from different gestational weeks as indicated were stained with anti-CCR3 antibodies. Panel A shows the positive staining in the germinal matrix. Big black arrows indicate the staining in the ependymal cells, small black arrows in the endothelium of the capillaries, small open arrows in the neurons of the hippocampus, and big open arrows in the choroid plexus. Panels G and H represent samples from an infant with long-term asphyxia with remarkable capillary proliferation and an intense CCR3 staining of the endothelia. Panels I and J represent samples from an infant who died from an acute asphyxia. Original magnification in all figures is ×200.
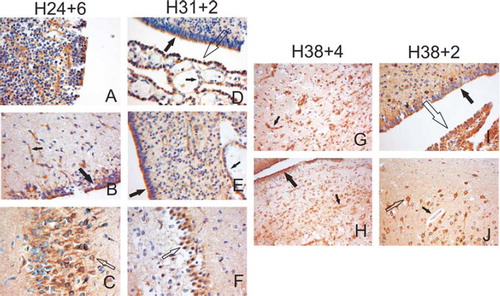
The full-term, non-IVH brain control samples showed positive staining in the choroid plexus, the ependymal cells, and in the neurons of the hippocampus (n = 5). The germinal matrix was negative at term. One infant who died of acute asphyxia had faint staining in the endothelia of the capillaries. A similar finding was seen in another infant who died of early septicemia. Interestingly, samples from brain tissue of three term infants who died several days after an episode of severe asphyxia demonstrated neuronal loss, laminar necrosis, and remarkable capillary proliferation with highly positive CCR3 staining of the endothelial cells ().
Discussion
We demonstrated that very preterm infants developing IVH shortly after birth have lower levels of cord serum CCL18 than very preterm infants without IVH or with a small germinal matrix hemorrhage. Among 107 immunoproteins analyzed, CCL18 was uniquely associated with IVH. This association was independent of known risk factors and was maintained even among the subgroups. CCL18 has not previously been associated with the risk of intraventricular hemorrhage.
Neither HCA nor funisitis showed association with IVH among the whole cohort. However, in infants born before 28 weeks of gestation, HCA did associate with IVH grade II–IV. Previously, HCA has been associated with increased risk of IVH in VLGA infants in several (Citation24–29), but not in all studies (Citation30–32). The inconsistencies in these reports may be explained by non-uniform definition of chorioamnionitis (Citation33) and by differences in treatment practices, particularly variable use of antenatal glucocorticoids which decrease the risk of IVH (Citation15,Citation16). In the present study, antenatal glucocorticoids were provided in nearly all pregnancies with very preterm births.
CCL18 belongs to the CC-chemokine family, and it is encoded in chromosome 17q11.2. Since the CCL18 gene is rapidly evolving, being exclusively identified in primates (Citation34), it may be considered as a candidate for functionality in the human brain (Citation35). CCL18 has previously been identified as pulmonary and activation-regulated chemokine (PARC) (Citation36), dendritic cell-chemokine 1 (DC-CK1) (Citation37), alternative macrophage activation-associated CC-chemokine-1 (AMAC-1) (Citation22), and macrophage inflammatory protein-4 (MIP-4) (Citation38). It participates in physiological homing of lymphocytes and primary immune responses. CCL18 may be increased in specific inflammatory conditions, such as atopic dermatitis, pneumonitis, arthritis, vasculitis, and, interestingly, in chorioamnionitis (Citation34). However, in the present study, the cord blood level of CCL18 did not associate with either HCA or funisitis.
Preterm infants with early IVH grade II–IV had a lower level of cord blood GM-CSF than preterm controls, and, together with CCL18, GM-CSF independently predicted early IVH grade II–IV. In the previous study of a different population, cord blood levels of CCL18 and GM-CSF were lower in preterm children who developed cerebral palsy than in gestational controls (Citation39). High levels of CCL18 and GM-CSF may thus be involved in protection against IVH and other forms of brain injury. In dendritic cells and in macrophages cultured with GM-CSF and IL-4, CCL18 mRNA was shown to be induced (Citation22,Citation37). In the present study of VLGA infants there was a positive correlation between CCL18 and IL-4 levels, a finding consistent with possible involvement of CCL18 in immune function and homing of T-lymphocytes. The present study revealed that the cord blood concentrations of CCL18, GM-CSF, and IL-4 increase between the 32nd week and the term. CCL18 further increases after term birth (Citation40). In the present study we additionally demonstrated a significant increase in CCL18 protein concentration within 24 hours after very preterm birth, and a non-significant increase from day 1 to day 7 (). The increase in the concentration of CCL18 shortly after very preterm birth is consistent with the proposed protective role of CCL18 against IVH, as most hemorrhages start within 24 hours after the birth, and there is a significant decrease in the rate of new IVH-events after the first day of life (Citation41).
CCL18 is not known to activate any chemokine receptor (Citation34). Instead, it serves as an antagonistic ligand for CCR3 (Citation23), a G-protein-coupled transmembrane receptor previously found in microvascular endothelial cells of adult brain (Citation42). Both neurons and glial cells of term-born, but not preterm infants have been shown to express CCR3 (Citation43). Remarkably, we found CCR3 to be detectable in periventricular capillary endothelium, choroid plexus, ependymal cells, in germinal matrix, and in the neurons of the hippocampus of preterm infants already at 23 weeks of gestation. Many other CC-chemokines, such as CCL5, CCL7, CCL8, CCL11, CCL13, CCL24, and CCL26, are also recognized by CCR3. These chemokines activate CCR3-mediated intracellular signaling (Citation44). Of the several ligands of CCR3 analyzed in the present study, only CCL18 was associated with the risk of IVH.
Previous study showed that CCL18 inhibits the chemokine-induced, CCR3-mediated eosinophil chemotaxis in HOS cells (Citation23). Considering this antagonistic function of CCL18, it is possible that a high concentration of CCL18 may block the action of agonistic ligands on CCR3, thereby inhibiting the leukocyte degranulation and proinflammatory activation (Citation45) that are known to influence the endothelial permeability and, thus, the risk of bleeding. However, this putative direct action of CCL18 on CCR3 remains to be demonstrated. Recently, the expression of CCL18 was detected in several cell types in brain biopsies from patients with traumatic brain injuries (Citation46). Potentially, CCL18 as an inhibitory ligand may locally limit the generation of specific immune responses (Citation46,Citation47).
We detected CCR3 immunopositivity in the brain capillary endothelium of very preterm infants. High CCR3 expression may be a characteristic of capillary endothelium during active angiogenesis (Citation48,Citation49). We also detected high levels of CCR3 in the proliferating capillaries of the brain in term infants after lethal asphyxia (). In addition, the expression of CCL18 has been shown to be inhibited by hypoxia in immature dendritic cells (Citation50). Ischemia-reperfusion plays a role in the pathogenesis of IVH (Citation2,Citation4). Predisposition of the periventricular area in very preterm infants to hypoxia-reperfusion- associated injuries with functional consequences, most notably spastic diplegia, is in accordance with the proposed concept of inflammatory dysregulation (Citation17,Citation35). In support of the present finding, CCL18, together with some other serum immunoproteins, was tentatively associated with cerebral palsy in a case-control study (Citation39). Fragility of germinal matrix vasculature is another proposed risk factor of IVH. Astrocyte endfeet likely provide structural integrity to germinal matrix blood vessels. Glial fibrillary acidic protein and other support structures of germinal matrix vessels may be deficient in IVH, and they are developmentally regulated (Citation51). CCL18 has been shown to increase the synthesis of an extracellular matrix protein in vitro (Citation52), and, as shown here, CCL18 has a distinct ontogeny associating with the risk of IVH. However, specific roles of CCL18 in immature periventricular tissues remain unclear.
With a limited time window for intervention, high risk, and severe long-term consequences, IVH in very preterm infants is an important therapeutic target. In the present study we aimed to define a biomarker that may have a direct role in the sequence leading to altered risk of IVH. Further studies on CCL18 could help to define new interventions that lead to further prevention of brain damage.
Table showing collated results
Download PDF (169.7 KB)Acknowledgements
We thank Mrs Riitta Vuento for technical assistance on immunohistochemistry, Mrs Riitta Vikeväinen for collection of clinical data, Jarmo Tuimala PhD for the CART analysis, and Risto Bloigu MSc for his advice on statistical analyses.
Declaration of interest: This study has been funded by the Foundation for Pediatric Research, the Alma och K. A. Snellman Foundation (H.K., T.K.), and the Sigrid Jusélius Foundation (M.H.). The authors declare no conflict of interest.
References
- McCrea HJ, Ment LR. The diagnosis, management, and postnatal prevention of intraventricular hemorrhage in the preterm neonate. Clin Perinatol. 2008;35:777–92.
- Volpe JJ. Neurology of the newborn. 5th. Philadelphia: Elsevier; 2008.
- Papile LA, Burstein J, Burstein R, Koffler H. Incidence and evolution of subependymal and intraventricular hemorrhage: a study of infants with birth weights less than 1,500 gm. J Pediatr. 1978;92:529–34.
- du Plessis AJ. Cerebrovascular injury in premature infants: current understanding and challenges for future prevention. Clin Perinatol. 2008;35:609–41.
- Whitelaw A. Intraventricular haemorrhage and posthaemorrhagic hydrocephalus: pathogenesis, prevention and future interventions. Semin Neonatol. 2001;6:135–46.
- Sherlock RL, Anderson PJ, Doyle LW. Victorian Infant Collaborative Study Group. Neurodevelopmental sequelae of intraventricular haemorrhage at 8 years of age in a regional cohort of ELWB/very preterm infants. Early Hum Dev. 2005;81:909–16.
- Inder TE. Neurodevelopmental impact of low-grade intraventricular hemorrhage in very preterm infants. J Pediatr. 2006;149:152–4.
- Luu TM, Ment LR, Schneider KC, Katz KH, Allan WC, Vohr BR. Lasting effects of preterm birth and neonatal brain hemorrhage at 12 years of age. Pediatrics. 2009;123:1037–44.
- Leviton A, Paneth N, Reuss ML, Susser M, Allred EN, Dammann O, . Maternal infection, fetal inflammatory response, and brain damage in very low birth weight infants. Developmental Epidemiology Network Investigators. Pediatr Res. 1999;46:566–75.
- Gomez R, Romero R, Ghezzi F, Yoon BH, Mazor M, Berry SM. The fetal systemic inflammatory response. Am J Obstet Gynecol. 1998;179:194–202.
- Heep A, Behrendt D, Nitsch P, Fimmers R, Bartmann P, Dembinski J. Increased serum levels of interleukin 6 are associated with severe intraventricular haemorrhage in extremely premature infants. Arch Dis Child Fetal Neonatal Ed. 2003;88:F501–4.
- Tauscher MK, Berg D, Brockmann M, Seidenspinner S, Speer CP, Groneck P. Association of histologic chorioamnionitis, increased levels of cord blood cytokines, and intracerebral hemorrhage in preterm neonates. Biol Neonate. 2003;83:166–70.
- Andrews WW, Goldenberg RL, Faye-Petersen O. The Alabama Preterm Birth study: polymorphonuclear and mononuclear cell placental infiltrations, other markers of inflammation, and outcomes in 23- to 32-week preterm newborn infants. Am J Obstet Gynecol. 2006;195:803–8.
- Babnik J, Štucin-Gantar I, Kornhauser-Cerar L, Šinkovec J, Wraber B, Derganc M. Intrauterine inflammation and the onset of peri-intraventricular hemorrhage in premature infants. Biol Neonate. 2006;90:113–21.
- Kari MA, Hallman M, Eronen M, Teramo K, Virtanen M, Koivisto M, . Prenatal dexamethasone treatment in conjunction with rescue therapy of human surfactant: a randomized placebo-controlled multicenter study. Pediatrics. 1994;93:730–6.
- Heuchan AM, Evans N, Henderson Smart DJ, Simpson JM. Perinatal risk factors for major intraventricular haemorrhage in the Australian and New Zealand Neonatal Network, 1995–97. Arch Dis Child Fetal Neonatal Ed. 2002;86:F86–90.
- Dammann O, O'Shea TM. Cytokines and perinatal brain damage. Clin Perinatol. 2008;35:643–63.
- Cardona AE, Li M, Liu L, Savarin C, Ransohoff RM. Chemokines in and out of the central nervous system: much more than chemotaxis and inflammation. Leukocyte Biol. 2008;84:587–94.
- Kaukola T, Tuimala J, Herva R, Kingsmore S, Hallman M. Cord immunoproteins as predictors of respiratory outcome in preterm infants. Am J Obstet Gynecol. 2009;200:100. e1–8.
- Schweitzer B, Roberts S, Grimwade B, Shao W, Wang M, Fu Q, . Multiplexed protein profiling on microarrays by rolling-circle signal amplification. Nat Biotechnol. 2002;20:359–65.
- Salafia CM, Weigl C, Silberman L. The prevalence and distribution of acute placental inflammation in uncomplicated term pregnancies. Obstet Gynecol. 1989;73:383–9.
- Kodelja V, Müller C, Politz O, Hakij N, Orfanos CE, Goerdt S. Alternative macrophage activation-associated CC-chemokine-1, a novel structural homologue of macrophage inflammatory protein-1 with Th2-associated expression pattern. J Immunol. 1998;160:1411–8.
- Nibbs RJB, Salcedo TW, Campbell JDM, Yao XT, Li Y, Nardelli B, . CC chemokine receptor 3 antagonism by the β-chemokine macrophage inflammatory protein 4, a property strongly enhanced by an amino-terminal alanine-methionine swap. J Immunol. 2000;164:1488–97.
- Salafia CM, Minior VK, Rosenkrantz TS, Pezzullo JC, Popek EJ, Cusick W, . Maternal, placental, and neonatal associations with early germinal matrix/intraventricular hemorrhage in infants born before 32 weeks’ gestation. Am J Perinatol. 1995;12:429–36.
- Dammann O, Leviton A. Maternal intrauterine infection, cytokines, and brain damage in the preterm newborn. Pediatr Res. 1997;42:1–8.
- DiSalvo D. The correlation between placental pathology and intraventricular hemorrhage in preterm infant. The Developmental Epidemiology Network Investigators. Pediatr Res. 1998;43:15–19.
- Dexter SC, Pinar H, Malee M, Hogan J, Carpenter MW, Vohr BR. Outcome of very low birth weight infants with histopathologic chorioamnionitis. Obstet Gynecol. 2000;96:172–7.
- Vergani P, Patanè L, Doria P, Borroni C, Cappellini A, Pezzullo JC, . Risk factors for neonatal intraventricular haemorrhage in spontaneous prematurity at 32 weeks gestation or less. Placenta. 2000;21:402–7.
- De Felice C, Toti P, Laurini RN, Stumpo M, Picciolini E, Todros T, . Early neonatal brain injury in histologic chorioamnionitis. J Pediatr. 2001;138:101–4.
- Yoon BH, Romero R, Kim CJ, Jun JK, Gomez R, Choi JH, . Amniotic fluid interleukin-6: A sensitive test for antenatal diagnosis of acute inflammatory lesions of preterm placenta and prediction of perinatal morbidity. Am J Obstet Gynecol. 1995;172:960–70.
- Sarkar S, Kaplan C, Wiswell TE, Spitzer AR. Histological chorioamnionitis and the risk of early hemorrhage in infants born < or =28 weeks gestation. J Perinatol. 2005;25:749–52.
- Richardson BS, Wakim E, daSilva O, Walton J. Preterm histologic chorioamnionitis: impact on cord gas and pH values and neonatal outcome. Am J Obstet Gynecol. 2006;195:1357–65.
- Wu YW. Systematic review of chorioamnionitis and cerebral palsy. Ment Retard Dev Disabil Res Rev. 2002;8:25–9.
- Schutyser E, Richmond A, Van Damme J. Involvement of CC chemokine ligand 18 (CCL18) in normal and pathological processes. J Leukoc Biol. 2005;78:14–26.
- Rostène W, Kitabgi P, Parsadaniantz SM. Chemokines: a new class of neuromodulator? Nat Rev Neurosci. 2007;8:895–903.
- Hieshima K, Imai T, Baba M, Shoudai K, Ishizuka K, Nakagawa T, . A novel human CC chemokine PARC that is most homologous to macrophage-inflammatory protein-1/LD78 and chemotactic for T lymphocytes, but not for monocytes. J Immunol. 1997;159:1140–9.
- Adema GJ, Hartgers F, Verstraten R, de Vries E, Marland G, Menon S, . A dendritic-cell-derived C-C chemokine that preferentially attracts naive T cells. Nature. 1997;387:713–7.
- Wells TN, Peitsch MC. The chemokine information source: identification and characterization of novel chemokines using WorldWideWeb and expressed sequence tag databases. J Leukoc Biol. 1997;61:545–50.
- Kaukola T, Satyaraj E, Patel DD, Tchernev VT, Grimwade BG, Kingsmore SF, . Cerebral palsy is characterized by protein mediators in cord serum. Ann Neurol. 2004;55:186–94.
- Koga Y, Matsuzaki A, Suminoe A, Hattori H, Hara T. Expression of cytokine-associated genes in dendritic cells (DCs): comparison between adult peripheral blood- and umbilical cord blood-derived DCs by cDNA microarray. Immunol Lett. 2008;116:55–63.
- Paneth N, Pinto-Martin J, Gardiner J, Wallenstein S, Katsikiotis V, Hegyi T, . Incidence and timing of germinal matrix/intraventricular hemorrhage in low birth weight infants. Am J Epidemiol. 1993;137:1167–76.
- Berger O, Gan X, Gujuluva C, Burns AR, Sulur G, Stins M, . CXC and CC chemokine receptors on coronary and brain endothelia. Mol Med. 1999;5:795–805.
- Van Der Meer P, Goldberg SH, Fung KM, Sharer LR, González, Lavi E. Expression pattern of CXCR3, CXCR4, and CCR3 chemokine receptors in the developing human brain. J Neuropathol Exp Neurol. 2001;60:25–32.
- Elsner J, Escher SE, Forssmann U. Chemokine receptor antagonists: a novel therapeutic approach in allergic diseases. Allergy. 2004;59:1243–58.
- Fujisawa T, Kato Y, Nagase H, Atsuta J, Terada A, Iguchi K, . Chemokines induce eosinophil degranulation through CCR-3. J Allergy Clin Immunol. 2000;106:507–13.
- Chang CY, Lee YH, Leu SJ, Wang CY, Wei CP, Hung KS, . CC-chemokine ligand 18/pulmonary activation-regulated chemokine expression in the CNS with special reference to traumatic brain injuries and neoplastic disorders. Neuroscience. 2010;165:1233–43.
- Vulcano M, Struyf S, Scapini P, Cassatella M, Bernasconi S, Bonecchi R, . Unique regulation of CCL18 production by maturing dendritic cells. J Immunol. 2003;170:3843–9.
- Salcedo R, Young HA, Ponce ML, Ward JM, Kleinman HK, Murphy WJ, . Eotaxin (CCL11) induces in vivo angiogenic responses by human CCR3+ endothelial cells. J Immunol. 2001;166:7571–8.
- Hillyer P, Mordelet E, Flynn G, Male D. Chemokines, chemokine receptors and adhesion molecules on different human endothelia: discriminating the tissue-specific functions that affect leukocyte migration. Clin Exp Immunol. 2003;134:431–41.
- Ricciardi A, Elia AR, Cappello P, Puppo M, Vanni C, Fardin P, . Transcriptome of hypoxic immature dendritic cells: modulation of chemokine/receptor expression. Mol Cancer Res. 2008;6:175–85.
- Ballabh P. Intraventricular hemorrhage in premature infants: Mechanism of disease. Pediatr Res. 2010;67:1–8.
- Atamas SP, Luzina IG, Choi J, Tsymbalyuk N, Carbonetti NH, Singh IS, . Pulmonary and activation-regulated chemokine stimulates collagen production in lung fibroblasts. Am J Respir Cell Mol Biol. 2003;29:743–9.