Abstract
The heart as a functional organ first appeared in bilaterians as a single peristaltic pump and evolved through arthropods, fish, amphibians, and finally mammals into a four-chambered engine controlling blood-flow within the body. The acquisition of cardiac complexity in the evolving heart was a product of gene duplication events and the co-option of novel signaling pathways to an ancestral cardiac-specific gene network. T-box factors belong to an evolutionary conserved family of transcriptional regulators with diverse roles in development. Their regulatory functions are integral in the initiation and potentiation of heart development, and mutations in these genes are associated with congenital heart defects. In this review we will discuss the evolutionary conserved cardiac regulatory functions of this family as well as their implication in disease in an aim to facilitate future gene-targeted and regenerative therapeutic remedies.
Abbreviations | ||
ASD | = | atrial septal defects |
AV | = | atrioventricular |
AVC | = | atrioventricular canal |
Baf60c | = | BRG-1/Brm associated factor subunit c |
BMP | = | bone morphogenetic protein |
CHD | = | congenital heart defects |
DGS | = | DiGeorge syndrome |
DKK1 | = | Dickkopf 1 |
Dll | = | distal-less |
Dpp | = | decapentaplegic |
EnR | = | engrailed |
FGF | = | fibroblast growth factor |
Fox | = | Forkhead box transcription factors |
Gja | = | gap junction protein alpha |
Hand | = | heart and neural crest derivative |
Has2 | = | hyaluronan synthase2 |
Hcn4 | = | hyperpolarization-activated cyclic nucleotide-gated potassium channel 4 |
Hey | = | hairy/enhancer-of-split related with YRPW motif |
HOS | = | Holt–Oram syndrome |
HrT | = | heartless |
Id2 | = | inhibitor of differentiation 2 |
Irx | = | Iroquois transcription factors |
Isl1 | = | islet 1 transcription factor |
IVS | = | interventricular septum |
KV | = | Kupffer's vesicle |
Mef | = | myocyte enhancer factor |
Mesp | = | mesoderm posterior transcription factor |
Mga | = | MAX gene associated |
Mhc | = | myosin heavy chain |
Mlc2v | = | myosin light chain 2v |
MMP | = | matrix metalloproteinase |
Msx | = | muscle segment homeobox |
Nfat | = | nuclear factor for activated T cells |
Nkx2.5 | = | NK homeodomain transcription factor |
Nppa | = | natriuretic peptide precursor type A |
Ntl | = | no tail |
PCAF | = | p300/CBP-associated factor |
Pitx | = | paired-like homeodomain transcription factor 1 |
Raldh2 | = | retinaldehyde dehydrogenase 2 |
SA | = | sinoatrial |
Sc-35 | = | splicing factor 35 |
Shox2 | = | short stature homeobox 2 |
SMAD | = | mothers against decapentaplegic |
Smpx | = | small nuclear protein, X-linked (Chisel) |
Sox | = | SRY-box-containing gene |
Srf | = | serum response factor |
TAZ | = | tafazzin |
Tbr1 | = | T-box brain gene 1 |
Tbx | = | T-box transcription factors |
TGF-b | = | transforming growth factor b |
TIP60 | = | Tat-interactive protein 60 |
UMS | = | ulnar-mammary syndrome |
VSD | = | ventricular septal defects |
Wg | = | wingless |
Xbra | = | Xenopus brachyury |
Xnr | = | Xenopus nodal homolog 2 |
Key messages
T-box factors belong to an evolutionary conserved family of transcriptional regulators that modulate a myriad of developmental events. Mutations in these genes have been correlated with congenital anomalies.
Innovative additions to the primitive cardiac pump marked the emergence of the multi-chambered heart as an adaptation to the transition from aquatic to terrestrial organisms.
An evolutionary conserved T-box network regulates the different steps in heart development.
Cardiac pathologies remain a primary cause of mortality in industrialized countries, manifesting at the embryonic as well as postnatal stages (Citation1). Congenital heart defects (CHD) comprise a multitude of diseases arising from aberrations in cardiac development. With an incidence rate of 1/100, nearly 3 out of 1,000 births require a surgical or catheter-based intervention during the first year of life (Citation2). Accordingly, developmental biologists embarked on characterizing the molecular pathways and the associated genetic networks that dictate normal heart development as a preliminary step to understanding the molecular basis of congenital and postnatal cardiac anomalies, as well as the development of appropriate therapeutic interventions (Citation1). The data obtained from gene-targeted mouse models as well as the forward and reverse genetic approaches in other model organisms (Xenopus, zebrafish, drosophila, and chick) have been integrated into a systems biology-based pipe-line that allowed the comprehensive study of the multiple steps of cardiac development and elucidated several transcription factors required to establish the molecular blue-print of cardiac design (Citation2). Members of the Nk homeobox, Gata, Hand, Mef, Nfat, Pitx, Forkhead, Iroquois, and T-box families of transcriptional regulators have been implicated in dictating cardiac-specific gene expression programs that modulate the timing, induction, and morphogenesis of cardiac structures (Citation3–5). In this review we will focus on the T-box family of transcription factors and their roles in cardiac lineage determination, patterning, growth, and chamber specification, septation, and valve formation, as well as the specialization of the conduction system. We will delineate the distribution, function, and targets of these proteins in the developing heart across diverse phylogenetic distances.
Establishing the blue-print for cardiac architecture
The circulatory system consisting of the heart, blood cells, and blood vessels constitutes one of the remarkable outputs of the lateral plate mesoderm; it is the first functional unit of the developing embryo with the heart being the first functional organ (Citation6,Citation7). In its simplest form, the drosophila heart or dorsal vessel, composed of segmental units, functions as a linear peristaltic pump. This hemolymph pumping organ displays several features shared with the primary vertebrate heart tube in regards to structure and development. In this arthropod, cardiac specification requires regulatory interactions between the trunk and dorsal mesoderm characterized by the expression of tinman and pannier, the orthologues of Nkx2-5 and Gata4, respectively. In birds and mammals, cardiac precursors arise from the splanchnic mesoderm that interacts with adjacent tissue in the lateral plate mesoderm and assemble in a crescent-shaped region termed the heart field expressing Nkx2-5, Gata4, and Tbx5 (Citation8–12). Inductive signals are required from the underlying endoderm; these include: BMP, FGF, crescent, cerberus, and DKK1 (Citation13–16). Two other heart fields have been implicated in contributing precursors for cardiac development, and these have been termed the second and caudal heart fields (Citation5,Citation17). The second heart field is situated in the pharyngeal mesoderm anterior to the first heart field; its cellular contributions give rise to the right ventricle and outflow tract (Citation5,Citation7). Cells expressing ISL1, FOXH1, HAND2, MEF2C, TBX20, and TBX1 demarcate the secondary heart field (Citation5,Citation18–23). The caudal heart field is localized laterally and cranially to the cardiac crescent and gives rise to the sinoatrial node (Citation5); this region expresses Tbx18 (Citation24), a unique molecular signature distinct from the other heart fields. Following cardiac specification, the precursors converge along the ventral mid-line, and fusion of the paired primordia takes place, giving rise to the heart tube (Citation6). Composed of myocardial and endocardial layers, the heart tube is organized along an antero-posterior axis and is structured into the outflow and inflow tracts and an embryonic ventricle (Citation5). The heart tube then elongates, and chamber specification commences with the expression of chamber-specific genes Nppa, Gja5, and Smpx (Citation25). Rightward looping establishes a switch from antero-posterior to left-right polarity, a process that requires a diversified transcriptional network and signaling pathways (Citation6). The non-chamber myocardium gives rise to the sinus venosus, outflow tract, and atrioventricular canal (AVC), contributing to the nodal components of the conduction system (Citation5). Septation and valve formation separate the chambers and ensure a unidirectional blood-flow within separated pulmonary and systemic circulations (Citation7).
Brachyury and the evolution of the T-box gene
In 1927, the discovery of a mutation that produced short truncated tails in mice sparked enthusiasm among geneticists; the cloned gene was termed brachyury, and a novel evolutionary conserved family of transcription factors emerged (Citation26). The defining hallmark of these proteins resides in the presence of a highly conserved DNA binding domain dubbed the T domain or T-box, which recognizes the consensus palindromic DNA motif TCACACCT. Numerous members of this gene family have been identified in both vertebrates and invertebrates from hydra to humans; however, they are completely absent from the plant model Arabidopsis thaliana (Citation27), suggesting an evolutionary divergence of metazoan lineages (Citation28). The Drosophila and Caenorhabditis elegans encode for 8 and 20 T-box members, respectively, and mammals have at least 18 (Citation29). Phylogenetic and pairwise alignment studies suggest that T-box genes may have expanded from duplication of a single ancestral gene by virtue of an unequal cross-over or transposition (Citation30). Studies from Amphioxus, a cephalochordate that diverged prior to the genome duplication events, have been fundamental in modeling the phylogeny and fate of T-box genes (Citation31–33) (). A duplicated T-box gene may disappear in evolutionary time as in the case of Tbx16 which is present in zebrafish and birds but absent from mammalian genomes, suggesting that the gene may have been lost during the divergence of the avian and mammalian lineages (Citation34). Gene duplication may also underlie functional redundancy as seen with Tbx5 and its novel paralogue in zebrafish, tbx5b. Finally, the expansion of the ancestral T-box genes may provide a mechanism for the evolution of novel structures such as the paired limbs, whose development is dictated by Tbx2/3/4/5 (Citation26). Based on these studies, the T-box gene family can be divided into five distinct subfamilies: T, Tbx1, 2, 6, and Tbr1.
Figure 1. Neighbor joining phylogenetic tree based on the conservation of the T-Box domain in two species (Homo sapiens and Bronchiostoma). The full alignment profile of the studied sequences was created in Phylip format (Citation31). The distance matrix was calculated using the Kimura model implemented in T-Rex software (Citation32).
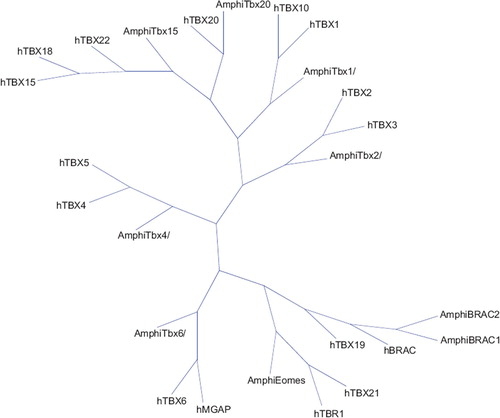
The cardiac T-box network
T-box factors belong to an evolutionary conserved family of transcription factors that modulate a myriad of developmental events. Mutations in these genes have been correlated with congenital anomalies underlying the importance of these proteins during embryonic patterning and organogenesis (Citation26). These proteins function as repressors or activators, thus implicating T-box factors in different regulatory modes within distinct molecular contexts (Citation35).
The role of this family of regulatory proteins in cardiac development emerged after the finding that mutations in T-box genes are associated with CHD, namely those involving septation, valve formation, conduction system, and outflow tract defects (Citation29). The spatio-temporal expression of T-box genes in the cardiogenic region modulates key steps in the localized genesis of the cardiac components. These factors play a prominent role in the recruitment of progenitor cells from the heart fields as well as in the antero-posterior and ventro-dorsal patterning of the differentiated chambers (Citation5). Tbx1/2/3/5/18/20 are expressed in an overlapping fashion () throughout the different stages of heart development in birds, mammals, fish, and amphibians (Citation5).
Figure 2. Expression of T-box proteins in the mammalian multi-chambered heart. TBX20 is expressed throughout the heart (gray), whereas TBX5 (blue) is expressed in in both atria (RA and LA) and ventricles (RV and LV) with a gradient pattern in the ventricles from left (highest) to right (lowest). TBX2 and TBX3 (red) are both expressed in the outflow tract (OFT), the atrioventricular (canal), and the inflow tract (IFT). TBX1 is restricted to the upper arterial trunk, whereas TBX18 is expressed in the sinus horns and node (green).
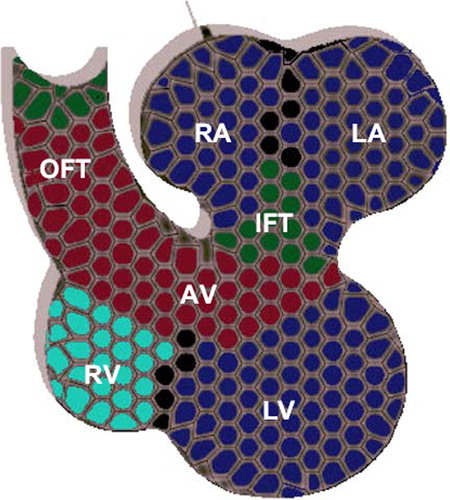
T-box genes in the specification of cardiac progenitors
Cardiac precursor cells are derived from mesodermal contributions termed the first, second, and caudal heart fields. These cells transiently express the early cardiac markers Mesp1/2 and are important for the formation of the lateral plate mesoderm (Citation36,Citation37). T-box genes are important in determining cardiac fate across different species in evolutionary time. The use of drosophila genetics has been instrumental in delineating the hierarchal transcriptional network that programs the cardiac fate mainly due to a lack of functional redundancies (Citation7). Cardiac induction requires Dpp (TGFβ) and Wg signaling derived from the dorsal ectoderm. The Tbx6 orthologues Dorsocross1-3 function in parallel with the Nkx2-5 orthologue, tinman, to regulate pannier, a Gata4 orthologue. These interactions are important in cardioblast specification; Dorsocross genes are then re-expressed in a subset of tinman2 cardioblasts (Citation38) which develop into the inflow valves (ostiae) (Citation39). Another drosophila T-box gene, Neuromancer(H15), is a Tbx20 orthologue expressed in cardiac progenitor cells (Citation29). Neuromancer appears to be downstream to Dorsocross and regulates tinman expression in the developing cardioblasts (Citation39). It is worth noting that Drosophila lacks a Tbx5 orthologue, which may indicate an evolutionary role for Tbx5 associated with an increased cardiac complexity (Citation29). In the simple chordate Ciona intestinalis, the Tbx6 orthologue Tbx6c drives the expression of Mesp which is integral for early chordate mesodermal commitment for heart development (Citation40). In birds, Tbx2/3/5/20 are expressed in the early primordia, and Tbx5/12/20 are similarly expressed throughout the cardiac crescent of murine embryos (Citation29,Citation41). In Xenopus, the use of the dominant negative fusion protein TBX5EnR blocks heart formation, thus implicating T-box genes in early cardiac development (Citation29,Citation42). SOX7 and SOX18 were demonstrated to act indirectly through Xnr2 to induce mesodermal expression of the T-box eumesodermin initiating cardiogenesis in Xenopus (Citation43). Other markers of cardiac lineage specification include Nkx2-5 and Gata4, whose expression appears to be conserved as well across species () (Citation37).
Figure 3. T-box proteins are involved in a complex network of interactions during heart development. (A) Combinatorial interactions of T-box proteins with a number of transcriptional factors function to regulate primary and secondary heart field identities early on in cardiac development. (B) Cardiac specification is tightly modulated and involves different T-box proteins that act in concert to direct distinct regulatory networks promoting proper cardiac architecture. Aberrations in these networks have been linked to congenital cardiac defects. See text for more details. Red arrows represent inductive stimuli. Red perpendicular lines indicate inhibition whereas yellow triangles, squares and arrows indicate interactions. Positive regulatory effects are shown in green.
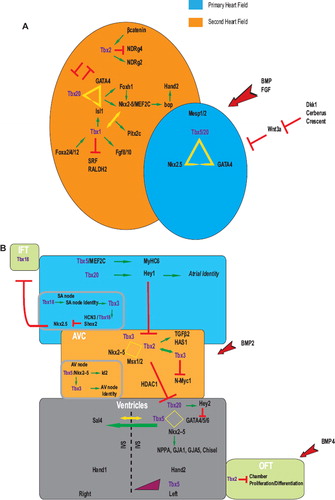
The right ventricle and outflow tract, which represent evolutionary additions to the primitive heart, are derived primarily from a population of precursors referred to as the second or anterior heart field (Citation7). Present medially to the cardiac crescent at E7.5 of mouse development, the second heart field is then positioned anterior to the first heart field in the pharyngeal mesoderm following the looping of the heart tube (Citation5). The expression program in the second heart field appears to be modulated by T-box factors (). Tbx1 is expressed in the second heart field, the pharyngeal endoderm, and the mesodermal component of the pharyngeal arches (Citation5,Citation22,Citation21). Lineage studies indicate that Tbx1+ cells contribute to the outflow tract myocardium (Citation5,Citation21). Mutations in mouse Tbx1 recapitulate those observed in human DiGeorge syndrome (MIM #188400) with outflow tract abnormalities (Citation5,Citation44,Citation45). Similarly, mutations in the Tbx1 orthologue van gogh display defective development of neural crest derivatives in zebrafish (Citation29,Citation46), indicating an evolutionary conserved mechanism in the second heart field precursor specification. Tbx1 regulates Fgf8/10 and Pitx2c; Tbx1 mouse mutants display cardiac defects similar to those observed in Fgf8 and Pitx2c, suggesting a common regulatory pathway (Citation2,Citation5,Citation47,Citation48). In fact, TBX1 interacts with NKX2-5 to activate the Pitx2c promoter (Citation48). Members of the Forkhead family of transcription factors (Foxa2, Foxc1/2) were shown to modulate Tbx1 expression in the second heart field (Citation49). Furthermore, recent data implicate Tbx1 in the center of a regulatory node that modulates the expansion of second heart field progenitors by activating Isl1, Hop, and Nkx2-6 versus differentiation through the repression of Srf (Citation50) as well as Raldh2 and its downstream targets, Gata4 and Tbx5 (Citation51).
Mice deficient for Tbx20 display outflow tract defects with underdeveloped short heart tubes, suggesting failure in the deployment of second heart field progenitors (Citation5) and implicating Tbx20 in second heart field specification. Mouse Tbx20 and its orthologues in zebrafish (hrT) and chick (cTbx20) are expressed in a subset of second heart field precursors (Citation5,Citation52,Citation53). A protein–protein interaction between TBX20, GATA4 as well as the second heart field marker, ISL1, has been implicated in the transcriptional activation of Nkx2-5 and Mef2c in the right ventricle and outflow tract; these targets were severely down-regulated in Tbx20 null embryos (Citation5,Citation54) with an ectopic up-regulation of Tbx2 (Citation55). Tbx2 misexpression in the mouse down-regulates Tbx20 and cell adhesion molecules (Alcam and N-cadherin), impairing the recruitment of the second heart field progenitors. In addition, TBX2 modulates the expression of Ndrg2/4 involved in cell growth and proliferation (Citation56). Furthermore, Tbx2/3 expression was shown to be positively regulated by a component of the Wnt signaling pathway, β-catenin (Citation57).
A third distinct heart field, the caudal heart field, has been identified laterally and cranially to the cardiac crescent, the precursors of which populate the sinus horns that gives rise to the sinoatrial (SA) node (Citation5). The caudal field displays a unique molecular signature distinct from the other heart fields; using lineage analysis, the precursors were identified to be Tbx18+, Nkx2-52, and Isl12. Tbx18+regions are associated with progenitors that contribute to the septum transversum which gives rise to the proepicardium and the mesenchyme that borders the inflow tract, specifically the sinus venosus (Citation5,Citation58,Citation59); the coronary smooth muscles and the cardiac fibroblasts are also derived from Tbx181 progenitors (Citation60). Tbx18 is also expressed on the right sinus horn of Xenopus and the epicardial tissue in chick and zebrafish, respectively (Citation61,Citation62). The formation of cardiomyocytes from epicardial cells is still controversial, however, and recent studies did show that Tbx18 is expressed in myocardial cells independently from its expression in epicardial cells (Citation63). Moreover, the latter do not contribute to the formation of Tbx181 cells in myocytes of the interventricular septum.
T-box genes in the formation and patterning of the heart tube
The two bilateral regions of heart progenitors fuse across the mid-line to form the cardiac crescent. Embryo folding instigates complex morphogenetic events that culminate in the establishment of the primitive heart tube along an antero-posterior axis (Citation37). Inactivating mutations in Gata4 (Citation64) and Mga (Citation65), a member of the Tbx6 subfamily (Box1), produce bilateral cardiac defects, namely cardia bifida, implicating these proteins in the fusion of the paired primordia. Rightward looping of the heart tube marks a transition from an antero-posterior polarity to a left-right polarity positioning the ventricles in the proper orientation; this patterning involves a critical and complex process and is regulated by retinoic acid signaling as well as a number of T-box proteins. Retinoic acid plays a pivotal role in patterning through conveying positional information along an antero-posterior axis directing atrial chamber and SA node development. RALDH2 regulates retinoic acid synthesis, and deregulated expression of this gene is associated with patterning defects. TBX1 negatively regulates Raldh2 gene expression in the second heart field, ultimately lowering retinoic acid levels across the anterior cranial extremity of the heart tube. Retinoic acid signaling restricts the expansion of Isl1+progenitors from the second heart field into the caudal posterior end of the heart tube by reducing Fgf8 expression (Citation50,Citation51,Citation66). Establishing left-right polarity requires signaling from a unique structure referred to as the node in mouse, Hensen's node in chick, Kupffer's vesicle (KV) in zebrafish, and Spemann organizer in Xenopus (Citation67). The role of T-box proteins in regulating left-right polarity stems from their importance in proper functioning of these unique structures (Citation67). In zebrafish, no tail (Ntl), an orthologue of brachyury, and Tbx16/spadetail co-operatively regulate KV cell specification (Citation68). In Xenopus, the brachyury orthologue, Xbra, is important for proper polarity as using a dominant-negative of Xbra resulted in randomization of the heart tube (Citation69); the same phenotype is observed in the brachyury-mutant mice, suggesting an evolutionary conserved mechanism (Citation70). A novel role for Tbx6 in left/right body axis determination through the regulation of Dll and nodal was also demonstrated in mice (Citation71).
Discrimination between chamber and non-chamber myocardium involves a complex, tightly regulated T-box network
The heart tube elongates in length by deploying precursor cells at the poles. These will give rise to the AVC, atria, and inflow tract myocardium at the venous caudal end, as well as the right ventricle, ventricular septum, and outflow tract myocardium at the arterial cranial pole (Citation37). Chamber differentiation then commences expanding the region and forming an embryonic left ventricle (Citation5); the right ventricle and atrial chamber specification begin shortly after (Citation37). However, the sinus venosus, AVC, inner curvature, and the outflow tract of the heart escape the chamber differentiation program and will subsequently not expand (Citation5). T-box proteins display broad and overlapping expression patterns in chamber and non-chamber myocardium; however, a complex network of transcriptional regulators and signaling pathways fine-tune the stoichiometry of T-box proteins and spatio-temporal restrictions of interacting partners to generate localized gene expression profiles and properly oriented chamber specification ().
Chamber specification
The expression program that governs myocardial chamber formation involves an up-regulation of Nmyc1, Nppa, Smpx, Gja1 and Gja5 (Citation25); these genes are differentially regulated by T-box factors in different portions of the heart tube. Tbx5/20 are expressed in a posterior-to-anterior gradient with highest localization in the inflow region (Citation37). Interactions between TBX5/20, GATA4, and NKX2-5 culminate in the activation of chamber specification and up-regulation of the chamber-specific markers, notably Nppa (Citation25,Citation26). TBX5 up-regulates and co-operatively interacts with the zinc finger protein, SALL4, to promote the expression of Gja5 and Fgf10 (Citation5,Citation72). The restricted proliferative expansion of the chambers can be attributed to the induction of Nmyc1 by locally expressed T-box proteins (TBX20) (Citation73). TBX5/20 regulate chamber diversification into atrial and ventricular identities. The caudal-high expression of TBX5 invokes sinoatrial identity (Citation5) through its evolutionary conserved interaction with MEF2C to induce the expression of myosin heavy chain (Mhc6) (Citation74); this is consistent with the timing of atrial chamber determination (Citation12). Forced expression of Tbx5 in the heart abrogates the anterior marker Mlc2v and is associated with defective ventricular morphogenesis (Citation75); on the other hand, Tbx5 null embryos display severe atrial hypoplasia (Citation76), supporting its role in atrial elaboration. Interestingly, the deletion of one allele of Tbx5 leads to a dose-dependent decrease in the expression of atrial enriched genes, like Nppa and Gja5, highlighting the dosage effect in atrial chamber formation. In mutant embryos for heartstrings, the zebrafish orthologue of Tbx5, the heart fails to loop and degenerates displaying smaller ventricles and thinner atria (Citation75), suggesting an evolutionary conserved mechanism.
As Nppa is expressed in both cardiac chambers, the regulatory T-box complexes that dictate atrial versus ventricular expression are different (Citation1). Furthermore, TBX20 was shown to up-regulate Hey1 and Hey2 in the atria and ventricles, respectively (Citation77). HEY1 induces the expression of atrial specific genes (Citation77), whereas HEY2 down-regulates Tbx5 in the ventricles and represses atrial identity, thereby inducing ventricular fate (Citation78). The atrioventricular boundary is established by restricting Tbx2 expression to the AVC myocardium as a result of atrial and ventricular repression of Tbx2 through HEY1/2 (Citation77).
The regulatory outputs of the tightly regulated T-box protein network that dictates cardiac chamber architecture, described above, has been attributed to interactions with the chromatin remodeling machinery. Interactions between TBX5 and the WW protein, TAZ, recruit the histone acetyl transferase p300/PCAF and induce an active chromatin state on the Nppa promoter (Citation79). Similar interactions has been reported between TBX5 and the BAF60C complex (Citation80) as well as the histone acetyl transferase, TIP60 (Citation5), histone methyl transferases (Citation81), and demethylases (Citation81), switching the chromatin code to an ‘on’ state and alleviating the repressive conditions in the promoters of chamber-specific genes, thereby inducing chamber differentiation. Interestingly, the role of such histone modifiers like BAF60C is even more crucial in shaping the heart in the context of any given cardiac-enriched transcription factor haploinsufficiency as is the case in Tbx5 and Nkx2-5 heterozygous mice (Citation82).
To add another level of complexity to the regulatory nodes that govern the modular localized chamber specification, one must consider the possible roles of the alternatively spliced isoforms reported for cardiac T-box proteins, specifically TBX1/3/5/20. The role of the spliced TBX3 isoforms has been investigated in breast carcinoma (Citation83,Citation84). In the context of cardiac development, the alternatively spliced TBX5 isoforms function distinctly in regulating cell growth and differentiation. The shorter truncated isoform is incapable of interacting with GATA4 and is correlated with growth arrest, whereas the long isoform is associated with growth stimulation (Citation85). Interestingly, TBX5 was also shown to interact with the splicing factor, SC35, and participates in pre-mRNA splicing (Citation86). Taken together, these data may provide another explanation for the localized and restricted functions of T-box proteins despite their overlapping expression patterns in the cardiac fields as well as provide new scope for research directions in the investigation of the complex cardiac T-box network.
T-box and non-chamber myocardium
The sinus venosus, AVC, inner curvature, and the outflow tract of the heart escape the chamber differentiation program and are commonly referred to as the non-chamber myocardium. Tbx1 is expressed in the outflow tract, and Tbx2/3 are expressed in the AVC (Citation5). The outflow tract and AVC receive inductive signals of BMP4 and BMP2, respectively, which up-regulate Tbx2/3 in those compartments () (Citation87). Interactions between TBX2/3, NKX2-5, and MSX1/2 compete with TBX5/20 to suppress the chamber proliferation (Nmyc1) and differentiation (Nppa, Cx40, and Cx43) programs (Citation37); this role seems to be evolutionary conserved in zebrafish with the orthologues tbx2a and tbx3b (Citation88). The repressive nature of Tbx2 has also been attributed to its interaction with the histone deacetylase, HDAC1 (Citation89). A dynamic property of counter-repression exists between TBX2 and TBX20, restricting the regulatory effects of each protein to their respective regions of the expanding heart tube; this property is translated in Tbx2 null mice, which ectopically express the chamber-specific markers Nppa, Gja1/5 in the primitive myocardium of AVC (Citation5,Citation90). The interaction between TBX2 and TBX20 is not, however, reflective of a direct negative regulation of each other when present together. In fact, Tbx20 regulates TBX2 expression in the primary heart field by sequestering SMAD proteins, which normally ensure the activation of Tbx2 downstream of the BMP pathway. This hallmark of BMP signaling through TBX2 is well documented in the formation of the AVC, septa, and valves.
T-box genes in septation and valve formation
Throughout the course of evolutionary time, the adapted complexity of cardiac functions to separate oxygenated from deoxygenated blood and ensure a unidirectional flow has required the addition of novel structures such as septa and valves. These innovative additions to the primitive pump marked the emergence of the multi-chambered heart as an adaptation to the transition from aquatic to terrestrial organisms (Citation7). The non-chamber myocardium of the AVC, outflow tract, and the inner curvature of the heart signals to the underlying endocardial cushions resulting in an epithelial-to-mesenchymal transition and promoting septation and valve formation (Citation5,Citation91). Endocardial cushion formation appears to be regulated by BMP-SMAD signaling directing the expression of TGFβ2 and hyaluronan synthase2 (HAS2) through TBX2 (Citation92).
Establishing an atrial and interventricular septum (IVS) involves dose-dependent T-box regulation. Tbx5 haploinsufficiency in mice and humans is associated with atrial and ventricular septal defects, supporting a role in the evolutionary formation and patterning of septa (Citation11). Recently, it has been demonstrated that a steep left-right TBX5 gradient (Box 2) is critical for IVS formation (Citation93). The regulatory networks that define this steep and correctly positioned gradient remain to be elucidated; however, several hypotheses can be speculated upon. First, this gradient can be a result of directional TBX5 protein sequestration, through LMP4 (Citation94), or degradation in the ventricles via an miRNA-regulated mechanism to establish a left-high to right-low expression. The expression of TBX5 spliced isoforms with reduced activity in a left-to-right gradient may provide an alternative mechanism responsible for the observed expression pattern. It has also been noted that Nppa expression is higher in the left ventricle and absent from the IVS (Citation95); this has been attributed to an interaction between TBX5 and SALL4 to suppress Nppa expression, forming a clear boundary at the ventricular septum. In both Sall4 and Tbx5 haploinsufficient embryos, the ventricular boundary is lost with a marked increase in Nppa in the right ventricle (Citation95). In addition, Tbx18 was shown to be expressed at the IVS (Citation60), and an interaction with GATA4 and NKX2-5 on the Nppa promoter has been reported; this association is of repressive nature as TBX18 recruits Groucho co-repressors and competes with TBX5 on the transactivation of Nppa, providing yet another mechanism for the formation of the boundary at the ventricular septum (Citation96). In regard to atrial septation, results from our lab has identified a novel endocardial pathway involving TBX5, GATA4, and NOS3 required for atrial septum formation (Citation97).
The mature heart harbors mitral and tricuspid atrioventricular valves as well as semilunar aortic valves. Transcriptional regulation of heart valve progenitors involves TWIST1, TBX20, and MSX1/2; upon maturation, additional factors are expressed including products of the Nfatc1, Sox9 and Scleraxis genes (Citation91). Experiments on chick explants have provided insight into the regulatory role of TBX20 in valve formation (Citation91). Tbx20 expression is induced by BMP2 signaling through TWIST1 to promote cell proliferation and migration in valve progenitors by up-regulating Mmp9/13 extracellular matrix remodeling enzymes and down-regulating the chondroitin sulfate proteoglycans, Aggrecan and Versican (Citation98,Citation99).
T-box genes in the development of the cardiac conduction system
The evolution of the multi-chambered heart from the simple primitive pump required the addition of a conduction system capable of synchronous contractions driving blood at high pressure. The nodal components of the mammalian conduction system are the sinoatrial (SA) node and the atrioventricular (AV) node (Citation5). The SA node has a comet-like structure with a head and tail region (Citation100), and it is derived from the myocardium at the junction between the sinus horn and right atrium (Citation5); on the other hand, the AV node is derived from the AVC. Initially during development, Tbx2/3 are expressed in the node precursors; Tbx2 is then down-regulated, and Tbx3 expression is maintained in the nodes (Citation5), thus constituting a more specific marker for the central conduction system in the heart (Citation29). No conduction system aberrations have been observed in Tbx3 null mice as well as in human TBX3 mutations of the ulnar-mammary syndrome (MIM #181450), suggesting a possible functional redundancy between Tbx2/3 (Citation101,Citation102). On the other hand, ectopic atrial Tbx3 expression induces ectopic pacemaker sites coinciding with the suppression of normal atrial expression program and the induction of Hcn4 (Citation103). Tbx18 is expressed in the sinus horns, a region that contributes to the development of the SA node (Citation5). An interplay between TBX3 and TBX18 has been demonstrated to regulate SA node formation. Tbx18 is required for the development of the head region in the SA node and mesenchymal differentiation from the sinus horn; differentiation into the sinus node myocardium is then invoked by Tbx3 which dictates the pacemaker expression program (Citation103). The chamber-specific gene Nkx2-5 represses this developmental cascade, which is alleviated by the homeodomain transcription factor Shox2 (Citation104).
Mutations in TBX5 in the human Holt–Oram syndrome (MIM #142900) as well as Tbx5 heterozygous mice are associated with cardiac conduction system disease (Citation76,Citation105). In fact, the development of the AV node was shown to be dependent on Tbx5, Nkx2-5, Id2 (Citation106), as well as Tbx3 (Citation100).
Aberrations in T-box gene dosage manifest in congenital heart defects
Cardiac T-box genes function together in concert to modulate key steps during cardiac development. These genes display overlapping dose-dependent expression with distinct regulatory outputs mediated through spatio-temporal restricted interactions with other transcription factors as well as chromatin remodeling complexes. Mutations that disrupt the function of key nodal points of this complex cardiac network underlie a collection of cardiac developmental anomalies termed congenital heart defects (CHD). The common cardiac malformations include septal defects (atrial or ventricular), truncus arteriosis and aortic arch anomalies, conduction system malformations, pulmonary stenosis, pulmonary atresia, atrioventricular canal defects, transposition of great arteries, double outlet right ventricle, as well as hypoplastic left heart syndrome (Citation107). The implication of genes encoding cardiac-enriched transcription factors in CHD was the focus of many research projects in the last decade, and altogether the results do recapitulate the haploinsufficiency phenotypes encountered in model organisms from drosophila to mice (Citation108). As for members of the T-box-encoded genes, the results have shown so far that most mutations are associated with phenotypes that include one cardiac component inside a broader syndrome that covers other organs ().
Table I. Classification of the distinct mutations associated with the cardiac T-box proteins underlying congenital heart anomalies.
TBX1 mutations are associated with DiGeorge syndrome (DGS) characterized by a variety of abnormalities including cardiac anomalies. As Tbx1 is fundamental to the second heart field lineages, its mutations confer outflow tract and conotruncal defects as well as great vessel mis-patterning (Citation5,Citation44). Mutations in TBX5 are linked to the Holt–Oram syndrome (HOS) characterized by forelimb and heart defects. The aberrant cardiac phenotype manifests in atrial as well as ventricular septal defects in addition to conduction system disease and AVC defects (Citation109). Although there is no direct link between the severity and diversity of the phenotypes observed in HOS patients with any of the mutations described so far, the gene dosage effect is reflective of the importance of Tbx5 expression. This goes in parallel with the phenotype observed in heterozygous mice carrying only one Tbx5 allele (Citation76). On the other hand, TBX20 mutations correlate with aberrant valvulogenesis and septal defects (Citation110,Citation111). These mutations, however, can lead to either a gain or a loss of function, arguing once again for an important role of gene and protein dosage in regulating different aspects of chamber formation and subsequent function of the heart (Citation110). Interestingly, TBX3 mutations are correlated with the ulnar-mammary syndrome (UMS) with rare evident cardiac defects; this may be due to a functional redundancy with TBX2. However, two case studies identified UMS patients with overt ventricular septal defects (VSD) as well as cardiac conduction disease (Citation112,Citation113).
Functionally, most of the mutations lead to either a defect in the DNA binding capacity of the protein or alters its interactions with binding partners, thus producing different phenotypes.
Conclusion
Changes in the exogenous milieu of organisms across history have induced radical modular modifications in the genetic programs of these organisms. This has translated into the appearance of novel structures and traits required for the adaptation to new contexts. Most frequently, these modifications manifest in genome duplication events that allow for new patterns of gene expression and networks of protein interactions, thereby coupling the ancient signaling components with new pathways to generate innovative structures or adaptive functions for existing structures (Citation7). In this review, we have focused on the evolutionary conservation of cardiac T-box genes across diverse phylogenetic distances and their implications in the evolution of cardiac development from the single-chambered heart of fish into the multi-chambered mammalian heart owing to the aquatic-to-terrestrial transition.
These factors govern diverse developmental processes and act through a tightly regulated network to dictate cardiac lineage specification. Mutations in T-box genes have been associated with congenital heart diseases. In fact, the association of these factors with human congenital heart defects instigated research on their respective roles in cardiac development (Citation5). As this family is evolutionary conserved, animal models of human congenital heart diseases, HOS (Citation76) and DGS (Citation114), were subsequently established providing insight into understanding the molecular basis of human heart diseases. These tools proved to be imperative in the identification of interacting partners, the mutations in which correlate with the type and severity of the underlying congenital heart disease.
Whereas some aspects of heart morphogenesis during evolution were unraveled from extensive studies of T-box gene expression and function, like the role of Tbx5 in ventricular septum formation (Citation93), others like cardiomyocytes’ exit and re-entry into the cell cycle still need to be elucidated. In fact, the capacity of myocardial regeneration and repair appear to be restricted to fish, amphibians, mollusks, and arthropods, whereas it is completely absent from warm-blooded animals (Citation7). Studies from zebrafish demonstrated an unusual cardiac regeneration capacity after acute injury; this observation was concurrent with the induction of the embryonic epicardial markers Raldh2 and Tbx18 (Citation62). In fact, Tbx18 proepicardial cells were shown to derive a myocardial lineage that contributes to the myocytes of the IVS as well as the atrial and ventricular walls (Citation60). As RALDH2 activity dictates Tbx5 expression through its product, retinoic acid, it is not unlikely that Tbx5 participates in the regeneration process as well. Therefore, the pathways that induce the conditional expression of these evolutionary conserved genes (Raldh2 and Tbx18) may represent a primordial metazoan attribute that was lost in evolutionary time. The expression of upstream or downstream regulators might be behind this loss or simply a targeted mechanism of protein inactivation. The advents of stem cell research and nuclear reprogramming will be integral in conceiving the application of the Tbx18 proepicardial cells in cardiac repair and regeneration (Citation60,Citation115).
Box 1: Dual T-box and bHLH motifs
Few transcription factors share more than one DNA binding domain that is used to recognize the cognate sites. Such proteins presumably evolved as part of the duplication process with an acquisition of a functional domain that not only will help establish a protein–protein network but also plays a much broader versatile role in gene regulation. The Mga gene which encodes a unique protein made up of a bHLH leucine zipper and a T-box domain is conserved in evolution with regard to its expression and function. This protein is expressed in numerous organs during development, including the heart. Injection of Mga-specific morpholinos into fertilized eggs of zebrafish leads to morphogenetic abnormalities in the brain, gut, and heart (Citation65). The absence of Mga orthologue in Amphioxus and C. intestinalis and the relatively high homology in the T-box domain shared with Tbx6 in these organisms argue for an evolutionary duplication process. The presence of a bHLH and T-box DNA binding domains in one protein is potentially reflective of a co-operative interaction between members of these two families of transcription factors. Indeed we have characterized a novel interaction between HAND2 and both TBX5 and TBX20 proteins (Tanos R, Nemer G, unpublished results) that could unravel a spatio-temporal function in cardiac morphogenesis.
Box 2: Tbx5 and cardiac chamber evolution
The transition from aquatic to terrestrial life demanded an evolutionary adaptation in the cardiac design to separate oxygenated from deoxygenated blood within the systemic and pulmonary circulations. The aquatic heart of the fish is composed of a single atrium connected directly to a ventricle, whereas the terrestrial heart of birds and mammals is fast-contracting and multi-chambered with two auricles and two ventricles. On the other hand, the amphibian heart consists of two auricles and a single ventricle and may depict an intermediate in the emergence of the multichambered heart. The modular invention of the right ventricle in the multi-chambered heart represents an evolutionary milestone (Citation37) and sheds light on the presence of a second anterior heart field from which the components of the outflow tract are also derived. One hypothesis suggests that precursors from the second heart field serve to expand the original single ventricular structure prior to septation and the emergence of the right ventricle (Citation7). In birds and mammals, Tbx5 expression is restricted to the precursors of the left ventricle; on the other hand, in reptilians, Tbx5 is expressed initially throughout the cardiogenic region of the looping heart and is then restricted to the left ventricle in the chelonian turtle but not the squamate anole. TBX5 expression is then established as a steep left-to-right gradient in the developing chick, murine, and turtle hearts but not the anole; this properly positioned gradient is imperative for ventricular septation and the development of the IVS (Citation93). Ectopic Tbx5 expression in the murine heart results in IVS defects and a single ventricle with left ventricular identity, whereas localized expression produces a rightward shift in the IVS (Citation116). In this context, gradient expression of Tbx5 functions in the modular invention of a new cardiac structure, the IVS, inherent in the adaptation for an aquatic-to-terrestrial transition. What determines the left-to-right gradient of Tbx5 expression remains to be addressed (see text).
Understanding the evolutionary conservation of the T-box genes and their regulated pathways will provide insight into organogenesis as well as promote the development of novel therapeutic regimens for congenital and acquired heart disease.
Acknowledgements
We are grateful to Mr Zahy AbdulSater and Mr Nehmé El-Hachem for critical review of the manuscript.
Declaration of interest: The authors state no conflict of interest and have received no payment in preparation of this manuscript.
References
- Nemer G, Nemer M. Regulation of heart development and function through combinatorial interactions of transcription factors. Ann Med. 2001;33:604–10.
- Gruber PJ. Cardiac development: new concepts. Clin Perinatol. 2005;32:845–55, vii.
- Brand T. Heart development: molecular insights into cardiac specification and early morphogenesis. Dev Biol. 2003;258: 1–19.
- Buckingham M, Meilhac S, Zaffran S. Building the mammalian heart from two sources of myocardial cells. Nat Rev Genet. 2005;6:826–35.
- Hoogaars WM, Barnett P, Moorman AF, Christoffels VM. T-box factors determine cardiac design. Cell Mol Life Sci. 2007;64:646–60.
- Gilbert SF. Lateral plate mesoderm and endoderm. Developmental biology. 6th. Sinauer Associates, Inc., Publishers, Sunderland, MA, USA.
- Olson EN. Gene regulatory networks in the evolution and development of the heart. Science. 2006;313:1922–7.
- Lints TJ, Parsons LM, Hartley L, Lyons I, Harvey RP. Nkx-2.5: a novel murine homeobox gene expressed in early heart progenitor cells and their myogenic descendants. Development. 1993;119:419–31.
- Heikinheimo M, Scandrett JM, Wilson DB. Localization of transcription factor GATA-4 to regions of the mouse embryo involved in cardiac development. Dev Biol. 1994;164:361–73.
- Molkentin JD, Kalvakolanu DV, Markham BE. Transcription factor GATA-4 regulates cardiac muscle-specific expression of the alpha-myosin heavy-chain gene. Mol Cell Biol. 1994;14:4947–57.
- Bruneau BG, Logan M, Davis N, Levi T, Tabin CJ, Seidman JG, . Chamber-specific cardiac expression of Tbx5 and heart defects in Holt-Oram syndrome. Dev Biol. 1999;211: 100–8.
- Zaffran S, Frasch M. Early signals in cardiac development. Circ Res. 2002;91:457–69.
- Schultheiss TM, Burch JB, Lassar AB. A role for bone morphogenetic proteins in the induction of cardiac myogenesis. Genes Dev. 1997;11:451–62.
- Glinka A, Wu W, Delius H, Monaghan AP, Blumenstock C, Niehrs C. Dickkopf-1 is a member of a new family of secreted proteins and functions in head induction. Nature. 1998;391:357–62.
- Lough J, Barron M, Brogley M, Sugi Y, Bolender DL, Zhu X. Combined BMP-2 and FGF-4, but neither factor alone, induces cardiogenesis in non-precardiac embryonic mesoderm. Dev Biol. 1996;178:198–202.
- Pfeffer PL, De Robertis EM, Izpisua-Belmonte JC. Crescent, a novel chick gene encoding a Frizzled-like cysteine-rich domain, is expressed in anterior regions during early embryogenesis. Int J Dev Biol. 1997;41:449–58.
- Dyer LA, Kirby ML. The role of secondary heart field in cardiac development. Dev Biol. 2009;336:137–44.
- Lin Q, Schwarz J, Bucana C, Olson EN. Control of mouse cardiac morphogenesis and myogenesis by transcription factor MEF2C. Science. 1997;276:1404–7.
- Cai CL, Liang X, Shi Y, Chu PH, Pfaff SL, Chen J, . Isl1 identifies a cardiac progenitor population that proliferates prior to differentiation and contributes a majority of cells to the heart. Dev Cell. 2003;5:877–89.
- Srivastava D, Thomas T, Lin Q, Kirby ML, Brown D, Olson EN. Regulation of cardiac mesodermal and neural crest development by the bHLH transcription factor, dHAND. Nat Genet. 1997;16:154–60.
- Xu H, Morishima M, Wylie JN, Schwartz RJ, Bruneau BG, Lindsay EA, . Tbx1 has a dual role in the morphogenesis of the cardiac outflow tract. Development. 2004;131: 3217–27.
- Chapman DL, Garvey N, Hancock S, Alexiou M, Agulnik SI, Gibson-Brown JJ, . Expression of the T-box family genes, Tbx1-Tbx5, during early mouse development. Dev Dyn. 1996;206:379–90.
- von Both I, Silvestri C, Erdemir T, Lickert H, Walls JR, Henkelman RM, . Foxh1 is essential for development of the anterior heart field. Dev Cell. 2004;7:331–45.
- Christoffels VM, Mommersteeg MT, Trowe MO, Prall OW, de Gier-de Vries C, Soufan AT, . Formation of the venous pole of the heart from an Nkx2-5-negative precursor population requires Tbx18. Circ Res. 2006;98:1555–63.
- Christoffels VM, Burch JB, Moorman AF. Architectural plan for the heart: early patterning and delineation of the chambers and the nodes. Trends Cardiovasc Med. 2004;14: 301–7.
- Naiche LA, Harrelson Z, Kelly RG, Papaioannou VE. T-box genes in vertebrate development. Annu Rev Genet. 2005;39: 219–39.
- Wilson V, Conlon FL. The T-box family. Genome Biol. 2002; 3:REVIEWS3008.
- Degnan BM, Vervoort M, Larroux C, Richards GS. Early evolution of metazoan transcription factors. Curr Opin Genet Dev. 2009;19:591–9.
- Plageman TF Jr, Yutzey KE. T-box genes and heart development: putting the “T” in heart. Dev Dyn. 2005;232:11–20.
- Agulnik SI, Garvey N, Hancock S, Ruvinsky I, Chapman DL, Agulnik I, . Evolution of mouse T-box genes by tandem duplication and cluster dispersion. Genetics. 1996; 144:249–54.
- Felsenstein J. Evolutionary trees from DNA sequences: a maximum likelihood approach. J Mol Evol. 1981;17:368–76.
- Minguillon C, Gibson-Brown JJ, Logan MP. Tbx4/5 gene duplication and the origin of vertebrate paired appendages. Proc Natl Acad Sci U S A. 2009;106:21726–30.
- Makarenkov V. T-REX: reconstructing and visualizing phylogenetic trees and reticulation networks. Bioinformatics. 2001;17:664–8.
- Lardelli M. The evolutionary relationships of zebrafish genes tbx6, tbx16/spadetail and mga. Dev Genes Evol. 2003;213: 519–22.
- Smith J. T-box genes: what they do and how they do it. Trends Genet. 1999;15:154–8.
- Kitajima S, Takagi A, Inoue T, Saga Y. MesP1 and MesP2 are essential for the development of cardiac mesoderm. Development. 2000;127:3215–26.
- Boogerd CJ, Moorman AF, Barnett P. Protein interactions at the heart of cardiac chamber formation. Ann Anat. 2009;191:505–17.
- Zaffran S, Reim I, Qian L, Lo PC, Bodmer R, Frasch M. Cardioblast-intrinsic Tinman activity controls proper diversification and differentiation of myocardial cells in Drosophila. Development. 2006;133:4073–83.
- Reim I, Frasch M. The Dorsocross T-box genes are key components of the regulatory network controlling early cardiogenesis in Drosophila. Development. 2005;132: 4911–25.
- Davidson B, Shi W, Levine M. Uncoupling heart cell specification and migration in the simple chordate Ciona intestinalis. Development. 2005;132:4811–8.
- Carson CT, Kinzler ER, Parr BA. Tbx12, a novel T-box gene, is expressed during early stages of heart and retinal development. Mech Dev. 2000;96:137–40.
- Horb ME, Thomsen GH. Tbx5 is essential for heart development. Development. 1999;126:1739–51.
- Zhang C, Basta T, Klymkowsky MW. SOX7 and SOX18 are essential for cardiogenesis in Xenopus. Dev Dyn. 2005;234: 878–91.
- Merscher S, Funke B, Epstein JA, Heyer J, Puech A, Lu MM, . TBX1 is responsible for cardiovascular defects in velo-cardio-facial/DiGeorge syndrome. Cell. 2001;104: 619–29.
- Jerome LA, Papaioannou VE. DiGeorge syndrome phenotype in mice mutant for the T-box gene, Tbx1. Nat Genet. 2001;27:286–91.
- Piotrowski T, Ahn DG, Schilling TF, Nair S, Ruvinsky I, Geisler R, . The zebrafish van gogh mutation disrupts tbx1, which is involved in the DiGeorge deletion syndrome in humans. Development. 2003;130:5043–52.
- Frank DU, Fotheringham LK, Brewer JA, Muglia LJ, Tristani-Firouzi M, Capecchi MR, . An Fgf8 mouse mutant phenocopies human 22q11 deletion syndrome. Development. 2002;129:4591–603.
- Nowotschin S, Liao J, Gage PJ, Epstein JA, Campione M, Morrow BE. Tbx1 affects asymmetric cardiac morphogenesis by regulating Pitx2 in the secondary heart field. Development. 2006;133:1565–73.
- Maeda J, Yamagishi H, McAnally J, Yamagishi C, Srivastava D. Tbx1 is regulated by forkhead proteins in the secondary heart field. Dev Dyn. 2006;235:701–10.
- Chen L, Fulcoli FG, Tang S, Baldini A. Tbx1 regulates proliferation and differentiation of multipotent heart progenitors. Circ Res. 2009;105:842–51.
- Liao J, Aggarwal VS, Nowotschin S, Bondarev A, Lipner S, Morrow BE. Identification of downstream genetic pathways of Tbx1 in the second heart field. Dev Biol. 2008;316: 524–37.
- Iio A, Koide M, Hidaka K, Morisaki T. Expression pattern of novel chick T-box gene, Tbx20. Dev Genes Evol. 2001;211: 559–62.
- Chen JN, van Eeden FJ, Warren KS, Chin A, Nusslein-Volhard C, Haffter P, . Left-right pattern of cardiac BMP4 may drive asymmetry of the heart in zebrafish. Development. 1997;124:4373–82.
- Takeuchi JK, Mileikovskaia M, Koshiba-Takeuchi K, Heidt AB, Mori AD, Arruda EP, . Tbx20 dose-dependently regulates transcription factor networks required for mouse heart and motoneuron development. Development. 2005;132:2463–74.
- Stennard FA, Costa MW, Lai D, Biben C, Furtado MB, Solloway MJ, . Murine T-box transcription factor Tbx20 acts as a repressor during heart development, and is essential for adult heart integrity, function and adaptation. Development. 2005;132:2451–62.
- Dupays L, Kotecha S, Angst B, Mohun TJ. Tbx2 misexpression impairs deployment of second heart field derived progenitor cells to the arterial pole of the embryonic heart. Dev Biol. 2009;333:121–31.
- Lin L, Cui L, Zhou W, Dufort D, Zhang X, Cai CL, . Beta-catenin directly regulates Islet1 expression in cardiovascular progenitors and is required for multiple aspects of cardiogenesis. Proc Natl Acad Sci U S A. 2007;104: 9313–8.
- Kraus F, Haenig B, Kispert A. Cloning and expression analysis of the mouse T-box gene Tbx18. Mech Dev. 2001;100: 83–6.
- Mommersteeg MT, Dominguez JN, Wiese C, Norden J, de Gier-de Vries C, Burch JB, . The sinus venosus progenitors separate and diversify from the first and second heart fields early in development. Cardiovasc Res. 2010;87: 92–101.
- Cai CL, Martin JC, Sun Y, Cui L, Wang L, Ouyang K, . A myocardial lineage derives from Tbx18 epicardial cells. Nature. 2008;454:104–8.
- Jahr M, Schlueter J, Brand T, Manner J. Development of the proepicardium in Xenopus laevis. Dev Dyn. 2008;237: 3088–96.
- Wills AA, Holdway JE, Major RJ, Poss KD. Regulated addition of new myocardial and epicardial cells fosters homeostatic cardiac growth and maintenance in adult zebrafish. Development. 2008;135:183–92.
- Christoffels VM, Grieskamp T, Norden J, Mommersteeg MT, Rudat C, Kispert A. Tbx18 and the fate of epicardial progenitors. Nature. 2009;458:E8–9.
- Molkentin JD, Lin Q, Duncan SA, Olson EN. Requirement of the transcription factor GATA4 for heart tube formation and ventral morphogenesis. Genes Dev. 1997;11:1061–72.
- Rikin A, Evans T. The tbx/bHLH transcription factor mga regulates gata4 and organogenesis. Dev Dyn. 2010;239: 535–47.
- Sirbu IO, Zhao X, Duester G. Retinoic acid controls heart anteroposterior patterning by down-regulating Isl1 through the Fgf8 pathway. Dev Dyn. 2008;237:1627–35.
- Ryan K, Chin AJ. T-box genes and cardiac development. Birth Defects Res C Embryo Today. 2003;69:25–37.
- Amack JD, Wang X, Yost HJ. Two T-box genes play independent and cooperative roles to regulate morphogenesis of ciliated Kupffer's vesicle in zebrafish. Dev Biol. 2007;310: 196–210.
- Kitaguchi T, Mizugishi K, Hatayama M, Aruga J, Mikoshiba K. Xenopus Brachyury regulates mesodermal expression of Zic3, a gene controlling left-right asymmetry. Dev Growth Differ. 2002;44:55–61.
- King T, Beddington RS, Brown NA. The role of the brachyury gene in heart development and left-right specification in the mouse. Mech Dev. 1998;79:29–37.
- Hadjantonakis AK, Pisano E, Papaioannou VE. Tbx6 regulates left/right patterning in mouse embryos through effects on nodal cilia and perinodal signaling. PLoS One. 2008;3:e2511.
- Hiroi Y, Kudoh S, Monzen K, Ikeda Y, Yazaki Y, Nagai R, . Tbx5 associates with Nkx2-5 and synergistically promotes cardiomyocyte differentiation. Nat Genet. 2001;28: 276–80.
- Cai CL, Zhou W, Yang L, Bu L, Qyang Y, Zhang X, . T-box genes coordinate regional rates of proliferation and regional specification during cardiogenesis. Development. 2005;132:2475–87.
- Ghosh TK, Song FF, Packham EA, Buxton S, Robinson TE, Ronksley J, . Physical interaction between TBX5 and MEF2C is required for early heart development. Mol Cell Biol. 2009;29:2205–18.
- Garrity DM, Childs S, Fishman MC. The heartstrings mutation in zebrafish causes heart/fin Tbx5 deficiency syndrome. Development. 2002;129:4635–45.
- Bruneau BG, Nemer G, Schmitt JP, Charron F, Robitaille L, Caron S, . A murine model of Holt-Oram syndrome defines roles of the T-box transcription factor Tbx5 in cardiogenesis and disease. Cell. 2001;106:709–21.
- Kokubo H, Tomita-Miyagawa S, Hamada Y, Saga Y. Hesr1 and Hesr2 regulate atrioventricular boundary formation in the developing heart through the repression of Tbx2. Development. 2007;134:747–55.
- Koibuchi N, Chin MT. CHF1/Hey2 plays a pivotal role in left ventricular maturation through suppression of ectopic atrial gene expression. Circ Res. 2007;100:850–5.
- Murakami M, Nakagawa M, Olson EN, Nakagawa O. A WW domain protein TAZ is a critical coactivator for TBX5, a transcription factor implicated in Holt-Oram syndrome. Proc Natl Acad Sci U S A. 2005;102:18034–9.
- Lickert H, Takeuchi JK, von Both I, Walls JR, McAuliffe F, Adamson SL, . Baf60c is essential for function of BAF chromatin remodelling complexes in heart development. Nature. 2004;432:107–12.
- Miller SA, Huang AC, Miazgowicz MM, Brassil MM, Weinmann AS. Coordinated but physically separable interaction with H3K27-demethylase and H3K4-methyltransferase activities are required for T-box protein-mediated activation of developmental gene expression. Genes Dev. 2008;22:2980–93.
- Takeuchi JK, Lou X, Alexander JM, Sugizaki H, Delgado-Olguin P, Holloway AK, . Chromatin remodelling complex dosage modulates transcription factor function in heart development. Nat Commun. 2011;2:187.
- Fan W, Huang X, Chen C, Gray J, Huang T. TBX3 and its isoform TBX3 + 2a are functionally distinctive in inhibition of senescence and are overexpressed in a subset of breast cancer cell lines. Cancer Res. 2004;64:5132–9.
- Yarosh W, Barrientos T, Esmailpour T, Lin L, Carpenter PM, Osann K, . TBX3 is overexpressed in breast cancer and represses p14 ARF by interacting with histone deacetylases. Cancer Res. 2008;68:693–9.
- Georges R, Nemer G, Morin M, Lefebvre C, Nemer M. Distinct expression and function of alternatively spliced Tbx5 isoforms in cell growth and differentiation. Mol Cell Biol. 2008;28:4052–67.
- Fan C, Chen Q, Wang QK. Functional role of transcriptional factor TBX5 in pre-mRNA splicing and Holt-Oram syndrome via association with SC35. J Biol Chem. 2009;284: 25653–63.
- Singh R, Horsthuis T, Farin HF, Grieskamp T, Norden J, Petry M, . Tbx20 interacts with smads to confine tbx2 expression to the atrioventricular canal. Circ Res. 2009; 105:442–52.
- Ribeiro I, Kawakami Y, Buscher D, Raya A, Rodriguez-Leon J, Morita M, . Tbx2 and Tbx3 regulate the dynamics of cell proliferation during heart remodeling. PLoS One. 2007;2:e398.
- Vance KW, Carreira S, Brosch G, Goding CR. Tbx2 is overexpressed and plays an important role in maintaining proliferation and suppression of senescence in melanomas. Cancer Res. 2005;65:2260–8.
- Harrelson Z, Kelly RG, Goldin SN, Gibson-Brown JJ, Bollag RJ, Silver LM, . Tbx2 is essential for patterning the atrioventricular canal and for morphogenesis of the outflow tract during heart development. Development. 2004;131:5041–52.
- Chakraborty S, Combs MD, Yutzey KE. Transcriptional regulation of heart valve progenitor cells. Pediatr Cardiol. 2010;31:414–21.
- Shirai M, Imanaka-Yoshida K, Schneider MD, Schwartz RJ, Morisaki T. T-box 2, a mediator of Bmp-Smad signaling, induced hyaluronan synthase 2 and Tgfbeta2 expression and endocardial cushion formation. Proc Natl Acad Sci U S A. 2009;106:18604–9.
- Koshiba-Takeuchi K, Mori AD, Kaynak BL, Cebra-Thomas J, Sukonnik T, Georges RO, . Reptilian heart development and the molecular basis of cardiac chamber evolution. Nature. 2009;461:95–8.
- Camarata T, Bimber B, Kulisz A, Chew TL, Yeung J, Simon HG. LMP4 regulates Tbx5 protein subcellular localization and activity. J Cell Biol. 2006;174:339–48.
- Koshiba-Takeuchi K, Takeuchi JK, Arruda EP, Kathiriya IS, Mo R, Hui CC, . Cooperative and antagonistic interactions between Sall4 and Tbx5 pattern the mouse limb and heart. Nat Genet. 2006;38:175–83.
- Farin HF, Bussen M, Schmidt MK, Singh MK, Schuster-Gossler K, Kispert A. Transcriptional repression by the T-box proteins Tbx18 and Tbx15 depends on Groucho corepressors. J Biol Chem. 2007;282:25748–59.
- Nadeau M, Georges RO, Laforest B, Yamak A, Lefebvre C, Beauregard J, . An endocardial pathway involving Tbx5, Gata4, and Nos3 required for atrial septum formation. Proc Natl Acad Sci U S A. 2010;107:19356–61.
- Shelton EL, Yutzey KE. Tbx20 regulation of endocardial cushion cell proliferation and extracellular matrix gene expression. Dev Biol. 2007;302:376–88.
- Shelton EL, Yutzey KE. Twist1 function in endocardial cushion cell proliferation, migration, and differentiation during heart valve development. Dev Biol. 2008;317:282–95.
- McNally EM, Svensson EC. Setting the pace: Tbx3 and Tbx18 in cardiac conduction system development. Circ Res. 2009;104:285–7.
- Davenport TG, Jerome-Majewska LA, Papaioannou VE. Mammary gland, limb and yolk sac defects in mice lacking Tbx3, the gene mutated in human ulnar mammary syndrome. Development. 2003;130:2263–73.
- Hoogaars WM, Tessari A, Moorman AF, de Boer PA, Hagoort J, Soufan AT, . The transcriptional repressor Tbx3 delineates the developing central conduction system of the heart. Cardiovasc Res. 2004;62:489–99.
- Wiese C, Grieskamp T, Airik R, Mommersteeg MT, Gardiwal A, de Gier-de Vries C, . Formation of the sinus node head and differentiation of sinus node myocardium are independently regulated by Tbx18 and Tbx3. Circ Res. 2009;104:388–97.
- Espinoza-Lewis RA, Yu L, He F, Liu H, Tang R, Shi J, . Shox2 is essential for the differentiation of cardiac pacemaker cells by repressing Nkx2-5. Dev Biol. 2009;327: 376–85.
- Moskowitz IP, Pizard A, Patel VV, Bruneau BG, Kim JB, Kupershmidt S, . The T-Box transcription factor Tbx5 is required for the patterning and maturation of the murine cardiac conduction system. Development. 2004;131: 4107–16.
- Moskowitz IP, Kim JB, Moore ML, Wolf CM, Peterson MA, Shendure J, . A molecular pathway including Id2, Tbx5, and Nkx2-5 required for cardiac conduction system development. Cell. 2007;129:1365–76.
- Hoffman JI, Kaplan S. The incidence of congenital heart disease. J Am Coll Cardiol. 2002;39:1890–900.
- Nemer M. Genetic insights into normal and abnormal heart development. Cardiovasc Pathol. 2008;17:48–54.
- Mori AD, Bruneau BG. TBX5 mutations and congenital heart disease: Holt-Oram syndrome revealed. Curr Opin Cardiol. 2004;19:211–5.
- Posch MG, Gramlich M, Sunde M, Schmitt K, Lee SH, Richter S, . A gain-of-function TBX20 mutation causes congenital atrial septal defects, patent foramen ovale and cardiac valve defects. J Med Genet. 2010;47;230–5.
- Kirk EP, Sunde M, Costa MW, Rankin SA, Wolstein O, Castro ML, . Mutations in cardiac T-box factor gene TBX20 are associated with diverse cardiac pathologies, including defects of septation and valvulogenesis and cardiomyopathy. Am J Hum Genet. 2007;81:280–91.
- Linden H, Williams R, King J, Blair E, Kini U. Ulnar mammary syndrome and TBX3: expanding the phenotype. Am J Med Genet A. 2009;149A:2809–12.
- Meneghini V, Odent S, Platonova N, Egeo A, Merlo GR. Novel TBX3 mutation data in families with ulnar-mammary syndrome indicate a genotype-phenotype relationship: mutations that do not disrupt the T-domain are associated with less severe limb defects. Eur J Med Genet. 2006;49: 151–8.
- Meechan DW, Tucker ES, Maynard TM, LaMantia AS. Diminished dosage of 22q11 genes disrupts neurogenesis and cortical development in a mouse model of 22q11 deletion/DiGeorge syndrome. Proc Natl Acad Sci U S A. 2009;106: 16434–45.
- Lam JT, Moretti A, Laugwitz KL. Multipotent progenitor cells in regenerative cardiovascular medicine. Pediatr Cardiol. 2009;30:690–8.
- Takeuchi JK, Ohgi M, Koshiba-Takeuchi K, Shiratori H, Sakaki I, Ogura K, . Tbx5 specifies the left/right ventricles and ventricular septum position during cardiogenesis. Development. 2003;130:5953–64.