Abstract
Hypertension is the most common, chronic disease in the world, and there are many effective pharmacological agents available for its treatment. Despite the plethora of treatment options, data across the globe suggest that blood pressure control rates are < 50%, a fact likely influenced in part by the inability to predict the antihypertensive drug likely to be most effective for an individual patient. Pharmacogenomics in hypertension holds the promise of identifying genetic biomarkers for antihypertensive drug response, which might be used in the future in treatment selection. Research in the field is also likely to enhance our understanding of hypertension and the mechanisms by which the various drugs produce efficacy. There are several examples in the literature of genes with relatively strong data on associations of genetic polymorphisms with antihypertensive response; the data on ADRB1, CACNB2, and NEDD4L are detailed as examples. Substantial additional data in hypertension pharmacogenomics are expected to be forthcoming from recently completed genome-wide association studies. Increased collaboration among research groups will help insure successful discoveries from these large-scale studies. The next decade should clearly define the potential clinical implications of the research in hypertension pharmacogenomics that is currently in progress.
Key messages
Data from several genes provide promise for the potential importance of hypertension pharmacogenomics research.
Hypertension pharmacogenomics has the potential to lead to more targeted approaches for selection of antihypertensive therapy, and the data are also likely to add insight to our understanding of hypertension and the mechanisms by which antihypertensive drugs are efficacious.
Additional discoveries are needed, and it is expected there will be publication of substantial amounts of genome-wide association data from the hypertension pharmacogenomics community in the near future. Increased collaboration among research groups will help insure the success of these discoveries.
Hypertension is the most common chronic disease in the world, estimated to affect 1 billion individuals worldwide. It the most common cause for prescription of pharmacological therapy, and in the United States there are numerous antihypertensive drugs with an excess of 100 million prescriptions annually. There are a number of antihypertensive drug classes available, and many pharmacological agents available in each drug class, yet response rates to any given antihypertensive drug are typically in the 50% range (Citation1). Additionally, numerous lines of evidence suggest that blood pressure (BP) control rates are far from optimal. Globally, data suggest that rates of BP control are consistently < 50%, despite the majority of patients being treated with drug therapy (Citation2–6). Importantly, there are clear links between poor BP control and adverse cardiovascular outcomes. Selection of antihypertensive therapy is largely empirical, and, with the exception of age, race, and plasma renin activity (which is rarely measured clinically), there are few reliable predictors of antihypertensive efficacy (Citation7). The rates of poor BP control among treated patients are likely a combination of failure initially to select the most appropriate agent for the given patient, along with a lack of attention by the clinician to insuring BP is controlled and adjusting therapy when it is not. Collectively, these data suggest the need for additional methods to predict the most effective antihypertensive agent for an individual patient prior to initiation of therapy.
Pharmacogenomics, or the genetic/genomic determinants of drug response and adverse effects, is one tool that might be useful in helping to individualize therapy. Conceptually, the goal of hypertension pharmacogenomics is to be able to utilize genetic information, along with other pertinent clinical or demographic data, to select the antihypertensive regimen likely to provide the greatest efficacy, with the lowest risk for adverse effects.
Most studies in the hypertension pharmacogenomics literature have focused on candidate genes, with the earliest studies dating to the mid-1990s. Many of the earliest pharmacogenetics studies focused on single nucleotide polymorphisms (SNPs) within genes in the renin-angiotensin system (RAS), but none of these have been consistently replicated across studies, as concluded in a recent systematic review (Citation8). Similarly, candidate gene studies to identify genes that influence the inherited basis for BP and hypertension have been largely unsuccessful, and it was not until recent, very large genome-wide association (GWA) studies that well-replicated genetic markers for BP and hypertension have been identified (Citation9–14). The success with candidate genes has been better for hypertension pharmacogenomics, a phenomenon that is seen consistently in pharmacogenomics, where candidate gene approaches have been more successful than they have been in disease genetics.
Two of the more interesting candidate genes in hypertension pharmacogenomics have also been documented through GWA studies as blood pressure/hypertension genes. This makes their data in hypertension pharmacogenomics perhaps that much more interesting. These genes are ADRB1 and CACNB2 (Citation9,Citation12,Citation14,Citation15).
ADRB1
ADRB1 encodes the β1-adrenergic receptor, the primary protein target of all marketed β-adrenergic receptor blockers (β-blockers). ADRB1 contains two non-synonymous polymorphisms, Arg389Gly and Ser49Gly, for which there is substantial evidence for functional consequences, particularly for Arg389Gly (Citation16). Collectively the data provide clear evidence that the Arg389 form of the receptor leads to greater coupling to adenylyl cyclase, and thus greater downstream signaling in response to agonist. The Ser49Gly polymorphism is less well studied for functional effects, but data suggest the Ser49 form exhibits greater resistance to agonist-promoted down-regulation. Based on the functional data on these SNPs, they have been extensively studied for associations with response to β-blockers, with the hypotheses that the more sensitive/responsive Ser49 and Arg389 forms would be the ones associated with the greatest β-blocker efficacy. The literature on this topic has been recently reviewed (Citation16).
Consistent with the hypotheses, several studies have shown that Arg389Arg patients, or those with the Ser49Arg389 haplotype, have the greatest BP lowering with β-blockers (Citation17–19), while other studies have not observed a relationship between ADRB1 genotype and BP lowering with β-blockers (Citation19–21).
Two studies have also implicated ADRB1 polymorphisms with cardiovascular outcomes in hypertensive patients treated with β-blockers. A genetic sub-study of the INVEST trial found that carriers of the Ser49Arg389 allele were at significantly increased risk for all-cause mortality, but treatment with atenolol significantly reduced risk of this adverse event, compared to treatment with verapamil (Citation22). Outcomes did not differ between the β-blocker and calcium channel blocker (CCB) treatment arms in those who did not carry this haplotype. A case-control study from a population cohort also suggested that SNPs in ADRB1 are associated with differential outcomes based on treatment with a β-blocker versus alternative therapy (Citation23). These pharmacogenetics findings are also consistent with outcome data with β-blockers in heart failure, where Arg389Arg has been associated with better outcomes in β-blocker-treated patients (Citation16).
Overall, the well-described functional effects of the ADRB1 polymorphisms, the association in two studies suggesting ADRB1 is a BP/hypertension gene, and the data on pharmacogenetics associations with β-blockers in some studies suggest the need for larger studies and/or meta-analyses to define better the role of these polymorphisms in β-blockers’ BP lowering or reduction in adverse outcomes effects.
CACNB2
The other gene that has been well defined as a BP/hypertension gene, and for which there are also pharmacogenomics data, is CACNB2. CACNB2 encodes the β2-regulatory subunit of the L-type calcium channel, which controls the cell surface expression of the α1c subunit of the L-type calcium channel, the pore-forming subunit to which all marketed CCBs bind. CACNB2 is the only BP/hypertension gene for which there are two independent associations, with SNPs in both the 5’ and 3’ regions (Citation9,Citation12). An additional SNP, not studied in GWA studies, has been reported to be significantly associated with differential outcomes by treatment strategy. Specifically, for rs2357928, a SNP in an alternative promoter, it was shown that GG individuals had better outcomes if treated with a β-blocker than a CCB treatment strategy, while there were no differences in outcomes by treatment in A carriers () (Citation24). This effect was replicated in Hispanics. Functional studies suggested this SNP might influence protein expression, as reporter assays revealed differences in luciferase activities by genotype. The replication of the association across ethnic groups, along with the reporter assay data, suggests rs2357928 might be a functional SNP. It is in low levels of linkage disequilibrium with the two SNPs found in the BP GWA studies. The 5’ SNP from the BP GWA studies was also tested for treatment-related outcomes, and there was a modest association with this SNP also, though not as striking as with rs2357928. Additional pharmacogenomics studies are needed, but collectively, the BP GWA studies data, along with the pharmacogenomics data suggest that CACNB2 is an important gene in regulation of BP and response to antihypertensive drugs.
Figure 1. Pharmacogenetic association of rs2357928 in CACNB2 with treatment-related outcomes (all-cause death, non-fatal myocardial infarction, non-fatal stroke) in patients randomized to β-blocker (BB) versus calcium channel blocker (CCB) treatment strategy. Hazard ratios are depicted on a log scale. Reprinted with permission from Niu et al. (Citation24).
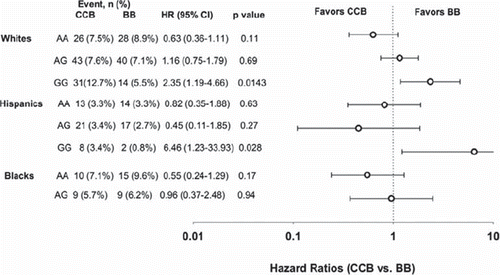
NEDD4L
Another potentially compelling pharmacogenomics example lies in NEDD4L, which encodes a regulatory protein that removes the epithelial sodium channel from the cell surface. The epithelial sodium channel represents the fine-tuning mechanism for sodium excretion in the kidney, and there are several lines of evidence for the role of NEDD4L in hypertension. These include knock-out mouse studies that indicate that mouse analog NEDD4L knock-outs have higher levels of ENaC expression, salt-sensitive hypertension, and responsiveness to amiloride, an ENaC-specific diuretic (Citation25). Numerous studies in humans, including genetic association studies, linkage analyses, and expression studies, also link NEDD4L, and specifically rs4149601, with hypertension (Citation26–29). This A > G polymorphism leads to alternative splicing with the G allele, and higher levels of BP, salt sensitivity, and decreased plasma renin activity (Citation25–29). Based on these data, one would hypothesize that G carriers should have greater BP response to thiazide diuretics, if the G allele contributes to salt-sensitive hypertension.
Data from the NORDIL trial support the potential role of this SNP in response to antihypertensive drugs. In NORDIL, patients were treated with a combination of thiazide diuretic and β-blocker, or either drug alone, compared to diltiazem. In this study, they found that G allele carriers had greater BP lowering than AA genotype patients when treated with thiazide diuretic/β-blocker, whereas there were no differences in response to diltiazem by genotype (Citation30). In the PEAR study (Citation31) we were able to test the association independently in hydrochlorothiazide (HCTZ) and atenolol (β-blocker)-treated patients and found a significant association in the HCTZ-treated patients, where there was increasing BP lowering with each G allele, but no association in the atenolol-treated patients (unpublished data). These data suggest the NORDIL findings were driven by the thiazide diuretic in the diuretic/β-blocker combination. Luo and colleagues also reported a significant association with HCTZ response by rs4149601 genotype (Citation27). Their data suggested greater BP lowering with the A allele, although there are questions about their labeling of the A versus G alleles (Citation32), so it is unclear if their finding is consistent with the NORDIL and PEAR data, and the alleles are mislabeled, or their data is opposite. Data from NORDIL further extend the potential implications for NEDD4L and rs4149601 in hypertension pharmacogenomics. Specifically, they found that patients treated in the β-blocker/thiazide arm of the trial had significantly better outcomes (related to myocardial infarction and stroke) if they were G allele carriers, while there were no differences in outcomes by genotype in diltiazem-treated patients. Collectively, these data suggest the potential importance of NEDD4L and specifically rs4149601 in salt-sensitive hypertension, response to thiazide diuretics, and treatment-related outcomes.
These pharmacogenomics examples with SNPs in ADRB1, CACNB2, and NEDD4L provide compelling data on the potential insights that might be gained from hypertension pharmacogenomics research and the potential clinical utility for such data to guide selection of an antihypertensive treatment regimen in an individual patient. These genes and their SNPs of focus have in common that they are strong biological candidates for links with the antihypertensive drug response, being involved directly as the protein target of antihypertensive drugs, or linked closely to the pharmacological targets. Further, the SNPs of focus have been well described for their functional effects in the cases of ADRB1 and NEDD4L, with initial data on the functional basis for CACNB2. These add to a long list of examples in the pharmacogenomics literature where strong biological candidates based on the pharmacology (or pharmacokinetics) of the drug have been successful targets of pharmacogenomics research. These examples in pharmacogenomics are in contrast to the candidate gene approaches in disease genetics, where successes have been minimal. This is likely because the pharmacological pathways and the proteins influencing pharmacokinetics of drugs have been well defined, in contrast to our basic understanding of pathophysiological processes of disease.
While the above examples represent compelling arguments for the potential for hypertension pharmacogenomics, more work needs to be done. First is that additional replication of the findings highlighted above is needed. This is a step that will be necessary to move these data to the level where they might be defined as clinically actionable. Sample sizes in hypertension pharmacogenomics studies remain a limitation, although it seems clear that genetic effect sizes are substantially greater in hypertension pharmacogenomics than they are for hypertension as a phenotype, or the BP phenotype. The variability in BP makes it a challenging phenotype for replication, and so high-quality BP data, e.g. from 24-hour ambulatory BP monitoring and/or numerous home BP measurements, will increase the likelihood for replication. This is an area where the hypertension pharmacogenomics data are likely stronger than the data used in hypertension genomics studies. Specifically, availability of 24-hour ambulatory or home BP data is much more common in hypertension pharmacogenomics studies, whereas the population cohorts used for hypertension genomics studies typically have limited amounts of office BP data, sometimes during treatment with antihypertensive drugs. The higher-quality BP phenotype data available in hypertension pharmacogenomics studies will increase the likelihood for replication.
Additional discoveries are also important, and moving forward these are most likely to arise from GWA studies, though the previous successes with candidate genes in pharmacogenomics will still give relevance to that approach. While only the GERA study has published GWA hypertension pharmacogenomics data to date (Citation33), such data will be emerging soon from PEAR (Citation31), GENRES (Citation34), NORDIL (Citation35), ASCOT (Citation36), and INVEST (Citation37). These studies, which focus on both the BP response phenotype (GERA, PEAR, GENRES) and antihypertensive treatment-related outcomes (NORDIL, ASCOT, INVEST), will allow generation of data for both types of antihypertensive drug response phenotypes. GenHAT is the genetic sub-study from the ALLHAT trial and, with nearly 40,000 DNA samples available, represents one of the richest potential resources for hypertension pharmacogenomics, but GWA studies are apparently not planned on this cohort (Citation38). It should be available, however, for replication efforts from the above studies. Additionally, the large genetic sub-study of EUROPA (PERGENE), a study of patients with stable coronary artery disease and not hypertension, tested the antihypertensive drug perindopril (Citation39). As such, it may also provide insights that will be useful in the identification of genetic markers for inhibitors of the renin-angiotensin system (e.g. ACE inhibitors, angiotensin receptor blockers), a group of antihypertensive drugs where there has been less success to date. Successful discoveries in hypertension genomics and elsewhere make clear that the ultimate successes in hypertension pharmacogenomics will likely lie with increased collaboration among this global group of investigators (and others that might have similar data). Efforts are underway to create a hypertension pharmacogenomics consortium to help create the mechanism for increasing such collaboration.
The key messages from the field are the following: 1) Data from several genes provide promise for the potential importance of hypertension pharmacogenomics research. 2) Hypertension pharmacogenomics has the potential to lead to more targeted approaches for selection of antihypertensive therapy, and the data are also likely to add insight to our understanding of hypertension and the mechanisms by which antihypertensive drugs are efficacious. 3) Additional discoveries are needed, and it is expected there will be publication of substantial amounts of GWA data from the hypertension pharmacogenomics research community in the near future. Increased collaboration among research groups will help insure the success of these discoveries. The next decade should clearly define the potential clinical implications of the research in hypertension pharmacogenomics that is currently in progress.
Declaration of interest: Dr. Johnson is funded by the National Institutes of Health to conduct research in hypertension pharmacogenomics. She alone is responsible for the content and writing of the paper.
References
- Materson BJ, Reda DJ, Cushman WC, Massie BM, Freis ED, Kochar MS, . Single-drug therapy for hypertension in men. A comparison of six antihypertensive agents with placebo. The Department of Veterans Affairs Cooperative Study Group on Antihypertensive Agents [see comments] [published erratum appears in N Engl J Med. 1994;330:1689]. N Engl J Med. 1993;328:914–21.
- Centers for Disease Control and Prevention (CDC). Vital signs: prevalence, treatment, and control of hypertension—United States, 1999–2002 and 2005–2008. MMWR Morb Mortal Wkly Rep. 2011;60:103–8.
- Ma J, Stafford RS. Screening, treatment, and control of hypertension in US private physician offices, 2003–2004. Hypertension. 2008;51:1275–81.
- Mori H, Ukai H, Yamamoto H, Saitou S, Hirao K, Yamauchi M, . Current status of antihypertensive prescription and associated blood pressure control in Japan. Hypertens Res. 2006;29:143–51.
- Rodriguez-Roca GC, Pallares-Carratala V, Alonso-Moreno FJ, Escobar-Cervantes C, Barrios V, Llisterri JL, . Blood pressure control and physicians’ therapeutic behavior in a very elderly Spanish hypertensive population. Hypertens Res. 2009;32:753–8.
- Thoenes M, Neuberger HR, Volpe M, Khan BV, Kirch W, Bohm M. Antihypertensive drug therapy and blood pressure control in men and women: an international perspective. J Hum Hypertens. 2010;24:336–44.
- Turner ST, Schwartz GL, Chapman AB, Beitelshees AL, Gums JG, Cooper-DeHoff RM, . Plasma renin activity predicts blood pressure responses to [beta]-blocker and thiazide diuretic as monotherapy and add-on therapy for hypertension. Am J Hypertens. 2010;23:1014–22.
- Konoshita T; Investigators tGDOCS. Do genetic variants of the renin-angiotensin system predict blood pressure response to renin-angiotensin system–blocking drugs? A systematic review of pharmacogenomics in the renin-angiotensin system. Curr Hypertens Rep. 2011;13:356–61.
- Ehret GB, Munroe PB, Rice KM, Bochud M, Johnson AD, Chasman DI, . Genetic variants in novel pathways influence blood pressure and cardiovascular disease risk. Nature. 2011;478:103–9.
- Fox ER, Young JH, Li Y, Dreisbach AW, Keating BJ, Musani SK, . Association of genetic variation with systolic and diastolic blood pressure among African Americans: the Candidate Gene Association Resource study. Hum Mol Genet. 2011;20:2273–84.
- Kato N, Takeuchi F, Tabara Y, Kelly TN, Go MJ, Sim X, . Meta-analysis of genome-wide association studies identifies common variants associated with blood pressure variation in east Asians. Nat Genet. 2011;43:531–8.
- Levy D, Ehret GB, Rice K, Verwoert GC, Launer LJ, Dehghan A, . Genome-wide association study of blood pressure and hypertension. Nat Genet. 2009;41:677–87.
- Newton-Cheh C, Johnson T, Gateva V, Tobin MD, Bochud M, Coin L, . Genome-wide association study identifies eight loci associated with blood pressure. Nat Genet. 2009;41:666–76.
- Wain LV, Verwoert GC, O'Reilly PF, Shi G, Johnson T, Johnson AD, . Genome-wide association study identifies six new loci influencing pulse pressure and mean arterial pressure. Nat Genet. 2011;43:1005–11.
- Johnson AD, Newton-Cheh C, Chasman DI, Ehret GB, Johnson T, Rose L, . Association of hypertension drug target genes with blood pressure and hypertension in 86,588 individuals. Hypertension. 2011;57:903–10.
- Johnson JA, Liggett SB. Cardiovascular pharmacogenomics of adrenergic receptor signaling: clinical implications and future directions. Clin Pharmacol Ther. 2011;89:366–78.
- Johnson JA, Zineh I, Puckett BJ, McGorray SP, Yarandi HN, Pauly DF. Beta 1-adrenergic receptor polymorphisms and antihypertensive response to metoprolol. Clin Pharmacol Ther. 2003;74:44–52.
- Liu J, Liu ZQ, Yu BN, Xu FH, Mo W, Zhou G, . beta1-Adrenergic receptor polymorphisms influence the response to metoprolol monotherapy in patients with essential hypertension. Clin Pharmacol Ther. 2006;80:23–32.
- Shin J, Johnson JA. Pharmacogenetics of beta-blockers. Pharmacotherapy. 2007;27:874–87.
- Filigheddu F, Reid JE, Troffa C, PinnaParpaglia P, Argiolas G, Testa A, . Genetic polymorphisms of the beta-adrenergic system: association with essential hypertension and response to beta-blockade. Pharmacogenomics J. 2004; 4:154–60.
- Suonsyrja T, Donner K, Hannila-Handelberg T, Fodstad H, Kontula K, Hiltunen TP. Common genetic variation of beta1- and beta2-adrenergic receptor and response to four classes of antihypertensive treatment. Pharmacogenet Genomics. 2010;20:342–5.
- Pacanowski MA, Gong Y, Cooper-Dehoff RM, Schork NJ, Shriver MD, Langaee TY, . beta-adrenergic receptor gene polymorphisms and beta-blocker treatment outcomes in hypertension. Clin Pharmacol Ther. 2008;84:715–21.
- Lemaitre RN, Heckbert SR, Sotoodehnia N, Bis JC, Smith NL, Marciante KD, . [beta]1- and [beta]2-Adrenergic receptor gene variation, [beta]-blocker use and risk of myocardial infarction and stroke. Am J Hypertens. 2008; 21:290–6.
- Niu Y, Gong Y, Langaee TY, Davis HM, Elewa H, Beitelshees AL, . Genetic variation in the beta2 subunit of the voltage-gated calcium channel and pharmacogenetic association with adverse cardiovascular outcomes in the INternational VErapamil SR-Trandolapril STudy GENEtic Substudy (INVEST-GENES). Circ Cardiovasc Genet. 2010;3:548–55.
- Shi PP, Cao XR, Sweezer EM, Kinney TS, Williams NR, Husted RF, . Salt-sensitive hypertension and cardiac hypertrophy in mice deficient in the ubiquitin ligase Nedd4 - 2. Am J Physiol Renal Physiol. 2008;295: F462–70.
- Dahlberg J, Nilsson LO, von Wowern F, Melander O. Polymorphism in NEDD4L is associated with increased salt sensitivity, reduced levels of P-renin and increased levels of Nt-proANP. PLoS One. 2007;2:e432.
- Luo F, Wang Y, Wang X, Sun K, Zhou X, Hui R. A functional variant of NEDD4L is associated with hypertension, antihypertensive response, and orthostatic hypotension. Hypertension. 2009;54:796–801.
- Russo CJ, Melista E, Cui J, DeStefano AL, Bakris GL, Manolis AJ, . Association of NEDD4L ubiquitin ligase with essential hypertension. Hypertension. 2005;46: 488–91.
- Zheng X, Morrison AC, Feingold E, Turner ST, Ferrell RE. Association between NEDD4L gene and sodium lithium countertransport. Am J Hypertens. 2011;24:145–8.
- Svensson-Farbom P, Wahlstrand B, Almgren P, Dahlberg J, Fava C, Kjeldsen S, . A functional variant of the NEDD4L gene is associated with beneficial treatment response with beta-blockers and diuretics in hypertensive patients. J Hypertens. 2011;29:388–95.
- Johnson JA, Boerwinkle E, Zineh I, Chapman AB, Bailey K, Cooper-Dehoff RM, . Pharmacogenomics of antihypertensive drugs: rationale and design of the Pharmacogenomic Evaluation of Antihypertensive Responses (PEAR) study. Am Heart J. 2009;157:442–9.
- Ishigami T, Araki N, Umemura S. Human Nedd4L rs4149601 G allele generates evolutionary new isoform I with C2 domain. Hypertension. 2010;55:e10; author reply e1.
- Turner ST, Bailey KR, Fridley BL, Chapman AB, Schwartz GL, Chai HS, . Genomic association analysis suggests chromosome 12 locus influencing antihypertensive response to thiazide diuretic. Hypertension. 2008;52:359–65.
- Hiltunen TP, Suonsyrja T, Hannila-Handelberg T, Paavonen KJ, Miettinen HE, Strandberg T, . Predictors of antihypertensive drug responses: initial data from a placebo-controlled, randomized, cross-over study with four antihypertensive drugs (The GENRES Study). Am J Hypertens. 2007;20:311–8.
- The Nordic Diltiazem Study (NORDIL). A prospective intervention trial of calcium antagonist therapy in hypertension. Blood Press. 1993;2:312–21.
- Dahlof B, Sever PS, Poulter NR, Wedel H, Beevers DG, Caulfield M, . Prevention of cardiovascular events with an antihypertensive regimen of amlodipine adding perindopril as required versus atenolol adding bendroflumethiazide as required, in the Anglo-Scandinavian Cardiac Outcomes Trial-Blood Pressure Lowering Arm (ASCOT-BPLA): a multicentre randomised controlled trial. Lancet. 2005;366: 895–906.
- Pepine CJ, Handberg EM, Cooper-DeHoff RM, Marks RG, Kowey P, Messerli FH, . A calcium antagonist vs a non-calcium antagonist hypertension treatment strategy for patients with coronary artery disease. The International Verapamil-Trandolapril Study (INVEST): a randomized controlled trial. JAMA. 2003;290:2805–16.
- Sherva R, Ford CE, Eckfeldt JH, Davis BR, Boerwinkle E, Arnett DK. Pharmacogenetic effect of the stromelysin (MMP3) polymorphism on stroke risk in relation to antihypertensive treatment. Stroke. 2011;42:330–5.
- Brugts JJ, Isaacs A, Boersma E, van Duijn CM, Uitterlinden AG, Remme W, . Genetic determinants of treatment benefit of the angiotensin-converting enzyme-inhibitor perindopril in patients with stable coronary artery disease. Eur Heart J. 2010;31:1854–64.