Abstract
The (pro)renin receptor (PRR) was initially believed to be a contributor to the pathogenesis of cardiovascular diseases via the amplification of renin- or prorenin-induced angiotensin (Ang) formation. However, a recent paradigm shift suggests a new role for PRR, separate from the renin-angiotensin system (RAS), in contributing to cellular homeostasis. Specifically, PRR is thought to be essential for vacuolar H+ -ATPase (V-ATPase) activity and acts as an adaptor between the V-ATPase and the Wnt signalling pathway. Recent PRR conditional knock-out studies have confirmed this link between V-ATPase and PRR, with deletion resulting in the accumulation of autophagic vacuoles and animal lethality. The molecular mechanism by which PRR contributes to V-ATPase activity, and whether multiple signalling pathways are affected by PRR loss, is currently unknown. Additionally, cleavage by furin at a single site within full-length PRR results in the production of a soluble form of the receptor, which is detectable in plasma. Soluble PRR is hypothesized to bind to specific ligands and receptors and mediate signal transduction pathways. Understanding the physiological function of full-length and soluble PRR will be important for establishing its role in pathology.
Key messages
The biological function of the prorenin receptor is unknown; however, recent reports point to it having an important role in V-ATPase activity and signal transduction.
Understanding the physiology of the prorenin receptor will be essential for determining its role in pathology.
The (pro)renin receptor (PRR/ATP6ap2) was first discovered and cloned by Ngyuen et al. in 2002 and was initially believed to be a contributor to the pathogenesis of cardiovascular diseases by assisting activation of the local renin-angiotensin system (RAS) (Citation1). Renin activates the RAS cascade by cleaving angiotensinogen, resulting in the production of Ang I, which is subsequently cleaved by the angiotensin-converting enzyme to produce Ang II. Current treatment of cardiovascular and renal diseases with RAS-blocking agents does not completely abolish end-organ damage, suggesting additional pathological mechanisms exist (Citation2,Citation3). Upon (pro)renin binding to the PRR, the catalytic activity of prorenin and renin is increased, resulting in increased RAS activation (Citation1). Additionally, signalling cascades are triggered resulting in the expression of inflammatory and fibrotic molecules such as VEGF and PAI-1 (Citation4,Citation5)(, left panel). Importantly, these signalling events were shown to occur independently of the generation of Ang II, leading to the hypothesis that the PRR may directly promote tissue damage, particularly in diabetes-related microvascular complications, such as diabetic nephropathy and retinopathy, where 7-fold elevations of serum prorenin can occur (Citation6). Therefore, with the discovery of PRR there was hope that pharmacological blockage of the receptor would prevent RAS-associated organ damage.
Figure 1. The (pro)renin receptor regulates the renin-angiotensin system by increasing the catalytic activity of (pro)renin and increasing angiotensin formation. (Pro)renin binding to the PRR also results in the activation of signalling cascades, resulting in the expression of inflammatory and fibrotic molecules (left panel). Additionally, PRR interacts with members of the Wnt receptor complex and contributes to the activity of the V-ATPase, thereby regulating the Wnt signal activation (right panel). See text for further details.
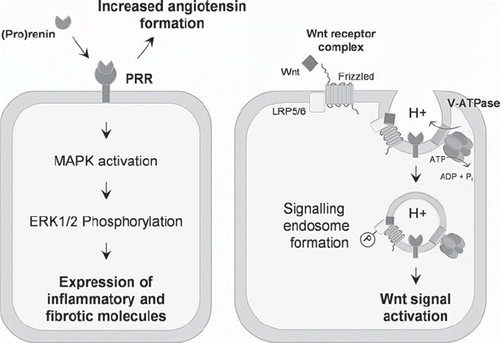
To establish if PRR has a direct role in mediating pathology, transgenic animals overexpressing PRR were generated. Rats constitutively overexpressing human PRR were found to have normal blood pressure and Ang II levels, but developed renal nephropathy (Citation7). However, this phenotype differed to rats overexpressing PRR only in smooth muscle cells. In contrast, these rats had an elevated systolic blood pressure and heart rate, with normal renal function (Citation8). An alternate approach to elucidate the role of the PRR in mediating pathology was attempted with the development of a specific PRR inhibitor. A peptide corresponding to amino acids 10 to 19 of the prorenin prosegment (handle region peptide or HRP) was developed by Ichihara et al. and hypothesized to bind to PRR, and thus block (pro)renin binding to PRR (Citation9). This peptide has been shown to be protective in several disease models including diabetic nephropathy and retinopathy (Citation10,Citation11). However, several other groups were unable to reproduce these results (Citation12,Citation13), and it has recently been shown that HRP administration is damaging to retinal neurons and glial cells (Citation11) and even counteracts beneficial treatment of direct renin inhibition with aliskiren in a rat model of hypertension (Citation14). More concerning is that a radioimmunoassay developed to measure intact HRP levels was unable to detect significant circulating amounts, suggesting that HRP is rapidly metabolized and may modulate its effects at the site of infusion, and not by inhibiting PRR at the organ of interest (Citation11). Thus, the efficacy and means by which HRP acts as a PRR inhibitor remain unclear and require further investigation.
Due to the controversy described above surrounding the PRR transgenic animal studies and those with HRP, questions have begun to arise as to what is the true biological function of PRR. Unfortunately, attempts to generate conventional complete PRR knock-out mice failed due to the inability of the embryonic stem cells to generate chimeras when injected into blastocysts (Citation15). This is in contrast to knock-out mice of other components of the RAS, which are not embryonic lethal (Citation16). PRR is expressed in all cell types including neurons, cardiomyocytes, pancreatic β-cells, podocytes, and T-cells (Citation1), suggesting it has a common and basic cellular role. In humans, the only described mutation in prr/atp6ap2 has been found to result in intellectual disability and epilepsy, suggesting an important role for PRR in neuronal development (Citation17). Together, these results suggest that PRR may have an important function in cell homeostasis, differentiation, and development.
In line with this thinking, a paradigm shift in the field over the last 12 months proposes a new role for PRR, separate from the RAS, in contributing to cellular homeostasis and signal transduction pathways. Initially, PRR was thought to have no homology to other proteins (Citation1); however, it is now apparent that the nucleotide sequence of PRR is identical to that of ATP6ap2, a gene identified as an accessory protein of the H+ vacuolar-ATPase (V-ATPase). The V-ATPase is a multi-subunit complex organized into two domains: an ATP hydrolysis domain acting like a molecular engine (V1), and a proton-pumping pore domain (V0) (Citation18). The V-ATPase is responsible for establishing and maintaining intracellular pH gradients along the secretory and endocytic pathways in all cell types. Acidification of vacuoles along these pathways is essential for many cellular functions including the processing of hormones such as insulin (Citation19), receptor endocytosis and recycling (Citation20), and membrane fusion events (Citation18).
An important mechanistic connection between PRR, V-ATPase activity, and cellular development/homeostasis was published in Science in 2010. In this study, PRR was found to interact with components of the V-ATPase and the canonical Wnt receptor-signalling complex (Citation21) (, right panel). Wnts are a family of secreted glycoproteins, and canonical Wnt signalling has been shown to be essential for many aspects of cell development and growth, with mutations in Wnt molecules resulting in carcinogenesis (Citation22,Citation23) and also heart (Citation24) and renal diseases (Citation25,Citation26). In the canonical pathway, Wnt signalling is initiated upon complex formation between soluble Wnt proteins, Frizzled and the plasma membrane receptor, LRP5/6, resulting in the downstream accumulation of β-catenin and gene transcription. Cruciat et al. showed that PRR ablation disrupts the formation of proton gradient by the V-ATPase, subsequently preventing the phosphorylation of LRP5/6 and the initiation of Wnt signal transduction. This ablation also resulted in developmental abnormalities in Xenopus embryos (Citation21).
In additional to the canonical pathway, it has also been shown that PRR is important for non-canonical Wnt signalling (also known as the planar cell polarity (PCP) pathway) (Citation27,Citation28). The non- canonical Wnt pathway shares several components with the canonical pathway, namely Frizzled and Dishevelled (Citation29). After receptor activation, the PCP pathway diverges from the non-canonical pathway and is considered to be β-catenin-independent (Citation30). The end result of PCP activation is the planar polarization of epithelial cells, important for processes such as vertebrate gastrulation (Citation31). Both studies by Buechling et al. and Hermle et al. show that PRR is essential for the PCP pathway, with deletion of PRR resulting in disturbances in PCP gene and protein expression, and malformation of wings as a result of follicle polarization defects. Additionally, these studies show that PRR interacts physically with the receptor Frizzled (Citation27,Citation28), and Hermle et al. provide some evidence that the effect of PRR deletion is mediated via a loss of V-ATPase function (Citation28). Taken together, these studies suggest that PRR has a broader cellular function than simply β-catenin signalling.
The first conditional PRR knock-out, which utilized the cre/loxP technology to circumvent the failure in generating complete PRR knock-out mice, deleted the PRR specifically in cardiomyocytes. This resulted in animal lethality after 3 weeks of birth, due to heart failure as a result of impaired ventricular function. Closer analysis of the cardiomyocytes revealed the formation and accumulation of autophagic vacuoles, which the authors conclude is a result of the disturbance of intracellular pH due to decreased expression of V0 subunits (Citation32). These results were mimicked by treatment with bafilomycin, a selective chemical inhibitor of V-ATPase. The authors therefore conclude that ablation of PRR disrupts cardiomyocyte function due to a loss of V-ATPase function.
This study was also mirrored by recent work in our lab where we examined the conditional knock-out of PRR in podocytes (Citation33). Podocyte-specific PRR knock-out mice developed a severe renal phenotype with nephrotic syndrome and acute kidney failure leading to death of the mice at the age of 2–3 weeks. We identified a decrease in vacuolar acidification in podocytes, most likely by suspended V-ATPase activity, which finally led to a complete block in protein degradation and processing by inhibiting the autophago-lysosomal system. This block in protein degradation resulted in the accumulation of toxic proteins and enhanced ER stress, ultimately leading to disintegration and massive podocyte cell death. Our results were confirmed in an identical study by Oshima et al., who also observed a severe renal phenotype as a result of podocyte dysfunction related to a loss of V-ATPase function (Citation34).
Based on the observations that PRR is essential for V-ATPase function and subsequently Wnt signalling (Citation21,Citation27,Citation28), it can be predicted that PRR is essential for Wnt-related developmental mechanisms, such as controlling the genetic programmes of embryonic development, organogenesis, and tissue homeostasis (Citation35,Citation36). This provides an explanation as to why complete PRR knock-out animals are unviable. However, comparisons of PRR conditional knock-out models to those for canonical Wnt molecules have several discrepancies. In contrast to our PRR podocyte study (Citation33), β-catenin podocyte-specific knock-out mice had no difference to wild-type littermates in body weight and kidney/body weight ratio, and urine albumin was detected up to 12 months after birth (Citation25). Additionally, β-catenin knock-out animals were protected against a disruption in podocyte integrity after experimental injury of glomerulosclerosis, indicating that pathologic activation of the Wnt pathway occurs in podocyte disorders. Also, mice with cardiomyocyte-specific ablation of β-catenin only show a mild cardiac hypertrophy which is not accompanied by a cardiac malfunction or any other cardiac deterioration up to the age of 6 months (Citation37). This study also utilized a cardiac specific αMHC promoter-driven cre, like that used by Kinouchi et al. (Citation32), excluding the possibility that any developmental related discrepancies are responsible for the phenotype differences between β-catenin and PRR cardiomyocyte knock-outs.
The comparison of these canonical Wnt-related phenotypes to PRR phenotypes suggests that knock-out of PRR results in a much more severe biological effect. This is most likely due to the involvement of PRR in V-ATPase function. The V-ATPase is an indispensable component of almost all eukaryotic cells, with its primary function being the regulation of pH along the endocytic and secretory pathways (Citation38) (although this can also be modulated by other factors such as proton diffusion, ClC chloride channels, and sodium-potassium pumps (Citation39,Citation40)).
Intracellular pH along the endocytic pathway is very tightly regulated, ranging from neutral pH in the endoplasmic reticulum, weakly acidic pH (6.8–5.9) in early endosomes, and highly acidic pH (6.0–4.9) in late endosomes/lysosomes (Citation41). In general, endocytosis functions to internalize extracellular macromolecules and fluid, and also plasma membrane proteins, receptors, and their bound ligands. It is well established that the acidification of organelles/vesicles is obligatory for plasma membrane receptor trafficking along endocytic pathways. The internalization and trafficking of plasma membrane receptors is believed to be essential for the activation and regulation of several different signal transduction pathways (Citation42,Citation43). In the case of Wnt signalling, a recent study has shown that the internalization of the Wnt-Fz-LRP6 receptor complex results in the formation of a ‘signalosome’ which is essential for Wnt signal activation (Citation44). Importantly, in this study inhibition of Wnt receptor complex internalization did not affect LRP6 phosphorylation, suggesting that both events are required for successful Wnt signal activation. This is supported by the study of Cruciat et al., who also showed that silencing of PRR and a V0 subunit of the V-ATPase prevented signalosome formation (Citation21). Another recent study has proposed that the V-ATPase acts as a bifunctional protein to establish and also to sense vesicular pH. Here, a subunit of the V0 domain was found to interact with cytosolic adaptor molecules of the endocytic transport machinery at a certain pH, and this interaction was subsequently shown to be important for the regulation of membrane receptor internalization and transport from early to late endosomes (Citation45). The molecular mechanism by which PRR is important for V-ATPase function remains unknown.
In addition to Wnt, other signalling pathways important for cell development and homeostasis, such as Notch, require V-ATPase activity (Citation46). One example is signalling by PRR itself: in cultured collecting duct cells, PRR knock-down by siRNA was mimicked by pharmacological V-ATPase inhibition and attenuated the increase in mitogen-activated protein kinase (MAPK) ERK1/2 phosphorylation induced by either renin or prorenin stimulation (Citation47), suggesting that (pro)renin signalling through the PRR is also dependent on vesicular acidification. We can thus speculate that other pathways that require plasma membrane receptor internalization for signal transduction, e.g. Notch (Citation46), LIF/STAT3 (Citation48), pre-T-cell receptor signalling (Citation49), and pathways such as Sonic-Hedgehog (Citation50,Citation51), where ligand internalization is required, may similarly be reliant on V-ATPase activity and thus PRR. These above-mentioned signalling pathways are essential for the development and proliferation of all cell types, thus providing an explanation as to why complete PRR KO embryos are unviable, and why such different cell types are severely affected by PRR loss (Citation32,Citation33).
The biological function of PRR becomes even more complex with the recent discovery by Nguyen et al. of a soluble form of PRR (Citation52). Soluble PRR (sPRR) is generated by cleavage of the full-length protein by furin, resulting in the production of a 28 kDa soluble protein. Soluble PRR has been detected in the conditioned media of various cell types (Citation52,Citation53) and in plasma (Citation52). A recent study showed that levels of sPRR are increased in the urine and renal medulla of rats infused with Ang II (Citation54), providing a prima facie case that sPRR has an important function. The biological role of sPRR is also unknown, but it has been speculated to have a role in vivo by binding to specific receptors and initiating signal transduction pathways (Citation15). Thus, it may be likely from the conditional PRR studies summarized above that part of the phenotype observed is due to the loss of sPRR. Future studies to distinguish between those cellular functions attributable to full-length PRR and those belonging to sPRR will be essential for understanding the role of PRR in physiology and pathology.
Declaration of interest: The authors report no conflicts of interest. The authors alone are responsible for the content and writing of the paper.
References
- Nguyen G, Delarue F, Burckle C, Bouzhir L, Giller T, Sraer JD. Pivotal role of the renin/prorenin receptor in angiotensin II production and cellular responses to renin. J Clin Invest. 2002;109:1417–27.
- Onozato ML, Tojo A, Goto A, Fujita T, Wilcox CS. Oxidative stress and nitric oxide synthase in rat diabetic nephropathy: effects of ACEI and ARB. Kidney Int. 2002;61:186–94.
- Parving HH, Persson F, Lewis JB, Lewis EJ, Hollenberg NK. Aliskiren combined with losartan in type 2 diabetes and nephropathy. N Engl J Med. 2008;358:2433–46.
- Satofuka S, Ichihara A, Nagai N, Koto T, Shinoda H, Noda K, . Role of nonproteolytically activated prorenin in pathologic, but not physiologic, retinal neovascularization. Invest Ophthalmol Vis Sci. 2007;48:422–9.
- Zhang J, Noble NA, Border WA, Owens RT, Huang Y. Receptor-dependent prorenin activation and induction of PAI-1 expression in vascular smooth muscle cells. Am J Physiol Endocrinol Metab. 2008;295:E810–9.
- Luetscher JA, Kraemer FB, Wilson DM, Schwartz HC, Bryer-Ash M. Increased plasma inactive renin in diabetes mellitus. A marker of microvascular complications. N Engl J Med. 1985;312:1412–7.
- Kaneshiro Y, Ichihara A, Sakoda M, Takemitsu T, Nabi AH, Uddin MN, . Slowly progressive, angiotensin II-independent glomerulosclerosis in human (pro)renin receptor-transgenic rats. J Am Soc Nephrol. 2007;18:1789–95.
- Burckle CA, Jan Danser AH, Muller DN, Garrelds IM, Gasc JM, Popova E, . Elevated blood pressure and heart rate in human renin receptor transgenic rats. Hypertension. 2006;47:552–6.
- Ichihara A, Hayashi M, Kaneshiro Y, Suzuki F, Nakagawa T, Tada Y, . Inhibition of diabetic nephropathy by a decoy peptide corresponding to the “handle” region for nonproteolytic activation of prorenin. J Clin Invest. 2004;114: 1128–35.
- Satofuka S, Ichihara A, Nagai N, Noda K, Ozawa Y, Fukamizu A, . (Pro)renin receptor-mediated signal transduction and tissue renin-angiotensin system contribute to diabetes-induced retinal inflammation. Diabetes. 2009; 58:1625–33.
- Wilkinson-Berka JL, Heine R, Tan G, Cooper ME, Hatzopoulos KM, Fletcher EL, . RILLKKMPSV influences the vasculature, neurons and glia, and (pro)renin receptor expression in the retina. Hypertension. 2010;55: 1454–60.
- Feldt S, Maschke U, Dechend R, Luft FC, Muller DN. The putative (pro)renin receptor blocker HRP fails to prevent (pro)renin signaling. J Am Soc Nephrol. 2008;19:743–8.
- Muller DN, Klanke B, Feldt S, Cordasic N, Hartner A, Schmieder RE, . (Pro)renin receptor peptide inhibitor “handle-region” peptide does not affect hypertensive nephrosclerosis in Goldblatt rats. Hypertension. 2008;51:676–81.
- van Esch JH, van Veghel R, Garrelds IM, Leijten F, Bouhuizen AM, Danser AH. Handle region peptide counteracts the beneficial effects of the renin inhibitor aliskiren in spontaneously hypertensive rats. Hypertension. 2011;57:852–8.
- Burckle C, Bader M. Prorenin and its ancient receptor. Hypertension. 2006;48:549–51.
- Reudelhuber TL. Prorenin, renin, and their receptor. Moving targets. Hypertension. 2010;55:1071–4.
- Ramser J, Abidi FE, Burckle CA, Lenski C, Toriello H, Wen G, . A unique exonic splice enhancer mutation in a family with X-linked mental retardation and epilepsy points to a novel role of the renin receptor. Hum Mol Genet. 2005;14:1019–27.
- Hinton A, Bond S, Forgac M. V-ATPase functions in normal and disease processes. Pflugers Arch. 2009;457:589–98.
- Sun-Wada GH, Toyomura T, Murata Y, Yamamoto A, Futai M, Wada Y. The a3 isoform of V-ATPase regulates insulin secretion from pancreatic beta-cells. J Cell Sci. 2006;119 (Pt 21):4531–40.
- Niehrs C, Boutros M. Trafficking, acidification, and growth factor signaling. Sci Signal. 2010;3:pe26.
- Cruciat CM, Ohkawara B, Acebron SP, Karaulanov E, Reinhard C, Ingelfinger D, . Requirement of prorenin receptor and vacuolar H+-ATPase-mediated acidification for Wnt signaling. Science. 2010;327:459–63.
- Goodwin AM, D'Amore PA. Wnt signaling in the vasculature. Angiogenesis. 2002;5:1–9.
- Reya T, Clevers H. Wnt signalling in stem cells and cancer. Nature. 2005;434:843–50.
- Bergmann MW. WNT signaling in adult cardiac hypertrophy and remodeling: lessons learned from cardiac development. Circ Res. 2010;107:1198–208.
- Dai C, Stolz DB, Kiss LP, Monga SP, Holzman LB, Liu Y. Wnt/beta-catenin signaling promotes podocyte dysfunction and albuminuria. J Am Soc Nephrol. 2009;20:1997–2008.
- He W, Dai C, Li Y, Zeng G, Monga SP, Liu Y. Wnt/ beta-catenin signaling promotes renal interstitial fibrosis. J Am Soc Nephrol. 2009;20:765–76.
- Buechling T, Bartscherer K, Ohkawara B, Chaudhary V, Spirohn K, Niehrs C, . Wnt/Frizzled signaling requires dPRR, the Drosophila homolog of the prorenin receptor. Curr Biol. 2010;20:1263–8.
- Hermle T, Saltukoglu D, Grunewald J, Walz G, Simons M. Regulation of Frizzled-dependent planar polarity signaling by a V-ATPase subunit. Curr Biol. 2010;20:1269–76.
- Huelsken J, Behrens J. The Wnt signalling pathway. J Cell Sci. 2002;115(Pt 21):3977–8.
- Gordon MD, Nusse R. Wnt signaling: multiple pathways, multiple receptors, and multiple transcription factors. J Biol Chem. 2006;281:22429–33.
- Roszko I, Sawada A, Solnica-Krezel L. Regulation of convergence and extension movements during vertebrate gastrulation by the Wnt/PCP pathway. Semin Cell Dev Biol. 2009;20:986–97.
- Kinouchi K, Ichihara A, Sano M, Sun-Wada GH, Wada Y, Kurauchi-Mito A, . The (pro)renin receptor/ATP6AP2 is essential for vacuolar H+-ATPase assembly in murine cardiomyocytes. Circ Res. 2010;107:30–4.
- Riediger F, Quack I, Qadri F, Hartleben B, Park JK, Potthoff SA, . Prorenin receptor is essential for podocyte autophagy and survival. J Am Soc Nephrol. 2011;22: 2193–202.
- Oshima Y, Kinouchi K, Ichihara A, Sakoda M, Kurauchi-Mito A, Bokuda K, . Prorenin receptor is essential for normal podocyte structure and function. J Am Soc Nephrol. 2011;22:2203–12.
- Grigoryan T, Wend P, Klaus A, Birchmeier W. Deciphering the function of canonical Wnt signals in development and disease: conditional loss- and gain-of-function mutations of beta-catenin in mice. Genes Dev. 2008;22:2308–41.
- Pulkkinen K, Murugan S, Vainio S. Wnt signaling in kidney development and disease. Organogenesis. 2008;4:55–9.
- Baurand A, Zelarayan L, Betney R, Gehrke C, Dunger S, Noack C, . Beta-catenin downregulation is required for adaptive cardiac remodeling. Circ Res. 2007;100:1353–62.
- Nishi T, Forgac M. The vacuolar (H+)-ATPases—nature's most versatile proton pumps. Nat Rev Mol Cell Biol. 2002;3:94–103.
- Grabe M, Oster G. Regulation of organelle acidity. J Gen Physiol. 2001;117:329–44.
- Jentsch TJ. Chloride and the endosomal-lysosomal pathway: emerging roles of CLC chloride transporters. J Physiol. 2007;578(Pt 3):633–40.
- Casey JR, Grinstein S, Orlowski J. Sensors and regulators of intracellular pH. Nat Rev Mol Cell Biol. 2010;11:50–61.
- Conner SD, Schmid SL. Regulated portals of entry into the cell. Nature. 2003;422:37–44.
- Di Fiore PP, De Camilli P. Endocytosis and signaling. an inseparable partnership. Cell. 2001;106:1–4.
- Yamamoto H, Komekado H, Kikuchi A. Caveolin is necessary for Wnt-3a-dependent internalization of LRP6 and accumulation of beta-catenin. Dev Cell. 2006;11:213–23.
- Hurtado-Lorenzo A, Skinner M, El Annan J, Futai M, Sun-Wada GH, Bourgoin S, . V-ATPase interacts with ARNO and Arf6 in early endosomes and regulates the protein degradative pathway. Nat Cell Biol. 2006;8:124–36.
- Lange C, Prenninger S, Knuckles P, Taylor V, Levin M, Calegari F. The H(+) vacuolar ATPase maintains neural stem cells in the developing mouse cortex. Stem Cells Dev. 2011;20:843–50.
- Advani A, Kelly DJ, Cox AJ, White KE, Advani SL, Thai K, . The (Pro)renin receptor: site-specific and functional linkage to the vacuolar H+ -ATPase in the kidney. Hypertension. 2009;54:261–9.
- Thiel S, Behrmann I, Dittrich E, Muys L, Tavernier J, Wijdenes J, . Internalization of the interleukin 6 signal transducer gp130 does not require activation of the Jak/STAT pathway. Biochem J. 1998;330(Pt 1):47–54.
- Panigada M, Porcellini S, Barbier E, Hoeflinger S, Cazenave PA, Gu H, . Constitutive endocytosis and degradation of the pre-T cell receptor. J Exp Med. 2002; 195:1585–97.
- Incardona JP, Lee JH, Robertson CP, Enga K, Kapur RP, Roelink H. Receptor-mediated endocytosis of soluble and membrane-tethered Sonic hedgehog by Patched-1. Proc Natl Acad Sci USA. 2000;97:12044–9.
- McCarthy RA, Barth JL, Chintalapudi MR, Knaak C, Argraves WS. Megalin functions as an endocytic sonic hedgehog receptor. J Biol Chem. 2002;277:25660–7.
- Cousin C, Bracquart D, Contrepas A, Corvol P, Muller L, Nguyen G. Soluble form of the (pro)renin receptor generated by intracellular cleavage by furin is secreted in plasma. Hypertension. 2009;53:1077–82.
- Biswas KB, Nabi AN, Arai Y, Nakagawa T, Ebihara A, Ichihara A, . Qualitative and quantitative analyses of (pro)renin receptor in the medium of cultured human umbilical vein endothelial cells. Hypertens Res. 2011; 34:735–9.
- Gonzalez AA, Lara LS, Luffman C, Seth DM, Prieto MC. Soluble form of the (pro)renin receptor is augmented in the collecting duct and urine of chronic angiotensin II-dependent hypertensive rats. Hypertension. 2011;57:859–64.