Abstract
Brown adipose tissue (BAT) is the site of sympathetically activated adaptive thermogenesis during cold exposure and after hyperphagia, thereby controlling whole-body energy expenditure (EE) and body fat. BAT thermogenesis is primarily dependent on the energy-dissipating activity of uncoupling protein 1 (UCP1). There are two types of UCP1-expressing adipocyte, classical brown and beige/brite adipocytes. Recent radionuclide studies have demonstrated the existence of metabolically active BAT composed of mainly beige/brite adipocytes in adult humans. Human BAT is activated by acute cold exposure, being positively correlated to cold-induced increases in EE. The inverse relationship between the BAT activity and body fatness suggests that BAT, because of its energy-dissipating activity, is protective against body fat accumulation. In fact, either repeated cold exposure or daily ingestion of some food ingredients acting on transient receptor potential channels recruited BAT in association with increased EE and decreased body fat. Moreover, possible contribution of BAT to glucose tolerance has been suggested. In addition to the sympathetic nervous system, some endocrine factors also have potential for activation/recruitment of BAT. Thus, BAT is a promising therapeutic target for combating human obesity and related metabolic disorders.
Brown adipose tissue (BAT), because of its energy-dissipating activity, is protective against body fat accumulation in humans.
Repeated cold exposure or daily ingestion of some food ingredients acting on transient receptor potential channels recruits BAT in association with increased energy expenditure and decreased body fat in healthy non-obese subjects.
BAT is a promising therapeutic target for combating human obesity and related metabolic disorders.
Introduction
We are now facing a global increase in obesity and related metabolic disorders, which underscores the urgent need for effective treatments. As obesity is a result of prolonged positive energy balance, it can be treated by reducing food intake and/or increasing energy expenditure (EE). For the latter, while increased physical activity is usually recommended, current attention has also been paid to brown adipose tissue (BAT). BAT has long been recognized in small rodents as the major site of adaptive non-shivering thermogenesis during cold exposure and probably during spontaneous overfeeding, and thereby contributing to the regulation of body fat content. BAT thermogenesis is primarily dependent on uncoupling protein 1 (UCP1), a unique mitochondrial membrane protein, and is directly regulated by sympathetic nerves distributing abundantly to this tissue. In humans, although BAT was once thought to present only in infants, recent radionuclide imaging studies have revealed the existence of a considerable amount of BAT in adult humans (Citation1–5). The metabolic activity of human BAT can be assessed by fluorodeoxyglucose (FDG)-positron emission tomography (PET) combined with X-ray computed tomography (CT), one of the powerful diagnostic tools for malignant tumors. Both clinical and experimental FDG-PET/CT studies have demonstrated an inverse relationship between BAT activity and body fat content, suggesting BAT as a regulatory site of thermogenesis and body fat metabolism in humans (Citation2–4).
In addition to rediscovery of human BAT, several major advancements have also been made in the research field of BAT over the past years. First, studies in rodents revealed two types of UCP1-positive adipocytes arising from distinct developmental lineages: ‘classical brown adipocytes’ and ‘beige/brite adipocytes’ (Citation6,Citation7). Several key transcriptional regulators and signaling molecules in the developmental pathways of brown and beige/brite adipocytes have been identified. Second, while the sympathetic nervous system is a central regulator of BAT function, some endocrine/paracrine molecules were identified as significant activators/recruiters of BAT (Citation8). Finally, very recent studies investigating the effects of BAT transplantation suggest that BAT may also regulate glucose and lipid metabolism through some body fat-independent mechanisms (Citation9,Citation10).
BAT is thus an intriguing target for combating human obesity and associated metabolic disorders. In this review, we summarize the regulatory roles of BAT in energy homeostasis and body fatness, and some mechanisms to activate and recruit BAT with reference to their anti-obesity potential.
There are two types of UCP1-expressing adipocytes
The major BAT depot in small rodents is found in the interscapular region. Brown adipocytes in this depot originate from Myf5-positive myoblastic cells that also give rise to skeletal muscle cells (Citation11), and are called ‘classical brown adipocytes’. The structure of classical brown adipocytes is characterized by numerous small lipid droplets and a large number of mitochondria harboring UCP1 in the inner membrane. UCP1-expressing adipocytes also develop in fat depots usually considered as white adipose tissue (WAT) after prolonged cold exposure or repeated administration of sympathomimetics including β-adrenoceptor (βAR) agonists (Citation12–14). These adipocytes, named ‘beige or brite adipocytes’, arise from lineages developmentally distinct from classical brown adipocytes; they originate from a Myf5-negative precursor cells (Citation15,Citation16) and/or are transdifferentiated from white adipocytes (Citation17). Despite a common expression of some specific genes including UCP1, Cidea, and PRDM16, these two types of adipocyte have distinguishing gene signatures (Citation18), for example, Zic1 in classical brown adipocytes and Hoxc9 in beige/brite adipocytes. In brief, classical brown adipocytes display a strong myogenic signature compared to brite/beige adipocytes.
The presence of BAT in adult humans was first suggested in clinical observations in patients having malignant tumors (Citation19,Citation20): that is, FDG-PET/CT showed, in some cases, substantial and bilateral FDG uptake into adipose tissue in the supraclavicular and paravertebral regions. These were revisited by the observations that FDG uptake in these regions is markedly increased after acute cold exposure (Citation2,Citation3,Citation5), and that tissue samples isolated from these regions contain numerous multilocular adipocytes expressing UCP1 (Citation2–5,Citation21). It is thus undoubted that adult humans have considerable amounts of metabolically active BAT.
Wu et al. (Citation15) and Sharp et al. (Citation22) independently proposed that BAT of adult humans is mainly composed of beige/brite adipocytes because supraclavicular fat expresses rodent- validated marker genes of beige/brite adipocytes, but not those of classical brown adipocytes. However, some other groups reported the expression of both classical brown and beige/brite markers in the supraclavicular/neck fat of adults (Citation23,Citation24). These conflicting results might be due to where tissue samples were obtained. Indeed, BAT in the interscapular depot of neonates, the most characteristic BAT depot in small rodents, expresses genes specific to classical brown adipocytes, whereas that in the supraclavicular depot expresses beige/brite marker genes (Citation25). Taken together, human BAT may comprise both classical brown and beige/brite adipocytes, but its distribution may depend on the regions and age (Citation24,Citation25). It should be noted that any conclusion derived from using such marker genes would be limited by the reliability of the marker chosen. Thus, further studies are needed to categorize better the cell types of human BAT and, more importantly, to clarify their metabolic activities and regulatory roles in whole-body energy metabolism as discussed later.
BAT contributes to adaptive thermogenesis
It has been established in small rodents that BAT is the major site for adaptive non-shivering thermogenesis, a physiological process during which heat production increases in response to environmental cues (Citation26,Citation27). One of the typical examples is cold-induced thermogenesis (CIT), an increase in EE seen when mammals are exposed to lower temperatures (Citation28,Citation29). For example, when mice are exposed to cold at 4°C, sympathetic nerve activity in BAT is increased to activate UCP1, which short-circuits the electrochemical gradient that drives ATP synthesis, thereby dissipating energy as heat. Heat generated in BAT is distributed to the rest of the body through the circulation to prevent body temperature drop. The indispensable role of BAT for CIT was clearly confirmed by findings that mice lacking UCP1 are intolerant to cold exposure (Citation30) and have a much shorter life-span in the cold (Citation31).
In humans, BAT was once thought to have a physiological role only in infants whose shivering thermogenesis ability is still immature. However, the recent rediscovery of a considerable amount of BAT in adults rekindled research into the physiological significance of human BAT. Particularly, the fact that human BAT can be best detected by FDG-PET/CT after acute cold exposure implies a significant role of BAT in CIT. To test this, we measured whole-body EE under thermoneutral conditions (27°C) and after acute cold exposure (19°C, 2 hours) in healthy subjects with wide ranges of body composition and BAT activity () (Citation32,Citation33). EE was positively correlated with fat-free lean body mass both under the thermoneutral condition and after cold exposure, while EE was positively correlated with BAT activity only after cold exposure. Thus, CIT calculated from the difference between EE under the two conditions was positively correlated with BAT activity, but not with lean body mass. Consistently with these results, there have been reports that CIT seen under conditions inducing no muscle shivering is higher in a subject group with detectable BAT activities than in those without them (Citation34–37). All these results strongly support an idea that BAT plays a significant role in CIT in healthy adult humans. Moreover, they may suggest a minor role of shivering thermogenesis by skeletal muscle, which is a major metabolically active component of fat-free mass, at least under mild cold conditions. This was further supported by the observation of negligible effects of cold exposure on FDG uptake into skeletal muscle () (Citation10,Citation34).
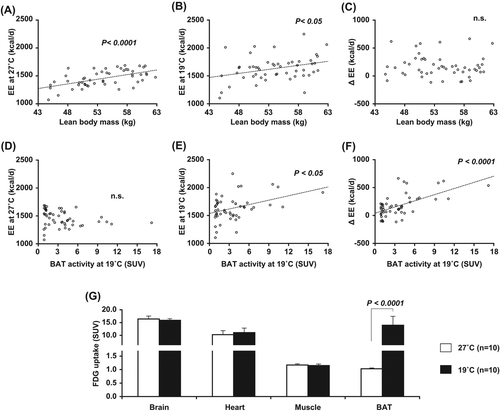
Another example of adaptive thermogenesis is diet-induced thermogenesis (DIT), an increase in EE seen after food intake. Since the first report of BAT hyperplasia in small rodents kept on prolonged overfeeding on highly palatable cafeteria-diets (Citation38), it has repeatedly been reported that BAT mass and/or UCP1 expression are decreased in animals deprived of food but increased in those fed on high-calorie diets (Citation26,Citation27), suggesting that the thermogenic capacity of BAT is increased in response to excessive energy intake, thereby functioning as a homeostatic buffer of EE. However, this does not necessarily mean that BAT is the major site of DIT (Citation39). In fact, to our knowledge, there has been no direct evidence for postprandial activation of BAT thermogenesis in small rodents.
A possible contribution of BAT to DIT has also been investigated in adult humans. Vosselman et al. (Citation40) reported that FDG uptake into BAT depots was increased a couple of hours after ingestion of a high-calorie meal. It is to be noted, however, that a similar increase in FDG uptake was also observed in skeletal muscle, probably because of the action of insulin released after meal ingestion. We also measured EE for 2 hours after meal ingestion, and found that postprandial thermogenesis is slightly higher in individuals with higher BAT activities (Citation41). Moreover, Nagai et al. reported a significant effect of UCP1 gene polymorphism on postprandial thermogenesis after a high-fat meal in healthy boys (Citation42). Thus, BAT seems to be involved, at least in part, in DIT, while its quantitative contribution relative to other tissues including skeletal muscle remains to be investigated not only in humans but also in small rodents.
BAT activity inversely relates to body fatness
It has repeatedly been reported in small rodents that thermogenic activity of BAT is inversely related to body fatness. For example, almost all obese model animals express lower levels of UCP1 in BAT, while mice over-expressing UCP1 are leaner (Citation26,Citation27). Moreover, mice lacking UCP1 are susceptible to diet-induced obesity when they are maintained at thermoneutral temperatures (∼29°C), whereas they did not show obese phenotype in conventional animal room temperatures (∼20°C) (Citation30,Citation43). BAT is thus accepted as a long-term regulator of energy balance and body fat content.
Consistent with this view, retrospective readings of FDG-PET/CT in thousands of patients have revealed that BAT prevalence is lower in patients with higher body mass index (BMI) (Citation4,Citation44–46). Prospective studies in healthy participants also demonstrated an inverse correlation between BAT activity and body fat content () (Citation2,Citation3,Citation47). It is to be noted that the apparent association between BAT and adiposity may suggest a protective role of BAT in body fat accumulation but does not give any conclusion for their causal relationship. Vijgen et al. reported the tendency of increased BAT activity and CIT after severe weight loss by bariatric surgery in morbidly obese patients (Citation48). An insignificant increase in BAT activity was also reported in patients losing body fat by life-style intervention (Citation49). This may be explained by the changes in the subcutaneous fat layer and the surface-to-volume ratio: that is, severe weight loss decreases the insulation capacity of the subcutaneous fat layer and thereby recruits BAT due to the need for additional thermogenesis to maintain body temperature.
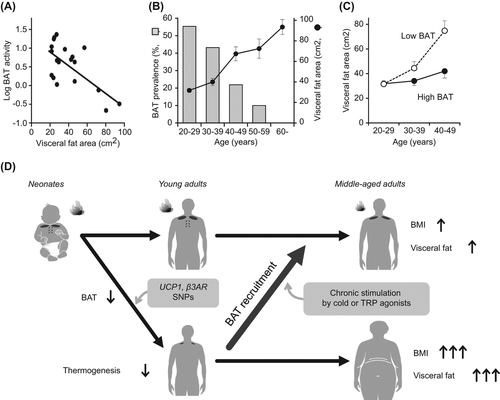
Moreover, the apparent association between BAT and body fatness should be carefully evaluated because these are influenced by age in small rodents (Citation50,Citation51) and more greatly in humans. A strong impact of age on human BAT is reported in both clinical and experimental studies (Citation44–47). In our previous study (Citation47), for example, the prevalence of cold-activated BAT is more than 50% in young subjects in their 20s, decreased with age, and is less than 10% in subjects in their 50s and 60s (). On the other hand, the aging process produces notable changes in EE and body composition: that is, in general, percent body fat increases, while physical activity, daily EE, lean body mass, and bone mineral density decrease with age (Citation52). Thus, it is possible that the inverse relationship between BAT and adiposity might be a spurious correlation. In fact, the apparent association of BAT with adiposity was reported in studies for older populations, but not in those of younger populations (Citation47,Citation53).
In more detailed analysis of the effects of BAT on age-related accumulation of body fat, we found that subjects without detectable BAT were prone to age-related accumulation of visceral fat, whereas those with detectable BAT were slim even in their 40s and 50s () (Citation47). These results, together with a finding that mice lacking UCP1 showed severe diet-induced obesity in an age-dependent manner (Citation54), suggest that the age-related decrease in BAT activity gradually but significantly accelerates body fat accumulation in humans (). This is also supported by our studies showing that single nucleotide polymorphisms at –3826 A to G of the UCP1 gene and at 64 Trp to Arg of the beta3 adrenergic receptor (β3AR) gene accelerate age-related decrease in BAT activity in parallel with an increase in body fatness (Citation55).
At present, the physiological mechanisms underlying the age-related reduction of BAT are largely unknown. It is to be noted that sympathetic nerve activity and sympathetic signaling to BAT increase with age, implying a decreased sensitivity of BAT to sympathetic stimulation (Citation50). Very recently, it was reported that the age-related impairment of BAT is accelerated by orexin deficiency but rescued by orexin administration, suggesting a role of orexin in the long-term regulation of BAT (Citation51).
Studies in small rodents show BAT as an anti-obesity target
Given that BAT is protective against body fat accumulation, activation and/or recruitment of BAT might be expected to be a hopeful therapeutic regimen for combating obesity. This is particularly intriguing because people with lower or undetectable BAT activities are more obese and to be treated. Based on the stimulatory role of the sympathetic nervous system in BAT thermogenesis, there have been numerous trials to recruit BAT in small rodents. Typical are prolonged cold exposure and β3AR agonist treatment, both of which give rise to an increased UCP1 expression, mitochondriogenesis, and proliferation/differentiation of classical brown adipocytes, all of which result in BAT hyperplasia and an increased thermogenic capacity, and thereby a reduction of body fat content (Citation26,Citation27). Chronic cold exposure and repeated β3AR agonist treatment produce not only BAT hyperplasia but also a remarkable induction of beige/brite adipocytes in white fat pads (Citation12–14), which may also contribute to the reduction of body fat.
Recent studies using isolated and/or in vitro differentiated adipocytes demonstrated that beige/brite adipocytes have comparable thermogenic activities to classical brown adipocytes (Citation56–58). However, as the total amount of UCP1 protein in beige/brite adipocytes per animal is low, the relative contribution of these cells to overall thermogenic capacity may be marginal. In this context, several lines of evidence suggest significant roles of beige/brite adipocytes compared to classical brown adipocytes for body fat regulation. In a series of studies using recombinant inbred mouse strains, Kozak's group found that susceptibility to diet-induced obesity was associated more strongly with the propensity of beige/brite induction than UCP1 expression levels in interscapular BAT composed of classical brown adipocytes (Citation59,Citation60). Moreover, several mouse models with an increased number of beige/brite adipocytes are protected from diet-induced obesity. For example, Fabp4-PRDM16 transgenic mice, which show beige/brite adipocyte induction in subcutaneous white fat pads without substantially changing UCP1 levels in classical BAT, display increased EE and limited weight gain under a high-fat diet (Citation61).
Thus, beige/brite adipocytes are responsible for reducing body fat, despite their relatively small amounts of UCP1. This may imply the contribution of some mechanisms/factors derived from beige/brite adipocytes in white fat pads, in addition to their thermogenic activity, to whole-body EE and adiposity. In this context, there are interesting reports that ectopic expression of very low levels of UCP1 in epididymal fat pads ameliorates leptin sensitivity through afferent nerve signals from the fat tissue to the hypothalamus (Citation62,Citation63). Transplantation of a tissue fragment of BAT into intra-abdominal white fat pads was also effective in decreasing body fatness even though the recipient mouse had considerable amounts of its own BAT (Citation9,Citation64). As various endocrine and paracrine factors including fibroblast growth factor 21 (FGF21) and interleukin-6 (IL-6) are known to be produced in BAT (Citation56,Citation65,Citation66), some of them may be involved in the regulation of EE and adiposity.
Chronic cold exposure recruits BAT and reduces body fat in humans
The anti-obesity effect of BAT is now well established in small rodents, regardless of whether it is attributed to classical brown or beige/brite adipocytes, or both. This encourages us to confirm it in humans. As mentioned in the previous sections, cold exposure is the most powerful and physiological stimulus for reactivation and recruitment of BAT. In fact, it has been reported that BAT was more detectable in winter, and in outdoor workers and people who died of cold (Citation2,Citation26,Citation44,Citation67–69). We examined the effects of repeated cold exposure on BAT activity and also body fat content in healthy volunteers with low or undetectable activities of BAT (Citation33). After chronic cold exposure at 17°C for 2 h every day for 6 weeks, their BAT activity significantly increased (). Van der Lans et al. also reported increased BAT activity and volume in human adults placed in cold suits to acclimatize them to cold conditions a few hours a day for 10 days (Citation37). These findings, being comparable with those in small rodents, indicate that BAT can be recruited by chronic cold exposure. It was also found that the daily cold exposure increased CIT, with increments positively related to those in BAT activity. These results, together with a highly positive correlation between CIT and BAT activity, indicate that recruited BAT actually contributes to CIT. Most important of our findings is that body fat mass significantly decreased after the chronic cold exposure, whereas body weight and fat-free mass did not change. The change in body fat mass was inversely correlated with those in BAT activity and CIT, suggesting a significant contribution of recruited BAT to body fat reduction.
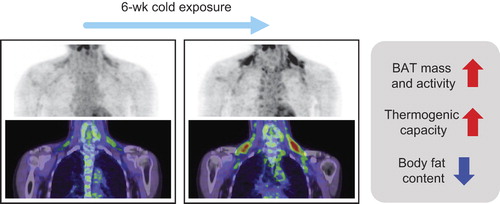
Since the cause-and-effect relationship between BAT and body fatness is not fully clarified in humans, as noted above, it may be possible that the body fat reduction seen after chronic cold exposure may be a cause, not a result, of increased BAT. However, in our study using non-obese healthy subjects, the decrease in body fat mass was only 0.7 kg. It is thus highly unlikely that such a small reduction results in a significant change in insulation capacity of the subcutaneous fat layer. Apparent BAT recruitment without body fat change was also found after only a 10-day cold acclimation (Citation37). All this taken together, it is concluded that human BAT can be recruited by chronic cold exposure, thereby increasing whole-body EE and decreasing body fat even in individuals who have lost detectable activities of BAT. Although it remains to be tested whether the recruited human BAT is composed of classical brown or beige/brite adipocytes, Lee et al. recently reported that human neck preadipocytes differentiated into beige/brite adipocytes more than classical brown adipocytes in vitro (Citation56). The same group also demonstrated that UCP1-positive adipocytes were differentiated even from preadipocytes isolated from subjects without detectable BAT in FDG-PET/CT (Citation70), supporting again a high ability of recruitment of human BAT.
Thermoreceptor agonists can mimic the effects of cold
Although daily cold exposure can recruit human BAT, it would seem difficult to increase exposure to cold in daily life. Moreover, it is to be noted that mice exposed to cold at 4°C show increased cardiovascular risk such as atherosclerotic plaque growth or instability in mice (Citation71), although human BAT can be activated and recruited by rather mild cold conditions at 10–19°C (Citation33,Citation37). It is known that the stimulatory effects of cold on BAT are initiated by peripheral stimulation of transient receptor potential (TRP) channels in sensory neurons (Citation72,Citation73). TRP vanilloid (TRPV) is a family of transient receptor potential ion channels. TRPV1 is activated by a number of environmental cues such as heat greater than 43°C and some pungent compounds in chili peppers such as capsaicin. Capsaicin is known to increase EE and fat oxidation through the activation of the adreno-sympathetic nervous system, and to reduce body fat in small rodents and also in humans (Citation74,Citation75). However, because of its strong pungency, not all people can take it in large quantities. Capsinoids, non-pungent capsaicin analogs, are also known to activate TRPV1 to a similar extent to capsaicin. Although capsinoids are much less pungent than capsaicin, they are as potent as capsaicin to increase sympathetic nerve activity, thermogenesis, EE, and fat oxidation, and to reduce body fat both in small rodents and humans (Citation74–76). We reported previously that a single oral ingestion of capsinoids increases EE in human individuals with metabolically active BAT, but not in those without it, indicating that the thermogenic effect of capsinoids is mediated through the activation of BAT (Citation77).
Such acute effects of capsinoids are quite similar to those of cold exposure, thus implying repeated capsinoid treatment can mimic the chronic effects of cold exposure and may recruit BAT in humans. To test this idea, we examined the effects of a daily ingestion of capsinoids on BAT and whole-body EE in subjects with low or undetectable activities of BAT (Citation33). EE under a thermoneutral condition at 27°C was almost the same before and 6 weeks after the daily ingestion of capsinoids or a placebo, while EE after 2-h cold exposure at 19°C was increased only after capsinoid treatment. Consequently, CIT after capsinoid treatment was significantly higher than before the treatment and after placebo treatment. As CIT is proportional to BAT activity assessed by FDG-PET/CT, and thus a predictive index of BAT activity, the capsinoid-induced increase in CIT would reflect an enhancement of the thermogenic capacity and activity of BAT. These results, together with the stimulatory effects of capsinoids on BAT-dependent EE (Citation77), suggest that the anti-obesity effects of capsinoids are attributable, at least in part, to the thermogenic activity of recruited BAT. The primary action site of capsaicin/capsinoids would be TRPV1 on sensory neurons located in the gastrointestinal tract, but it seems also possible that a part of the effects may be through the action on TRPV1 expressed in BAT and WAT (Citation78). TRPV1 as well as other subtypes of TRP may also be involved in the direct action of cold on white and beige adipocytes (Citation79).
Not only agonists of TRPV1 but also those of other subtypes of TRPs are candidates for BAT recruiters. For example, TRPM8 and TRPA1 are the most likely receptor candidates sensitive to low temperatures. The mean activation temperatures of TRPA1 and TRPM8 are around 20°C, being comparable with those applied in human studies to activate BAT. There are various ingredients in food acting as agonists for these TRPs (Citation80), a representative of which is menthol, a cooling and flavor compound in mint, acting on TRPM8. TRPA1 is activated by allyl- and benzyl-isothiocyanates, pungent elements in mustard and wasabi (Japanese horseradish). Animal studies have demonstrated that these compounds increase BAT thermogenesis through the activation of TRPs and the sympathetic nervous system, and decrease body fat. Thus, these food ingredients as well as capsaicin/capsinoids are promising as an anti-obesity regimen easily applicable in daily life.
Endocrine and paracrine activators of BAT thermogenesis
The effects of cold exposure and TRP stimulation on BAT are primarily mediated through the activation of the sympathetic nerve and βAR system, which is undoubtedly a central regulator of brown and beige adipocytes. In addition to or in combination with this system, some hormones and factors have been identified as activators/recruiters of BAT (). A representative is triiodothyronine (T3), which is well established as a potent transcriptional activator of the UCP1 gene. There are reports showing an association of BAT activity with thyroid function both in mice and humans (Citation81). However, it is noted that T3 in BAT is produced from thyroxin (T4) by the action of type II deiodinase (D2) in this tissue, more than derived from circulating T3, at least in small rodents (Citation81). A critical role of D2 for BAT thermogenesis was confirmed by a finding that D2-deficient mice were cold-sensitive (Citation82). As D2 in BAT is greatly activated by noradrenaline released from sympathetic nerves (Citation83), it is likely that the sympathetic nervous system is important for the thyroidal control of BAT thermogenesis and recruitment. D2 in BAT is also shown to be activated by bile acids coming from the liver (Citation84). This may explain why bile acids cause increased EE and reduced body fatness without considerable effect on food intake. Thus, the effects of thyroid hormones are to be evaluated in association with these other interacting factors.
FGF21, another liver-derived endocrine factor, has gathered increasing attention because it reduces body fatness and improves glucose tolerance when administrated to rodent models of obesity and type 2 diabetes (Citation85). FGF21 has potential for inducing the thermogenic program in BAT, and also for driving beige/brite adipocyte recruitment in WAT (Citation86). This may be a cause of the favorable effects on metabolism, and crucial for activating thermogenesis particularly in neonates, where hepatic production of FGF21 is markedly increased in response to suckling (Citation87). In addition to being produced in the liver, FGF21 is also produced in BAT after cold exposure and adrenergic stimulation (Citation56,Citation88). Thus, BAT is not only a target for but also a secreting site of FGF21, indicating an autocrine action of FGF21 on BAT (Citation65).
There have been reports demonstrating significant roles of heart-derived natriuretic peptides (NP), well-known regulators of fluid and hemodynamic homeostasis, in BAT recruitment (Citation89). NP enhances whole-body EE, probably due to an upregulation of UCP1 in BAT and also due to induction of WAT browning (Citation90). Importantly, high circulating levels of NP are found after cold exposure (Citation90,Citation91), and associated with weight loss in humans (Citation91), suggesting its physiological and clinical relevance. Mechanistically, NP triggers lipolysis, UCP1 expression, and WAT browning through its binding to a specific receptor NPRA and resulting activation of cyclic GMP-dependent protein kinase, which phosphorylates various proteins responsible for lipid metabolism and thermogenesis (Citation92). Thus, this pathway works in parallel with the well-established βAR-cyclic AMP-dependent protein kinase pathway.
Irisin was first identified as a peroxisome proliferator-activated receptor γ co-activator-1α (PGC-1α)-dependent myokine that is cleaved from a membrane protein, fibronectin type III domain-containing 5 (FNDC5). Irisin promotes a thermogenic gene program in primary adipocytes in vitro, and adenoviral delivery of FNDC5 in the mouse liver induces beige/brite adipocytes in WAT and protects animals from diet-induced obesity (Citation93). Since endurance exercise was reported to increase tissue expression and circulating level of irisin, this unique peptide seems to be a factor responsible for various beneficial effects of exercise, including on body fatness. However, the recent data in humans are rather controversial (Citation94). In fact, there is a report that neither longer-term nor single exercise markedly increases skeletal muscle FNDC5 expression or serum irisin levels (Citation95). Some studies showed a positive correlation of serum irisin with BMI (Citation96,Citation97), while others reported a negative correlation (Citation98). Moreover, the human FNDC5 gene was revealed to have a mutation of the conserved start codon ATG to ATA, and thereby producing only a small amount of full-length protein (Citation99). It is thus obvious that further studies are needed, particularly to translate the beneficial effects of irisin observed in mice to humans.
Perspectives
It is now accepted that BAT is significantly involved in adaptive thermogenesis, and thereby in the regulation of whole-body EE and body fatness, in not only small rodents but also adult humans. Human BAT can be recruited even in individuals who have undetectably small amounts of BAT and thereby get obese. This encourages us to develop practical, easy, and effective anti-obesity regimens, particularly by searching for thermogenic food ingredients and endogenous factors inducing beige/brite adipocytes. In addition to the regulatory roles of BAT in EE and body fatness, BAT may be relevant to some metabolic disorders. Some clinical studies of thousands of patients have shown a negative association of BAT activity with diabetic status or blood glucose level (Citation4,Citation46,Citation100,Citation101). We have also found a significant independent impact of cold-activated BAT on blood glucose and hemoglobin A1c (HbA1c) in healthy human subjects, suggesting a possible effect of BAT on glucose metabolism, in addition to but independently of those of body fatness (Citation102). This is compatible with the well-known ameliorating effect of cold exposure on insulin sensitivity (Citation103,Citation104), and also with recent reports in mice demonstrating augmented glucose tolerance and insulin sensitivity in animals transplanted with a tissue piece of BAT (Citation9,Citation64,Citation105) or brown adipocytes differentiated from human iPS cells in vitro (Citation10). Moreover, a regulatory role of BAT was proposed for plasma triglyceride metabolism (Citation10,Citation106,Citation107). Thus, BAT may be an intriguing target for combating not only obesity but also some related metabolic disorders.
Declaration of interest: The authors report no conflicts of interest.
This study was supported by a Grant-in-Aid for Scientific Research from the Ministry of Education, Culture, Sports, Science, and Technology of Japan (22590227), and a Special Research Grant from Tenshi College.
References
- Nedergaard J, Bengtsson T, Cannon B. Unexpected evidence for active brown adipose tissue in adult humans. Am J Physiol Endocrinol Metab. 2007;293:E444–52.
- Saito M, Okamatsu-Ogura Y, Matsushita M, Watanabe K, Yoneshiro T, Nio-Kobayashi J, et al. High incidence of metabolically active brown adipose tissue in healthy adult humans: effects of cold exposure and adiposity. Diabetes. 2009;58:1526–31.
- van Marken Lichtenbelt WD, Vanhommerig JW, Smulders NM, Drossaerts JM, Kemerink GJ, Bouvy ND, et al. Cold-activated brown adipose tissue in healthy men. N Engl J Med. 2009;360:1500–8.
- Cypess AM, Lehman S, Williams G, Tal I, Rodman D, Goldfine AB, et al. Identification and importance of brown adipose tissue in adult humans. N Engl J Med. 2009;360:1509–17.
- Virtanen KA, Lidell ME, Orava J, Heglind M, Westergren R, Niemi T, et al. Functional brown adipose tissue in healthy adults. N Engl J Med. 2009;360:1518–25.
- Wu J, Cohen P, Spiegelman BM. Adaptive thermogenesis in adipocytes: is beige the new brown?Genes Dev. 2013;27:234–50.
- Harms M, Seale P. Brown and beige fat: development, function and therapeutic potential. Nat Med. 2013;19:1252–63.
- Villarroya F, Vidal-Puig A. Beyond the sympathetic tone: the new brown fat activators. Cell Metab. 2013;17:638–43.
- Stanford KI, Middelbeek RJ, Townsend KL, An D, Nygaard EB, Hitchcox KM, et al. Brown adipose tissue regulates glucose homeostasis and insulin sensitivity. J Clin Invest. 2013;123:215–23.
- Nishio M, Yoneshiro T, Nakahara M, Suzuki S, Saeki K, Hasegawa M, et al. Production of functional classical brown adipocytes from human pluripotent stem cells using specific hemopoietin cocktail without gene transfer. Cell Metab. 2012;16:394–406.
- Seale P, Bjork B, Yang W, Kajimura S, Chin S, Kuang S, et al. PRDM16 controls a brown fat/skeletal muscle switch. Nature. 2008;454:961–7.
- Cousin B, Cinti S, Morroni M, Raimbault S, Ricquier D, Pénicaud L, et al. Occurrence of brown adipocytes in rat white adipose tissue: molecular and morphological characterization. J Cell Sci. 1992;103: 931–42.
- Nagase I, Yoshida T, Kumamoto K, Umekawa T, Sakane N, Nikami H, et al. Expression of uncoupling protein in skeletal muscle and white fat of obese mice treated with thermogenic beta 3-adrenergic agonist. J Clin Invest. 1996;97:2898–904.
- Guerra C, Koza RA, Yamashita H, Walsh K, Kozak LP. Emergence of brown adipocytes in white fat in mice is under genetic control. Effects on body weight and adiposity. J Clin Invest. 1998;102:412–20.
- Wu J, Boström P, Sparks LM, Ye L, Choi JH, Giang AH, et al. Beige adipocytes are a distinct type of thermogenic fat cell in mouse and human. Cell. 2012;150:366–76.
- Petrovic N, Walden TB, Shabalina IG, Timmons JA, Cannon B, Nedergaard J. Chronic peroxisome proliferator-activated receptor gamma (PPARgamma) activation of epididymally derived white adipocyte cultures reveals a population of thermogenically competent, UCP1-containing adipocytes molecularly distinct from classic brown adipocytes. J Biol Chem. 2010;285:7153–64.
- Cinti S. Transdifferentiation properties of adipocytes in the adipose organ. Am J Physiol Endocrinol Metab. 2009;297:E977–86.
- Waldén TB, Hansen IR, Timmons JA, Cannon B, Nedergaard J. Recruited vs. nonrecruited molecular signatures of brown, “brite,” and white adipose tissues. Am J Physiol Endocrinol Metab. 2012; 302:E19–31.
- Cohade C, Osman M, Pannu HK, Wahl RL. Uptake in supraclavicular area fat (“USA-Fat”): description on 18F-FDG PET/CT. J Nucl Med. 2003;44:170–6.
- Minotti AJ, Shah L, Keller K. Positron emission tomography/computed tomography fusion imaging in brown adipose tissue. Clin Nucl Med. 2004;29:5–11.
- Zingaretti MC, Crosta F, Vitali A, Guerrieri M, Frontini A, Cannon B, et al. The presence of UCP1 demonstrates that metabolically active adipose tissue in the neck of adult humans truly represents brown adipose tissue. FASEB J. 2009;23:3113–20.
- Sharp LZ, Shinoda K, Ohno H, Scheel DW, Tomoda E, Ruiz L, et al. Human BAT possesses molecular signatures that resemble beige/brite cells. PLoS One. 2012;7:e49452.
- Jespersen NZ, Larsen TJ, Peijs L, Daugaard S, Homøe P, Loft A, et al. A classical brown adipose tissue mRNA signature partly overlaps with brite in the supraclavicular region of adult humans. Cell Metab. 2013;17:798–805.
- Cypess AM, White AP, Vernochet C, Schulz TJ, Xue R, Sass CA, et al. Anatomical localization, gene expression profiling and functional characterization of adult human neck brown fat. Nat Med. 2013;19:635–9.
- Lidell ME, Betz MJ, Dahlqvist Leinhard O, Heglind M, Elander L, Slawik M, et al. Evidence for two types of brown adipose tissue in humans. Nat Med. 2013;19:631–4.
- Cannon B, Nedergaard J. Brown adipose tissue: function and physiological significance. Physiol Rev. 2004;84:277–359.
- Lowell BB, Spiegelman BM. Towards a molecular understanding of adaptive thermogenesis. Nature. 2000;404:652–60.
- Fedorenko A, Lishko PV, Kirichok Y. Mechanism of fatty-acid- dependent UCP1 uncoupling in brown fat mitochondria. Cell. 2012; 151:400–13.
- Ricquier D. Uncoupling protein 1 of brown adipocytes, the only uncoupler: a historical perspective. Front Endocrinol (Lausanne). 2011;2:85.
- Enerbäck S, Jacobsson A, Simpson EM, Guerra C, Yamashita H, Harper ME, et al. Mice lacking mitochondrial uncoupling protein are cold-sensitive but not obese. Nature. 1997;387:90–4.
- Golozoubova V, Hohtola E, Matthias A, Jacobsson A, Cannon B, Nedergaard J. Only UCP1 can mediate adaptive nonshivering thermogenesis in the cold. FASEB J. 2001;15:2048–50.
- Yoneshiro T, Aita S, Matsushita M, Kameya T, Nakada K, Kawai Y, et al. Brown adipose tissue, whole-body energy expenditure, and thermogenesis in healthy adult men. Obesity (Silver Spring). 2011; 19:13–16.
- Yoneshiro T, Aita S, Matsushita M, Kayahara T, Kameya T, Kawai Y, et al. Recruited brown adipose tissue as an antiobesity agent in humans. J Clin Invest. 2013;123:3404–8.
- Orava J, Nuutila P, Lidell ME, Oikonen V, Noponen T, Viljanen T, et al. Different metabolic responses of human brown adipose tissue to activation by cold and insulin. Cell Metab. 2011;14:272–9.
- Ouellet V, Labbé SM, Blondin DP, Phoenix S, Guérin B, Haman F, et al. Brown adipose tissue oxidative metabolism contributes to energy expenditure during acute cold exposure in humans. J Clin Invest. 2012;122:545–52.
- Chen KY, Brychta RJ, Linderman JD, Smith S, Courville A, Dieckmann W, et al. Brown fat activation mediates cold-induced thermogenesis in adult humans in response to a mild decrease in ambient temperature. J Clin Endocrinol Metab. 2013;98:E1218–23.
- van der Lans AA, Hoeks J, Brans B, Vijgen GH, Visser MG, Vosselman MJ, et al. Cold acclimation recruits human brown fat and increases nonshivering thermogenesis. J Clin Invest. 2013;123:3395–403.
- Rothwell NJ, Stock MJ. A role for brown adipose tissue in diet-induced thermogenesis. Nature. 1979;281:31–5.
- Kozak LP. Brown fat and the myth of diet-induced thermogenesis. Cell Metab. 2010;11:263–7.
- Vosselman MJ, Brans B, van der Lans AA, Wierts R, van Baak MA, Mottaghy FM, et al. Brown adipose tissue activity after a high-calorie meal in humans. Am J Clin Nutr. 2013;98:57–64.
- Saito M, Aita S, Yoneshiro T. Postprandial thermogenesis and brown adipose tissue in humans. Obesity (Silver Spring). 2011;19:S79.
- Nagai N, Sakane N, Ueno LM, Hamada T, Moritani T. The -3826 A--> G variant of the uncoupling protein-1 gene diminishes postprandial thermogenesis after a high fat meal in healthy boys. J Clin Endocrinol Metab. 2003;88:5661–7.
- Feldmann HM, Golozoubova V, Cannon B, Nedergaard J. UCP1 ablation induces obesity and abolishes diet-induced thermogenesis in mice exempt from thermal stress by living at thermoneutrality. Cell Metab. 2009;9:203–9.
- Ouellet V, Routhier-Labadie A, Bellemare W, Lakhal-Chaieb L, Turcotte E, Carpentier AC, et al. Outdoor temperature, age, sex, body mass index, and diabetic status determine the prevalence, mass, and glucose-uptake activity of 18F-FDG-detected BAT in humans. J Clin Endocrinol Metab. 2011;96:192–9.
- Pfannenberg C, Werner MK, Ripkens S, Stef I, Deckert A, Schmadl M, et al. Impact of age on the relationships of brown adipose tissue with sex and adiposity in humans. Diabetes. 2010;59:1789–93.
- Lee P, Greenfield JR, Ho KK, Fulham MJ. A critical appraisal of the prevalence and metabolic significance of brown adipose tissue in adult humans. Am J Physiol Endocrinol Metab. 2010;299:E601–6.
- Yoneshiro T, Aita S, Matsushita M, Okamatsu-Ogura Y, Kameya T, Kawai Y, et al. Age-related decrease in cold-activated brown adipose tissue and accumulation of body fat in healthy humans. Obesity (Silver Spring). 2011;19:1755–60.
- Vijgen GH, Bouvy ND, Teule GJ, Brans B, Hoeks J, Schrauwen P, et al. Increase in brown adipose tissue activity after weight loss in morbidly obese subjects. J Clin Endocrinol Metab. 2012;97:E1229–33.
- Orava J, Nuutila P, Noponen T, Parkkola R, Viljanen T, Enerbäck S, et al. Blunted metabolic responses to cold and insulin stimulation in brown adipose tissue of obese humans. Obesity (Silver Spring). 2013;21:2279–87.
- Florez-Duquet M, McDonald RB. Cold-induced thermoregulation and biological aging. Physiol Rev. 1998;78:339–58.
- Sellayah D, Sikder D. Orexin restores aging-related brown adipose tissue dysfunction in male mice. Endocrinology. 2014;155:485–501.
- St-Onge MP, Gallagher D. Body composition changes with aging: the cause or the result of alterations in metabolic rate and macronutrient oxidation?Nutrition. 2010;26:152–5.
- Gilsanz V, Smith ML, Goodarzian F, Kim M, Wren TA, Hu HH. Changes in brown adipose tissue in boys and girls during childhood and puberty. J Pediatr. 2012;160:604–9.
- Kontani Y, Wang Y, Kimura K, Inokuma KI, Saito M, Suzuki-Miura T, et al. UCP1 deficiency increases susceptibility to diet-induced obesity with age. Aging Cell. 2005;4:147–55.
- Yoneshiro T, Ogawa T, Okamoto N, Matsushita M, Aita S, Kameya T, et al. Impact of UCP1 and β3AR gene polymorphisms on age-related changes in brown adipose tissue and adiposity in humans. Int J Obes (Lond). 2013;37:993–8.
- Lee P, Werner CD, Kebebew E, Celi FS. Functional thermogenic beige adipogenesis is inducible in human neck fat. Int J Obes (Lond). 2014;38:170–6.
- Okamatsu-Ogura Y, Fukano K, Tsubota A, Omachi A, Uozumi1 A, Terao A, et al. Thermogenic ability of uncoupling protein 1 in beige adipocytes in mice. PLoS One. 2013;8:e84229.
- Shabalina IG, Petrovic N, de Jong JM, Kalinovich AV, Cannon B, Nedergaard J. UCP1 in brite/beige adipose tissue mitochondria is functionally thermogenic. PLoS One. 2013;8:e79980.
- Guerra C, Koza RA, Walsh K, Kurtz DM, Wood PA, Kozak LP. Abnormal nonshivering thermogenesis in mice with inherited defects of fatty acid oxidation. J Clin Invest. 1998;102:1724–31.
- Xue B, Rim JS, Hogan JC, Coulter AA, Koza RA, Kozak LP. Genetic variability affects the development of brown adipocytes in white fat but not in interscapular brown fat. J Lipid Res. 2007;48:41–51.
- Seale P, Conroe HM, Estall J, Kajimura S, Frontini A, Ishibashi J, et al. Prdm16 determines the thermogenic program of subcutaneous white adipose tissue in mice. J Clin Invest. 2011;121:96–105.
- Yamada T, Katagiri H, Ishigaki Y, Ogihara T, Imai J, Uno K, et al. Signals from intra-abdominal fat modulate insulin and leptin sensitivity through different mechanisms: neuronal involvement in food-intake regulation. Cell Metab. 2006;3:223–9.
- Okamatsu-Ogura Y, Nio-Kobayashi J, Iwanaga T, Terao A, Kimura K, Saito M. Possible involvement of uncoupling protein 1 in appetite control by leptin. Exp Biol Med (Maywood). 2011;236:1274–81.
- Zhu Z, Spicer EG, Gavini CK, Goudjo-Ako AJ, Novak CM, Shi H. Enhanced sympathetic activity in mice with brown adipose tissue transplantation (transBATation). Physiol Behav. 2014;125:21–9.
- Villarroya J, Cereijo R, Villarroya F. An endocrine role for brown adipose tissue?Am J Physiol Endocrinol Metab. 2013;305:E567–72.
- Burýsek L, Houstĕk J. beta-Adrenergic stimulation of interleukin- 1alpha and interleulin-6 expression in mouse brown adipocytes. FEBS Lett. 1997;411:83–6.
- Cohade C, Mourtzikos KA, Wahl RL.”USA-Fat”: prevalence is related to ambient outdoor temperature-evaluation with 18F-FDG PET/CT. J Nucl Med. 2003;44:1267–70.
- Lee P, Swarbrick MM, Ho KK. Brown adipose tissue in adult humans: a metabolic renaissance. Endocr Rev. 2013;34:413–38.
- Huttunen P, Hirvonen J, Kinnula V. The occurrence of brown adipose tissue in outdoor workers. Eur J Appl Physiol Occup Physiol. 1981;46:339–45.
- Lee P, Swarbrick MM, Zhao JT, Ho KK. Inducible brown adipogenesis of supraclavicular fat in adult humans. Endocrinology. 2011;152: 3597–602.
- Dong M, Yang X, Lim S, Cao Z, Honek J, Lu H, et al. Cold exposure promotes atherosclerotic plaque growth and instability via UCP1- dependent lipolysis. Cell Metab. 2013;18:118–29.
- Nakamura K. Central circuitries for body temperature regulation and fever. Am J Physiol Regul Integr Comp Physiol. 2011;301:R1207–28.
- Dhaka A, Viswanath V, Patapoutian A. TRP ion channels and temperature sensation. Annu Rev Neurosci. 2006;29:135–61.
- Saito M, Yoneshiro T. Capsinoids and related food ingredients activating brown fat thermogenesis and reducing body fat in humans. Curr Opin Lipidol. 2013;24:71–7.
- Ludy MJ, Moore GE, Mattes RD. The effects of capsaicin and capsiate on energy balance: critical review and meta-analyses of studies in humans. Chem Senses. 2012;37:103–21.
- Snitker S, Fujishima Y, Shen H, Ott S, Pi-Sunyer X, Furuhata Y, et al. Effects of novel capsinoid treatment on fatness and energy metabolism in humans: possible pharmacogenetic implications. Am J Clin Nutr. 2009;89:45–50.
- Yoneshiro T, Aita S, Kawai Y, Iwanaga T, Saito M. Nonpungent capsaicin analogs (capsinoids) increase energy expenditure through the activation of brown adipose tissue in humans. Am J Clin Nutr. 2012;95:845–50.
- Bishnoi M, Kondepudi KK, Gupta A, Karmase A, Boparai RK. Expression of multiple transient receptor potential channel genes in murine 3T3-L1 cell lines and adipose tissue. Pharmacol Rep. 2013;65:751–5.
- Ye L, Wu J, Cohen P, Kazak L, Khandekar MJ, Jedrychowski MP, et al. Fat cells directly sense temperature to activate thermogenesis. Proc Natl Acad Sci U S A. 2013;110:12480–5.
- Yoneshiro T, Saito M. Transient receptor potential activated brown fat thermogenesis as a target of food ingredients for obesity management. Curr Opin Clin Nutr Metab Care. 2013;16:625–31.
- Bianco A, McAninch E. The role of thyroid hormone and brown adipose tissue in energy homoeostasis. Lancet Diabetes Endcrinol. 2013;1:250–8.
- de Jesus LA, Carvalho SD, Ribeiro MO, Schneider M, Kim SW, Harney JW, et al. The type 2 iodothyronine deiodinase is essential for adaptive thermogenesis in brown adipose tissue. J Clin Invest. 2001;108:1379–85.
- Silva JE, Larsen PR. Adrenergic activation of triiodothyronine production in brown adipose tissue. Nature. 1983;305:712–13.
- Watanabe M, Houten SM, Mataki C, Christoffolete MA, Kim BW, Sato H, et al. Bile acids induce energy expenditure by promoting intracellular thyroid hormone activation. Nature. 2006;439:484–9.
- Iglesias P, Selgas R, Romero S, Díez JJ. Biological role, clinical significance, and therapeutic possibilities of the recently discovered metabolic hormone fibroblastic growth factor 21. Eur J Endocrinol. 2012;167:301–9.
- Fisher FM, Kleiner S, Douris N, Fox EC, Mepani RJ, Verdeguer F, et al. FGF21 regulates PGC-1α and browning of white adipose tissues in adaptive thermogenesis. Genes Dev. 2012;26:271–81.
- Hondares E, Rosell M, Gonzalez FJ, Giralt M, Iglesias R, Villarroya F. Hepatic FGF21 expression is induced at birth via PPARalpha in response to milk intake and contributes to thermogenic activation of neonatal brown fat. Cell Metab. 2010;11:206–12.
- Chartoumpekis DV, Habeos IG, Ziros PG, Psyrogiannis AI, Kyriazopoulou VE, Papavassiliou AG. Brown adipose tissue responds to cold and adrenergic stimulation by induction of FGF21. Mol Med. 2011;17:736–40.
- Collins S. A heart-adipose tissue connection in the regulation of energy metabolism. Nat Rev Endocrinol. 2014;10:157–63.
- Bordicchia M, Liu D, Amri EZ, Ailhaud G, Dessì-Fulgheri P, Zhang C, et al. Cardiac natriuretic peptides act via p38 MAPK to induce the brown fat thermogenic program in mouse and human adipocytes. J Clin Invest. 2012;122:1022–36.
- Chainani-Wu N, Weidner G, Purnell DM, Frenda S, Merritt-Worden T, Kemp C, et al. Relation of B-type natriuretic peptide levels to body mass index after comprehensive lifestyle changes. Am J Cardiol. 2010;105:1570–6.
- Moro C, Lafontan M. Natriuretic peptides and cGMP signaling control of energy homeostasis. Am J Physiol Heart Circ Physiol. 2013;304:H358–368.
- Boström P, Wu J, Jedrychowski MP, Korde A, Ye L, Lo JC, et al. A PGC1-α-dependent myokine that drives brown-fat-like development of white fat and thermogenesis. Nature. 2012;481:463–8.
- Novelle MG, Contreras C, Romero-Picó A, López M, Diéguez C. Irisin, two years later. Int J Endocrinol. 2013;2013:746281.
- Pekkala S, Wiklund PK, Hulmi JJ, Ahtiainen JP, Horttanainen M, Pöllänen E, et al. Are skeletal muscle FNDC5 gene expression and irisin release regulated by exercise and related to health?J Physiol. 2013;591:5393–400.
- Hee Park K, Zaichenko L, Brinkoetter M, Thakkar B, Sahin-Efe A, Joung KE, et al. Circulating irisin in relation to insulin resistance and the metabolic syndrome. J Clin Endocrinol Metab. 2013;98:4899–907.
- Stengel A, Hofmann T, Goebel-Stengel M, Elbelt U, Kobelt P, Klapp BF. Circulating levels of irisin in patients with anorexia nervosa and different stages of obesity—correlation with body mass index. Peptides. 2013;39:125–30.
- Moreno-Navarrete JM, Ortega F, Serrano M, Guerra E, Pardo G, Tinahones F, et al. Irisin is expressed and produced by human muscle and adipose tissue in association with obesity and insulin resistance. J Clin Endocrinol Metab. 2013;98:E769–78.
- Raschke S, Elsen M, Gassenhuber H, Sommerfeld M, Schwahn U, Brockmann B, et al. Evidence against a beneficial effect of irisin in humans. PLoS One. 2013;8:e73680.
- Jacene HA, Cohade CC, Zhang Z, Wahl RL. The relationship between patients’ serum glucose levels and metabolically active brown adipose tissue detected by PET/CT. Mol Imaging Biol. 2011;13:1278–83.
- Zhang Q, Ye H, Miao Q, Zhang Z, Wang Y, Zhu X, et al. Differences in the metabolic status of healthy adults with and without active brown adipose tissue. Wien Klin Wochenschr. 2013;125:687–95.
- Matsushita M, Yoneshiro T, Aita S, Kameya T, Sugie H, Saito M. Impact of brown adipose tissue on body fatness and glucose metabolism in healthy humans. Int J Obes (Lond).2013 Nov 11. [Epub ahead of print].
- Vallerand AL, Pesusse F, Bukowiecki LJ. Cold exposure potentiates the effect of insulin on in vivo glucose uptake. Am J Physiol. 1987;253:E179–R186.
- Gasparetti AL, de Souza CT, Pereira-da-Silva M, Oliveira RLGS, Saad MJA, Carneiro EM, et al. Cold exposure induces tissue-specific modulation of the insulin-signalling pathway in Rattus norvegicus. J Physiol. 2003;552:149–62.
- Gunawardana SC, Piston DW. Reversal of type 1 diabetes in mice by brown adipose tissue transplant. Diabetes. 2012;61:674–82.
- Bartelt A, Bruns OT, Reimer R, Hohenberg H, Ittrich H, Peldschus K, et al. Brown adipose tissue activity controls triglyceride clearance. Nat Med. 2011;17:200–5.
- Geerling JJ, Boon MR, van der Zon GC, van den Berg SA, van den Hoek AM, Lombès M, et al. Metformin lowers plasma triglycerides by promoting VLDL-triglyceride clearance by brown adipose tissue in mice. Diabetes. 2014;63:880–9.