Abstract
Background Biological rhythmicity has been extensively studied in animals for many decades. Although temporal patterns of physical activity have been identified in humans, no large-scale, multi-national study has been published, and no comparison has been attempted of the ubiquity of activity rhythms at different time scales (such as daily, weekly, monthly, and annual).
Methods Using individually worn actigraphy devices, physical activity of 2,328 individuals from five different countries (adults of African descent from Ghana, South Africa, Jamaica, Seychelles, and the United States) was measured for seven consecutive days at different times of the year.
Results Analysis for rhythmic patterns identified daily rhythmicity of physical activity in all five of the represented nationalities. Weekly rhythmicity was found in some, but not all, of the nationalities. No significant evidence of lunar rhythmicity or seasonal rhythmicity was found in any of the groups.
Conclusions These findings extend previous small-scale observations of daily rhythmicity to a large cohort of individuals from around the world. The findings also confirm the existence of modest weekly rhythmicity but not lunar or seasonal rhythmicity in human activity. These differences in rhythm strength have implications for the management of health hazards of rhythm misalignment.
Analysis of the pattern of physical activity of 2,328 individuals from five countries revealed strong daily rhythmicity in all five countries, moderate weekly rhythmicity in some countries, and no lunar rhythmicity or seasonal rhythmicity in any of the countries.
Key Messages
Introduction
Biological rhythms, many of which are endogenously generated, are ubiquitous and affect the physiology and behavior of possibly all organisms on Earth (Citation1–3). Rhythmic processes that recur in approximately 24 hours (daily or circadian rhythms) have been particularly well studied. In mammals, daily rhythms are under the control of a master pacemaker located in the ventral hypothalamus, which controls endogenous rhythmicity and uses input from the eyes to adjust circadian rhythms to the environmental cycle of light and darkness (Citation4,Citation5).
Daily rhythmicity of activity and other variables in human subjects has been investigated in controlled laboratory studies with a small number of subjects (Citation6–11), and use of ambulatory actigraphy more recently has made it possible to monitor the activity of individuals living natural lives for a year or longer, but again with a limited number of subjects (Citation12,Citation13). Consistently with the results of research on laboratory animals, the laboratory studies on human subjects indicated that humans possess an endogenous clock that oscillates with a period slightly longer than 24 hours and is synchronized by the daily cycle of light and darkness. Most humans start activity within a few hours of sunrise, are active for about 16 hours each day, and stop activity a few hours after sunset. Applied research has shown that rapid or inconsistent changes in the rhythmic environment (such as those associated with transcontinental travel, shift work, or even the extensive use of artificial light in the modern 24-hour society) can cause misalignment between the internal clock and the external time structure. Circadian disruption caused by or resulting in such misalignment can have serious negative health effects, such as cardiovascular disease (Citation14), higher incidence of breast cancer (Citation15), increased occurrence of psychiatric disorders (Citation16), and development of metabolic syndrome (Citation17).
Although daily or circadian rhythms are more pervasive and have been more thoroughly studied, biological rhythmicity in the time scale of weeks, months, and years has been documented in many species (Citation1–3). Seasonal rhythmicity, for example, is very strong in some species (such as in ground squirrels that alternate between being active and homeothermic during the summer and being torpid in the winter), whereas it is not detectable in other species (Citation18).
Various biological rhythms have been studied in human subjects (Citation19), but not even the basic daily pattern of physical activity has been subjected to a large-scale, multi-national study, and no attempt has been made to compare the strength of rhythmicity at different time scales (such as daily, weekly, monthly, and annual). Regarding weekly rhythms, a few studies, mostly involving cross-sectional populational data instead of individual longitudinal data, have documented that people editing articles on Wikipedia (the free online encyclopedia) do it more often on weekdays or on weekends, depending on location around the world (Citation20), that scientists refereeing journal manuscripts tend to do it early in the week, starting on Sunday (Citation21), and that people in general tend to sleep one to three hours longer on Friday and Saturday nights than on weekday nights (Citation22,Citation23). In the temporal scale of months, the frequency of heart attacks (myocardial infarction, ventricular fibrillation, cardiac arrest, etc.) has been shown to vary seasonally by about 3%, peaking in the winter (Citation24–27), while a 30% rise in the occurrence of human spouse-battering has been noted in the summer (Citation28) and a 15% rise in depressive mood, particularly in women, have been noted in the fall and winter (Citation29).
Whereas daily rhythmicity in humans is known to be driven by an endogenous clock, there is no consistent evidence of human endogenous clocks driving weekly, seasonal, or other so-called infradian rhythms, except for the menstrual cycle in women (Citation1–3). Nonetheless, the fact that autonomic and behavioral processes in humans do exhibit weekly and seasonal rhythmicity indicates that the interaction between circadian and infradian rhythms must be investigated if one wishes fully to understand human physiology.
In order to prevent misalignment between internal and external clocks (and the resulting negative health effects), it is necessary first to understand the functioning of the internal clock, which is expressed in the temporal organization of physical activity and other autonomic and behavioral variables. To advance this understanding, we analyzed actigraphy data obtained from more than 2,300 individuals from five countries and looked for rhythmic patterns at various time scales: daily (24 hours), weekly (7 days), lunar (29.5 days), and annual or seasonal (12 months). We were interested in finding out whether people who live in five countries with very different levels of socioeconomic development exhibit different activity patterns and, if so, whether these differences are limited to daily patterns or can also be observed in weekly, lunar, and seasonal patterns of activity.
Material and methods
A total of 2,500 adults of evolutionarily recent African descent, 25–45 years old, were enrolled in the Modeling the Epidemiologic Transition Study between January 2010 and September 2011 (Citation30); 500, approximately 50% of whom were female, were enrolled in each of five study sites: rural Ghana, urban South Africa, urban Jamaica, the small island state of Seychelles, and suburban United States (Maywood, a suburb of Chicago). The study sites represented a range of socioeconomic development as defined by the United Nations’ Human Development Index (HDI), with Ghana as a low–middle HDI country, South Africa as middle, Jamaica and the Seychelles as high, and the United States as a very high HDI country (Citation31). In all locations, individuals with obvious infectious diseases and pregnant or lactating women were excluded. Individuals who had a condition preventing them from engaging in normal physical activities (such as severe arthritis) were also excluded. Participant characteristics are listed in .
Table I. Participant characteristics (means ± standard deviations).
Physical activity was assessed using the Actical accelerometer (Phillips Respironics, Bend, OR, USA). The monitor was worn at the waist, positioned just behind the right hip. Participants were asked to wear the monitor at all times for 8 days, except while bathing, showering, or swimming. Reliable accelerometer data, recorded continuously for a week with 1-min resolution, were obtained from 2,328 men and women (454 from Ghana, 496 from South Africa, 429 from Jamaica, 450 from Seychelles, and 499 from the United States). All protocols for the study were approved by the Institutional Review Board or Ethics Committee of the participating institutions.
To compare the strength of daily, weekly, lunar, and seasonal rhythms of activity, one would ideally monitor each subject continuously for many years, an arrangement that is not feasible when thousands of individuals are involved. As an alternate approach, we sampled data segments from the week-long records of subjects monitored at various times of the year. Holidays were not controlled for, but, because data were collected over more than 1.5 years, holidays occurred at various times for different people, so that systematic biases were unlikely to occur.
To ensure that shorter cycles would not be favored by greater representation in the data set, a computer program written specifically for this study arranged the actigraphic data by reading each of the 2,328 individual files and computing physical activity as movement counts per hour within specific time intervals. To allow direct comparison of the ubiquity of daily, weekly, lunar, and seasonal rhythms through two-way ANOVAs with equal statistical power, the actigraphic data were arranged into four time intervals for each of the four cycles, as shown in . To enhance the similarity of statistical power in the various tests, the data were culled to produce comparable data sets according to a between-subjects design, as follows: for daily rhythmicity, only data from one of the four time intervals (and only from the second day of recording) were used from each subject. Data culling was done stochastically, using the computer’s random number generator with a new seed for each data set. For weekly rhythmicity, only data from the second day of recording were used from each subject, that day being assigned to the appropriate time interval (day of the week) based on the actual day of the week of the recording. For lunar rhythmicity, only data from the second day of recording were used, and the lunar phase of this day was determined by an algorithm based on the number of days since a known new moon (18 November 1998) and reducing this modulo 29.53058867 (the length of a synodic month). For seasonal rhythmicity, data from the second day of recording were used and were assigned to the appropriate month interval. When combining different countries for the ANOVA on seasonal rhythmicity, two arrangements were tested: one matched by calendar and one matched by season (with time intervals of countries in the southern hemisphere being in opposite order of those of countries in the northern hemisphere).
Table II. The time intervals used for the four cycles.
Although the procedure described in the preceding paragraph allowed for unbiased comparison of the strength of daily, weekly, lunar, and seasonal rhythms of activity, it did so at the cost of discarding large sections of data. Thus, additional analyses were conducted to take advantage of the available data. For the analysis of daily rhythmicity, the records of all 2,328 subjects were used for a repeated-measures ANOVA comparing the 24 hours of a day (averaged over several successive days for each subject). Additionally, the cosinor procedure was used to confirm 24-hour rhythmicity (Citation32). Although not all subjects had full 168-hour records, the records of 1,339 subjects were available for a repeated-measures ANOVA comparing the seven days of the week. For the analysis of seasonal rhythmicity, the records of all 2,328 subjects were used for a between-subjects ANOVA comparing the 12 months of the year. In all three cases, activity was expressed as counts per hour. Lunar rhythmicity could not be analyzed in the same manner because the data could not be arranged in either a repeated-measures or a between-subjects design with greater resolution than that attained in the procedure described in the previous paragraph.
Because a total of eight separate ANOVAs were conducted (five in the primary analysis and three in the secondary analysis), all probabilities expected under the null hypotheses were multiplied by 8 to prevent Type I errors. Corrected probabilities smaller than 0.05 were considered to be statistically significant. Considering that the high statistical power resulting from the large number of data points might give emphasis to trivial results, indices of effect size (ω2) were provided whenever possible.
Results and discussion
The results of the primary (unbiased) analysis are shown in . Two-way ANOVAs (country × time interval) revealed statistically significant effects in some but not all conditions, as detailed in . The main effect of country was significant for all four time scales, and the consistency among the time scales was to be expected given that the data were sampled from the same set of subjects for the analyses of the four time scales. The effect size was modest, with the effect of country being associated with only 2.8% of the total variance (2.8% being the average ω2 × 100 of the five tests of the effect of country in ). As previously reported, Ghanaians were slightly but significantly more active than residents of the United States, with residents of the other three countries somewhere in between, varying with sex (Citation33). The effect size of daily rhythmicity was much more robust, accounting for 22.8% of the variance in the data (), which indicates that physical activity varies consistently with time of day. A modest interaction effect (2.5%) was also present, indicating that time of day had a slightly greater effect in some countries than others.
Figure 1. Results of the unbiased analysis. The bars represent mean values (± SEM) of physical activity (in counts per hour ×1,000) for the four time intervals of each of the four cycles in each of the five countries. Codes for the time intervals are listed in Table II. The results of two-way ANOVAs are shown in Table III. Asterisks indicate probabilities associated with the F statistics obtained in post hoc one-way ANOVAs for each panel: ***P < 0.0001, *P < 0.05. Absence of asterisks indicates P > 0.10. When the main effect is significant, significance of multiple comparisons by Tukey’s test is indicated by lower-case letters: bars with different letters are significantly different from each other.
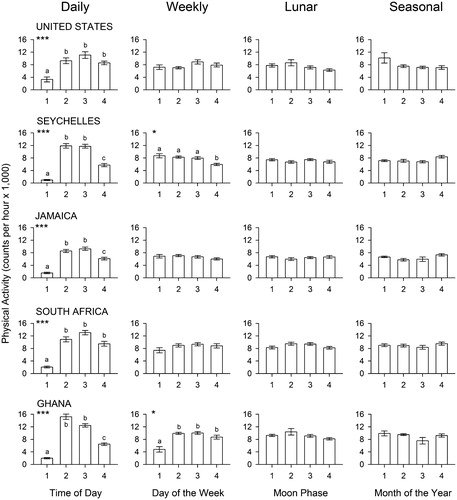
Table III. Results of two-way ANOVAs for the primary (unbiased) analysis.
One-way ANOVAs conducted post hoc on the same data sets for each country separately showed statistically significant daily variation of physical activity in all five countries, even if its strength varied from 8% in the United States to 40% in Ghana. Generally, activity was lowest at night (segment 1, with a grand mean of 1,636 counts per hour) and highest in the afternoon (segment 3, with a grand mean of 11,312 counts per hour). However, as indicated by the interaction effect in the two-way ANOVA, the temporal distribution of activity was not identical in the five countries. Notably, activity was higher in the morning (segment 2) than in the afternoon (segment 3) in Ghana, although differences between morning and afternoon were not significant in any of the five countries.
Secondary analysis for daily rhythmicity involved a repeated-measures ANOVA for consecutive hourly segments (). Here again, statistically significant rhythmicity was found in all countries, as well as in the combined data set of all 2,328 subjects: F(23, 53475) = 570.299, P < 0.0001. As seen in , the level of activity is relatively stable from 7 a.m. to 6 p.m., being lower prior to and after this interval. Cosinor analysis confirmed the presence of a rhythmic pattern with robustness (i.e. rhythm strength) at 85% and acrophase (i.e. idealized peak time) at 13:18 hours. This daily temporal variation is consistent with the findings of various small-scale studies that documented circadian rhythmicity of locomotor activity in humans (Citation6–11).
Figure 2. Results of the secondary analysis for the combined data set of men and women from the five countries. The bars represent mean values (± SEM) of physical activity (in counts per hour ×1,000). Asterisks indicate probabilities (corrected for multiple testing) associated with the F statistics obtained in one-way ANOVAs for each panel: ***P < 0.0001. Absence of asterisks indicates P > 0.10. When the main effect is significant, significance of multiple comparisons by Tukey’s test is indicated by lower-case letters: bars with different letters are significantly different from each other.
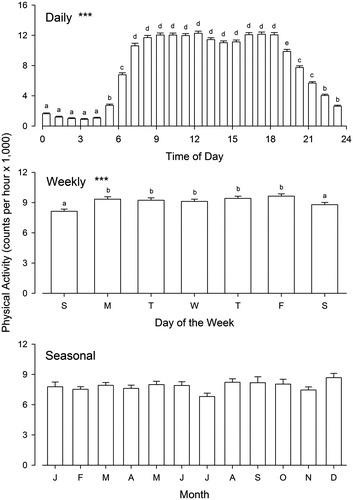
The two-way ANOVA for weekly rhythmicity revealed a modest but significant main effect of day of the week (with an effect size of not quite 1%) and no significant interaction with country ( and ). Individual post hoc one-way ANOVAs showed weekly variation of physical activity only in participants from Seychelles (ω2 = 0.04 with less activity in the weekend [segment 4] than in week days) and Ghana (ω2 = 0.03 with lower activity on Mondays and Tuesdays [segment 1]). The secondary analysis involved a repeated-measures ANOVA for seven consecutive days (). Here, statistically significant rhythmicity was found for the five countries combined, F(6, 8028) = 14.697, P < 0.0001, although, as in the primary analysis, weekly rhythmicity was found only in residents of Seychelles and Ghana when the countries were tested separately. Weekly variation in human activity has been previously documented in various specific behaviors (Citation12,Citation13,Citation34,Citation35), but the current results indicate that general physical activity does not necessarily follow a weekly pattern. It is likely that some individuals are more active on weekends whereas others are more active during week days, and this distribution evidently varies from country to country. A longitudinal study with several consecutive weeks of data collected from each subject would be needed to sort this out.
The primary analysis of lunar variation of physical activity did not reveal a significant main effect of moon phase or of the interaction between moon phase and country ( and ). The computed effect size was less than 0.5%. The failure to detect lunar variation was not due to lack of adequate statistical power (Type II error), as significant variation was detected in the daily and weekly time domains with an identical experimental design. This failure to find evidence of lunar rhythmicity is noteworthy because, despite the established view that lunar rhythmicity does not affect human physiology and behavior (Citation19), three recent studies have described lunar cycle effects on human sleep (Citation36–38). The authors of a more extensive study of sleep across lunar cycles failed to confirm this effect and recommended the publication of negative results to counter occasional claims of positive results (Citation39). This recommendation is reinforced by the fact that publication bias in the form of selective suppression of null findings has been documented also in the social sciences (Citation40). Thus, the present study serves as one more published record of negative results concerning effects of the lunar cycle on human physiology and behavior.
Whether trimesters were arranged chronologically or by seasons, the two-way ANOVA for seasonal rhythmicity failed to identify either an effect of seasonal variation or an effect of interaction between season and country (). Effect sizes were less than 0.5%. The secondary analysis involved a one-way ANOVA for 12 months with different subjects in each month (). Here again, no significant rhythmicity was detected: F(11, 2308) = 1.240, P > 0.500. It should be noted that, although there is not much seasonal variation of day length in Jamaica (18 °N), and almost no variation in Seychelles (4 °S), there is considerable seasonal variation in photoperiod in the Chicago area (latitude 42 °N), which could have been associated with changes in physical activity. The absence of seasonal changes may reflect the availability of artificial lighting (as well as ventilation, heating, and air conditioning) throughout the year.
In conclusion, this study revealed strong daily rhythmicity, modest weekly rhythmicity, and no lunar or seasonal rhythmicity of physical activity in data from 2,328 individuals from five different countries. Although previous studies had addressed rhythmicity in these four time domains separately, the present study provided for the first time a direct comparison of the strengths of the four rhythmic domains in human activity.
In this study, daily rhythmicity was found to account for 23% of the variance in physical activity, whereas weekly rhythmicity accounted for less than 1% of the total variance. Differences from one country to another accounted for 3% of the variance. This means that differences from one country to another have a much smaller impact on physical activity than has daily rhythmicity. Also, differences from one country to another have a greater impact on physical activity than has weekly rhythmicity. An implication of these findings is that misalignment between internal and external clocks is unlikely to have medical consequences for weekly, lunar, or seasonal rhythms, for the simple reason that these rhythms are either too weak on non-existent. In contrast, daily rhythmicity (as gauged by the rhythm of physical activity) is quite strong, and misalignment between the circadian clock and the environmental light–dark cycle has been shown to have serious negative health effects (Citation14–17). The fact that we found daily rhythmicity to be strong and to follow a similar pattern in residents of five countries differing greatly in socioeconomic development suggests that therapeutic procedures for circadian misalignment developed in one region of the world will likely be effective in other regions as well.
Limitations of this study include, as indicated above, the fact that longitudinal data were not available for analysis of weekly, lunar, and seasonal rhythms. By culling the data, the primary analysis ensured that the strength of daily rhythmicity was not overestimated, and it is unlikely that long-term records will provide evidence of strong rhythmicity in the scale of weeks, moon phase, and seasons. Nonetheless, at least the feeble weekly rhythmicity that we identified can be studied in more detail with data collected over several consecutive weeks. Another limitation of the study is that only physical activity was used as a measure of biological rhythmicity. Studies in many animal species have shown that the daily rhythm of locomotor activity has a temporal pattern very similar to that of the body temperature rhythm (Citation41), but it is possible that physical activity does not reflect accurately the weekly, lunar, or seasonal rhythmicity of an organism. Thus, studies using different variables for the monitoring of rhythmicity could lead to different conclusions about the relative strength of these four rhythmic processes.
Future studies might address the limitations indicated above by examining weekly rhythmicity through a longitudinal approach and by evaluating all four temporal domains using a variable other than physical activity. Also, although the five countries included in the present study spanned a wide range of socioeconomic development, future studies might include a larger number of countries to ensure that the chosen countries constitute a representative sample of the world population, both ethnically and culturally.
Declaration of interest
The authors report no conflicts of interest.
M.S. was supported by a Fulbright Scholar grant from the U.S. Council for International Exchange of Scholars. The Modeling the Epidemiologic Transition Study is funded in part by the U.S. National Institutes of Health (1R01DK080763).
References
- Dunlap JC, Loros JJ, DeCoursey PJ. Chronobiology: biological timekeeping. Sunderland (MA): Sinauer; 2004.
- Koukkari WL, Sothern RB. Introducing biological rhythms. New York: Springer; 2006.
- Refinetti R. Circadian physiology. 2nd ed. Boca Raton (FL): CRC Press; 2006.
- Dibner C, Schibler U, Albrecht U. The mammalian circadian timing system: organization and coordination of central and peripheral clocks. Ann Rev Physiol. 2010;72:517–49.
- Golombek DA, Rosenstein RE. Physiology of circadian entrainment. Physiol Rev. 2010;90:1063–102.
- Aschoff J, Gerecke U, Wever R. Desynchronization of human circadian rhythms. Jpn J Physiol. 1967;17:450–7.
- Czeisler CA, Weitzman ED, Moore-Ede MC, Zimmerman JC, Knauer RS. Human sleep: its duration and organization depend on its circadian phase. Science. 1980;210:1264–7.
- Gander PH, Connell LJ, Graeber RC. Masking of the circadian rhythm of heart rate and core temperature by the rest-activity cycle in man. J Biol Rhythms. 1986;1:119–35.
- Chandrashekaran MK, Marimuthu G, Geetha L. Correlations between sleep and wake in internally synchronized and desynchronized circadian rhythms in humans under prolonged isolation. J Biol Rhythms. 1997;12:26–33.
- Natale V. Circadian motor asymmetries in humans. Neurosci Lett. 2002;320:102–4.
- Eastman CI, Molina TA, Dziepak ME, Smith MR. Blacks (African Americans) have shorter free-running circadian periods than Whites (Caucasian Americans). Chronobiol Int. 2012;29:1072–7.
- Binkley S, Tome MB, Crawford D, Mosher K. Human daily rhythms measured for one year. Physiol Behav. 1990;48:293–8.
- Yoneyama S, Hashimoto S, Honma K. Seasonal changes of human circadian rhythms in Antarctica. Am J Physiol Reg Integr Comp Physiol. 1999;277:R1091–7.
- Nagai M, Hoshide S, Kario K. Role of 24-hour blood pressure management in preventing kidney disease and stroke. Contrib Nephrol. 2013;179:67–80.
- Jia Y, Lu Y, Wu K, Lin Q, Shen W, Zhu M, et al. Does night work increase the risk of breast cancer? A systematic review and meta-analysis of epidemiological studies. Cancer Epidemiol. 2013;37:197–206.
- Jagannath A, Peirson SN, Foster RG. Sleep and circadian rhythm disruption in neuropsychiatric illness. Curr Opin Neurobiol. 2013;23:888–94.
- Reiter RJ, Tan DX, Korkmaz A, Ma S. Obesity and metabolic syndrome: association with chronodisruption, sleep deprivation, and melatonin suppression. Ann Med. 2012;44:564–77.
- Geiser F. Metabolic rate and body temperature reduction during hibernation and daily torpor. Ann Rev Physiol. 2004;66:239–74.
- Foster RG, Roenneberg T. Human responses to the geophysical daily, annual and lunar cycles. Curr Biol. 2008;18:784–94.
- Yasseri T, Sumi R, Kertész J. Circadian patterns of Wikipedia editorial activity: a demographic analysis. PLoS One. 2012;7:e30091.
- Cutting JE. Rhythms of research. Psychol Sci. 2007;18:1023–6.
- Mauvieux B, Gouthière L, Sesboüe B, Davenne D. Etudes comparées des rythmes circadiens et reflet actimétrique du sommeil de sportifs et de sédentaires en poste régulier de nuit. Can J Appl Physiol. 2003;28:831–87.
- Roenneberg T, Wirz-Justice A, Merrow M. Life between clocks: daily temporal patterns of human chronotypes. J Biol Rhythms. 2003;18:80–90.
- Spielberg C, Falkenhahn D, Willich SN, Wegscheider K, Völler H. Circadian, day-of-week, and seasonal variability in myocardial infarction: comparison between working and retired patients. Am Heart J. 1996;132:579–85.
- Allegra JR, Cochrane DG, Allegra EM, Cable G. Calendar patterns in the occurrence of cardiac arrest. Am J Emerg Med. 2002;20:513–17.
- Müller D, Lampe F, Wegscheider K, Schultheiss HP, Behrens S. Annual distribution of ventricular tachycardias and ventricular fibrillation. Am Heart J. 2003;146:1061–5.
- Reavey M, Saner H, Paccaud F, Marques-Vidal P. Exploring the periodicity of cardiovascular events in Switzerland: variation in deaths and hospitalizations across seasons, day of the week and hour of the day. Int J Cardiol. 2013;168:2195–200.
- Michael RP, Zumpe D. An annual rhythm in the battering of women. Am J Psychiatry. 1986;143:637–40.
- Harmatz MG, Well AD, Overtree CE, Kawamura KY, Rosal M, Ockene IS. Seasonal variation of depression and other moods: a longitudinal approach. J Biol Rhythms. 2000;15:344–50.
- Luke A, Bovet P, Forrester TE, Lambert EV, Plange-Rhule J, Schoeller DA, et al. Protocol for the modeling the epidemiologic transition study: a longitudinal observational study of energy balance and change in body weight, diabetes and cardiovascular disease risk. BMC Public Health. 2011;11:927.
- Barro RJ, Lee JW. A new data set of educational attainment in the world, 1950-2010. Working Paper No. 15902. The National Bureau of Economic Research; 2011.
- Refinetti R, Cornélissen G, Halberg F. Procedures for numerical analysis of circadian rhythms. Biol Rhythm Res. 2007;38:275–325.
- Luke A, Bovet P, Plange-Rhule J, Forrester TE, Lambert EV, Schoeller DA, et al. A mixed ecologic-cohort comparison of physical activity and weight among young adults from five populations of African origin. BMC Public Health. 2014;14:397.
- Palmer JD, Udry JR, Morris NM. Diurnal and weekly, but no lunar rhythm in human copulation. Human Biol. 1982;54:111–21.
- De Castro JM. Weekly rhythms of spontaneous nutrient intake and meal pattern of humans. Physiol Behav. 1991;50:729–38.
- Röösli M, Jüni P, Braun-Fahrländer C, Brinkhof MWG, Low N, Egger M. Sleepless night, the moon is bright: longitudinal study of lunar phase and sleep. J Sleep Res. 2006;15:149–53.
- Cajochen C, Altanay-Ekici S, Münch M, Frey S, Knoblauch V, Wirz-Justice A. Evidence that the lunar cycle influences human sleep. Curr Biol. 2013;23:1485–8.
- Turányi CZ, Rónai KZ, Zoller R, Véber O, Czira ME, Ujszászi A, et al. Association between lunar phase and sleep characteristics. Sleep Med. 2014;15:1411–16.
- Cordi M, Ackermann S, Bes FW, Hartmann F, Konrad BN, Genzel L, et al. Lunar cycle effects on sleep and the file drawer problem. Curr Biol. 2014;24:R549–50.
- Franco A, Malhotra N, Simonovits G. Publication bias in the social sciences: unlocking the file drawer. Science. 2014;345:1502–5.
- Refinetti, R. The circadian rhythm of body temperature. Front Biosci. 2010;15:564–94.