Abstract
Objective. We aimed to characterize the expression of indoleamine 2,3-dioxygenase (IDO) or IDO-induced tryptophan degradation-dependent pathways, which may lead to suppression of T cells and possible protection against atherosclerosis.
Methods and results. Expression of IDO and IDO-related pathway components was analyzed in advanced human atherosclerotic plaques (n = 24) and in non-atherosclerotic arteries (n = 6). Up-regulation of IDO and genes related to the IDO pathway was found to be pronounced in atherosclerotic plaques. Immunohistochemistry demonstrated IDO protein in the atheromatous core and co-distribution with monocyte-macrophages (CD68-positive cells). In gene-set enrichment analysis, the IDO pathway revealed a significant (false discovery rate (FDR) = 0.07) regulatory T cell, fork-head box protein 3 (FoxP3)-initiated CD28-cytotoxic T lymphocyte-associated antigen 4 (CTLA-4)-inducible T cell co-stimulator (ICOS)-driven pathway leading to activation of IDO expression in antigen-presenting cells (APCs). Expression of these IDO pathway genes varied between 2.1- and 16.8-fold as compared to control tissues (P < 0.05 for all).
Conclusions. IDO and the IDO-related pathway are important mediators of the immunoinflammatory responses in advanced atherosclerosis offering new viable therapeutic targets for the development of antiatherogenic immunosuppressive therapies.
Key messages
Indoleamine 2,3-dioxygenase (IDO) expression was significantly up-regulated in atherosclerotic tissue compared to non-atherosclerotic control tissue. In a self-constructed hypothetical IDO pathway several genes involved in signaling between T cells and antigen-presenting cells (APCs) function in a co-operating manner, which may lead to suppression of T cell activity and possible inhibition of atherosclerosis.
Immunohistochemistry of atherosclerotic lesions demonstrated IDO expression in the atheromatous core of carotid artery plaques and co-localization with CD68-positive cells.
In humans IDO and the IDO-related pathway are important mediators of the immunoinflammatory responses in advanced atherosclerosis offering previously uncharacterized viable therapeutic targets and possibilities for the development of novel antiatherogenic immunosuppressive therapies.
Abbreviations | ||
AHA | = | American Heart Association |
APCs | = | antigen-presenting cells |
CHD | = | coronary heart disease |
CpG-ODN | = | cytosine-phosphate-guanosine oligodeoxynucleotides |
CTLA-4 | = | cytotoxic T lymphocyte-associated antigen 4 |
CXCR3 | = | Th1-associated chemokine receptor |
FDR | = | false discovery rate |
FoxP3 | = | fork-head box protein 3 |
GAPDH | = | glyceraldehyde 3-phosphate dehydrogenase |
GSEA | = | gene-set enrichment analysis |
GWEA | = | genome-wide expression array |
ICOS | = | inducible T cell co-stimulator |
IDO | = | indoleamine 2,3-dioxygenase |
IFN-γ | = | interferon gamma |
LDAs | = | TaqMan low-density arrays |
MHCII | = | class II major histocompatibility complex |
MsigDB | = | Molecular Signature Database |
TCR | = | T cell receptor |
Th1 | = | T helper cell type 1 |
TLR9 | = | Toll-like receptor 9 |
Treg | = | regulatory T cell |
Introduction
Atherosclerosis is a chronic inflammatory disease (Citation1,Citation2) regulated by a complex interplay of innate and adaptive immune responses (Citation3,Citation4). The immune system may protect from vascular inflammation by eliminating potentially harmful microbial agents and waste products such as modified lipoproteins and apoptotic fragments (Citation4). Characterization of a new atheroprotective immune mechanism is important for the development of novel preventive therapies against atherosclerosis and its complications, which are due to the plaque disruption and atherothrombosis (Citation1,Citation3–5).
In this study, we show the presence of indoleamine 2,3-dioxygenase (IDO), an intracellular enzyme expressed in antigen-presenting cells (APCs), in advanced atherosclerotic plaques. This enzyme is regulated by a complex array of immunological signals, and its main function is to promote degradation of an essential amino acid tryptophan to kynurenine. Consequently, this results in suppression of T cell activity by starvation due to decreased concentration of tryptophan in local microenvironments (Citation6–10). IDO-induced tryptophan degradation is a widely recognized defense mechanism regulating immunity, limiting growth of intracellular pathogens and proliferation of tumor cells (Citation9–12). However, the role of IDO or IDO-related tryptophan degradation pathway has been poorly investigated in patients with coronary heart disease (CHD). In a previous study, IDO activity, as measured by the kynurenine-to-tryptophan ratio, was increased, and as a consequence tryptophan degradation was increased in patients with CHD (Citation13). In another study, an oral load of L-tryptophan caused patients with myocardial infarction or angina pectoris to have higher kynurenine-to-tryptophan ratios as compared to controls, indicating higher tryptophan degradation in these patients (Citation14). In addition, we have shown an association of IDO activity with various risk factors for atherosclerosis in younger (Citation15) and middle-aged populations (Citation16), and also demonstrated up-regulation of IDO and IDO pathway genes in advanced atherosclerotic plaques by genome-wide expression analysis (GWEA) (Citation17).
To the best of our knowledge, the physical presence of either IDO or IDO-related tryptophan degradation-dependent T cell suppression pathways in human atherosclerotic plaques has not been characterized or reported previously. Previous studies have, however, elucidated atherosclerosis-related activation mechanisms between IDO, T cells, and APCs, especially in modulation of inflammatory responses (Citation18,Citation19) and cardiac allograft survival (Citation20–23).
In the present study, firstly we investigated the IDO mRNA and protein expression in advanced atherosclerotic lesions, and secondly characterized and verified the expression of the major components of the IDO-related pathway in advanced atherosclerotic tissues by quantitative RT-PCR, respectively. Thirdly, based on these results, we constructed a hypothetical model of the IDO-mediated tryptophan-dependent T cell suppression pathway in human advanced atherosclerosis and by using gene-set enrichment analysis (GSEA) tested whether the selected pathway genes function in a co-operating manner.
Material and methods
Vascular samples
Vascular samples from femoral artery, carotid artery, and aorta were obtained from 30 patients undergoing vascular surgery in the Division of Vascular Surgery as a part of the on-going Tampere Vascular Study. Control samples were taken from internal thoracic arteries obtained during normal coronary artery by-pass grafting from six patients in Tampere Heart Centre, Tampere University Hospital. The study has been approved by the Ethics Committee of Tampere University Hospital, and all participants gave signed informed consent. All clinical investigations were conducted according to the Declaration of Helsinki principles. The vascular samples were classified according to the recommendation of the American Heart Association (AHA) (Citation24).
RNA isolation and genome-wide expression analysis (GWEA)
The fresh tissue samples (n = 26) were immediately soaked to RNALater solution (Ambion Inc., Austin, TX, USA), and total-RNA was isolated with Trizol reagent (Invitrogen, Carlsbad, CA, USA) and RNAeasy Kit (Qiagen, Valencia, CA, USA). Concentration and quality of RNA was evaluated spectrophotometrically (BioPhotometer, Eppendorf, Wesseling-Berzdorf, Germany). Over 23,000 known and candidate genes were analyzed using Sentrix Human-8 Expression BeadChips according to the manufacturer's instructions (Illumina, San Diego, CA, USA). Approximately 250 genes were found to be differently expressed.
In brief, a 200 ng aliquot of total RNA from each sample was amplified to cDNA using Ambion's Illumina RNA Amplification kit according to instructions (cat. no I1755; Ambion, Inc.). Each sample of cRNA (1,500 ng) was hybridized to Illumina Sentrix® Human-8 Expression BeadChip arrays (Illumina, San Diego). Hybridized biotinylated cRNA was detected using 1 μg/mL Cyanine3-streptavidine (Amersham Biosciences, Pistacataway, NJ, USA). BeadChips were scanned with Illumina BeadArray Reader. The method has been described in more detail in our previous work in which GWEA was used to demonstrate up-regulation of IDO and IDO pathway genes in advanced atherosclerotic plaques (Citation17).
The accuracy of Illumina Sentrix® Human-8 Expression BeadChips microarray methodology for gene expression measurement was verified earlier by real-time quantitative TaqMan PCR in which expression of 20 genes with both methods was quantified with 95% concordance (Citation25).
Quantitative real-time PCR
From 30 tissue samples, gene expression analyses were performed with quantitative TaqMan low-density arrays (LDAs) (Applied Biosystems, Foster City, CA, USA). Total-RNA (500 ng) was reverse-transcribed to cDNA using High-Capacity cDNA Kit according to the manufacturer's instructions (Applied Biosystems). For the PCR, LDAs were loaded with 8 (μL undiluted cDNA, 42 μL H2O, and 50 μL PCR Universal Master Mix. PCR was performed according to the manufacturer's instructions (Applied Biosystems). Samples were analyzed as duplicates, and both cDNA synthesis and PCR reactions were validated for inhibition. Glyceraldehyde 3-phosphate dehydrogenase (GAPDH) was used as a housekeeping gene control and the qPCR results were analyzed using SDS 2.2 software (Applied Biosystems).
Immunohistochemistry
Immunohistochemistry was performed using the ABD-method (Vectastain Elite kit; Vector Laboratories, Burlingame, CA, USA) and paraffin-embedded vascular samples without any counterstain. IDO in vascular wall was detected with mouse monoclonal anti-human IDO antibody (AB9212; Upstate 05-840, Millipore, Billerica, MA, USA). The following primary antibodies were used to detect vascular cell markers in adjacent sections: Muscle actin (mouse anti-human muscle actin, clone HHF35; DakoCytomation, Glostrup, Denmark) was used to detect smooth muscle cells. Cluster of differentiation 68 (mouse anti-human CD68, clone PG-M1 (DakoCytomation)) was used as a marker of monocytes and macrophages. For the detection of endothelial cells, CD31 antibody (mouse anti-human CD31, endothelial cell, clone JC70A; DakoCytomation) was used.
The sections were subjected to microwave antigen retrieval treatment as described earlier (Citation26). Endogenous peroxidase activity was extinguished by treating the section with 0.3% H2O2 for 30 min. Subsequently, the sections were incubated overnight with the primary antibodies followed by biotinylated sheep anti-mouse (1:300; Amersham Int., Buckinghamshire, UK) and ABC-complex for 30 min. Diaminobenzidine was used as the chromogen. All antibodies were diluted in PBS containing 1% BSA and 0.3% of Triton X-100. Controls included omitting the primary antibody or replacing it with non-immune sera. No staining was seen in the controls. The sections were examined in a Nikon Microphot FXA microscope equipped with PCO Sensicam digital camera (PCO, Kelheim, Germany). The co-localization of IDO and CD68 was studied in 5 μm adjacent paraffin sections (mirror image sections). Sections were stained with ABC-method as described above.
Bioinformatics and statistical analysis
Raw intensity data obtained from the Illumina™ platform were normalized with R language and environment for statistical computing and related Bioconductor module. R related Bioconductor module was also used to conduct single-probe analysis including fold-change calculations and filtering the probes. Pathway analysis of the expression data was performed using the GSEA implemented in GSEA java desktop application version 2.0 and MsigDB (Molecular Signature Database) version 2.0. Before applying the GSEA, probes representing the same gene symbol in Illumina data were replaced with their maximum intensity in order to avoid duplicates in the analysis. In the GSEA we used self-constructed IDO pathway gene set and determined the function of these genes in a co-operated manner as recommended by Subramanian et al. (Citation27). Co-operation was considered statistically significant when false discovery rate (FDR) was less than 0.25. Otherwise, the data were analyzed with the SPSS software (version 14.00; SPSS Inc., IL, USA). The non-parametric Mann-Whitney U test was used for comparison of mRNA expression between atherosclerotic and control tissues, and between different arterial regions. Data are presented as mean ± SD unless otherwise stated. A P-value of less than 0.05 was considered statistically significant.
Results
Characteristics of the subjects and studied arterial samples
The median age of the subjects was 70.0 years and age range from 45 to 93 years; 75.9% of the subjects were men. The prevalence of risk factors were as follows: dyslipidemia 40.7%, hypertension 77.8%, diabetes 18.5%, history of smoking 86.2%, alcohol usage more than once a week 42.8%. According to the histological AHA classification 73.3% of the samples were classified as type V-VI advanced plaques.
IDO mRNA expression in the atherosclerotic tissue
We compared IDO expression level between atherosclerotic tissue (AHA types V-VI) and non-atherosclerotic control tissue, and expression levels between different arterial types. In each arterial bed IDO was up-regulated significantly compared to healthy controls (data not shown). For simplicity, arterial samples were pooled to demonstrate expression difference (). According to GWEA, the median IDO expression level in atherosclerotic tissues (n = 20) was 153 (119-185) and 115 (103-126) in non-atherosclerotic control tissues (n = 6) (1.3-fold relative to control, P < 0.0001 for difference, Mann-Whitney U test) (). The Illumina GWEA array results were verified using relative quantitative RT-PCR. In verified analysis, IDO mRNA expression was elevated 4.9-fold (P = 0.001) in atherosclerotic arteries relative to controls ().
Figure 1. Indoleamine 2,3-dioxygenase (IDO) expression in atherosclerotic and control artery samples. A: Gene expression value is the normalized average gene intensity for each group measured in the Illumina Expression BeadChips. Median IDO expression levels in atherosclerotic and non-atherosclerotic control tissues were 152.5 (119.1–185.1) and 114.8 (102.6–125.7), respectively. Mann-Whitney U test relative to control tissues. B–F: IDO immunoreactivity in human atherosclerotic plaques. Internal thoracic artery sample served as a control (B). In IDO staining, arrows point to IDO-positive macrophages. Also smaller cells (arrow-heads) are labeled (C). Staining with monocyte marker CD68 shows labeled macrophage (arrow) and monocytes (arrow-heads) (D), whereas labeled monocytes and macrophages could not be identified from smooth muscle cell marker HHF35 staining (E). No staining was seen with endothelial cell marker CD31 (F) in human carotid artery plaques. The stage of atherosclerosis was classified according to American Heart Association classification. 100× magnification.
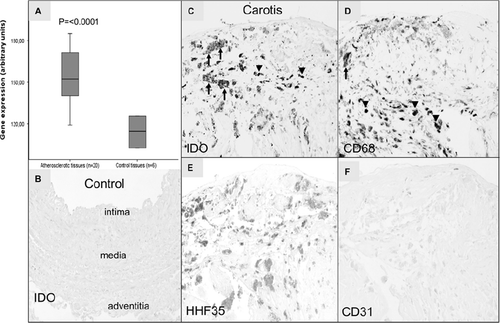
Table I. Indoleamine 2,3-dioxygenase (IDO) pathway related gene expression analysis using quantitative RT-PCR according to artery tissue type. The expression values are medians and numbers in parentheses interquartile ranges (q1–q3).
Immunohistochemistry of IDO
Immunohistochemistry of atherosclerotic lesions revealed that IDO is expressed in the atheromatous core of carotid artery plaques while only sparse cells were positive in control vessels (). In mirror image sections IDO immunoreactivities co-localized with CD68-positive cells ().
IDO-related pathway gene expression analysis
IDO pathway-related gene components were first searched and selected according to existing literature (). In the pathway analysis, the atherosclerotic tissues were compared with controls in respect of these components, first by using GWEA and after that by using quantitative RT-PCR analysis for verification of the results ().
IDO pathway component expression analysis () revealed that the fork-head box protein 3 (FoxP3) (4.7-fold expression as compared to control, P = 0.001), cytotoxic T lymphocyte-associated antigen 4 (CTLA-4) (4.7-fold, P = 0.001), CD28 (7.6-fold, P = 0.001), and inducible T cell co-stimulator (ICOS) (4.3-fold, P = 0.002) were all significantly up-regulated along with IDO-expression (4.9-fold, P = 0.001). In addition, their known counteracting surface molecules CD80 and CD86 were up-regulated 16.8-fold (P < 0.001) and 9.1-fold (P = 0.001), respectively, as compared to control tissues.
A signal transmission pair for T cell receptor (TCR), CD74 which is also known as class II major histocompatibility complex (MHCII) invariant polypeptide chain, had a 2.4-fold expression (P = 0.002) as compared to non-atherosclerotic tissues. Furthermore, the IDO expression-activating molecule interferon gamma (IFN-γ) had a 2.1-fold (P = 0.022) expression as compared to control tissues ().
Instead, no significant alterations were detected in TCR (0.9-fold, P = 0.543), CD25 (0.9-fold, P = 0.543) or Toll-like receptor 9 (TLR9) (1.0-fold, P = 0.885).
IDO-related pathway in atherosclerosis
In the GSEA analysis this self-constructed IDO pathway appeared to be highly significant (FDR < 0.07) in the atherosclerotic tissue according to the criteria (FDR < 0.25) recommended by Subramanian et al. (Citation27).
Furthermore, according to Spearman's correlation the Th1-associated chemokine receptor CXCR3 from GWEA correlated negatively (correlation coefficient -0.445, P = 0.049) with IDO expression suggesting that up-regulation of IDO suppresses Th1 cell activity in atherosclerotic patients.
On the basis of our expression results we constructed a hypothetical model of regulatory T cell (Treg)-driven pathways leading to the suppression of Th1 cell activity and possible inhibition of vascular atherosclerosis (, as a part of Discussion).
Figure 3. Hypothetical summary of the viable alternative options of indoleamine 2,3-dioxygenase (IDO) pathway components, through which signals may travel causing suppression of Th1 cells. The width of the arrows is in proportion to signal strength also expressed in folds. Utilized data is obtained from TaqMan low-density array. Statistical significances: *P < 0.05, **P < 0.01, ***P < 0.001 relative to control, Mann-Whitney U test. Statistics: The pathway analysis clearly indicated that the IDO pathway-forming gene set works highly significantly (FDR < 0.07) in a co-operating manner. (Abbreviations: APC = tolerogenic antigen-presenting cell; CD = cluster of differentiation; FDR = false discovery rate; FoxP3 = fork-head box protein 3; TLR9 = Toll-like receptor 9; TCR = T cell receptor; CTLA-4 = cytotoxic T lymphocyte-associated antigen 4; ICOS = inducible co-stimulatory molecule.)
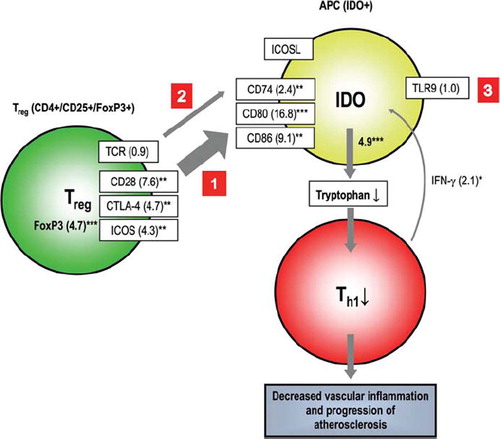
Discussion
In this study we demonstrated that IDO is present in advanced atherosclerotic plaques. Moreover, based on our results from GWEA and RT-PCR, we constructed a hypothetical Treg cell-initiated IDO-related pathway in atherosclerosis, presented in . In this Figure we describe three hypothetically viable gene expression pathways in advanced atherosclerotic plaques, which all may modify Th1 cell function. The suggested pathways are: 1) IDO activation via a CD28-CTLA-4-ICOS molecule complex, 2) IDO activation via TCR-CD74, and 3) TLR9-mediated IDO activation via naive APCs.
If activated, IDO-mediated pathways 1-3 in . may ultimately result in locally decreased tryptophan levels and subsequent starvation of Th1 cells. Irrespective of the activation route, the summary outcome of all these pathways could be decreased inflammation in the artery wall. The possible role of each of these hypothetical pathways in atherosclerosis is discussed in more detail in the following chapter.
IDO activation via a CD28-CTLA-4-ICOS route
A Tregcell FoxP3-initiated CD28, CTLA-4, and ICOS-driven pathway can potentially activate IDO expression by interacting with CD80/86 (, pathway 1) (Citation28–30). The essential role of transcription factor FoxP3 in Treg cells has been previously described (Citation31–33). Based on equal expression values on we speculate that CD28, CTLA-4, and ICOS could actually work simultaneously and synergistically as a single complex through which signals are transmitted to tolerogenic IDO+ APCs. This hypothesis is consolidated by the fact that in humans these three genes form a tightly linked cluster (Citation34), which suggests that their gene expression may be co-ordinately regulated (Citation28). On (pathway 1), gene activation folds from CD80 and CD86 (16.8- and 9.1-fold, respectively) demonstrate a significant enhancement compared to CD28, CTLA-4, and ICOS (7.6-fold, 4.7-fold, and 4.3-fold, respectively). Even though ICOS is not directly involved in contacting CD80 or CD86 (Citation35), it may nevertheless participate in synergistic amplification by assisting CD28 and CTLA-4 in signal transmission, thus causing accumulation of signals and increased gene activation. This hypothesis is supported by the possibility that CD28 and ICOS may share overlapping signaling pathways (Citation28).
All previously described events result in participation of IDO activation (4.9-fold), which is also affected by proinflammatory cytokine IFN-γ (2.1-fold), one of the key factors in the pathogenesis of atherosclerosis (Citation36). IFN-γ, secreted by activated Th1 cells, is probably regulated by a negative feedback loop since normal tryptophan-depleting function of IDO leads to suppression of Th1 lymphocytes. In addition, also IFN-γ secretion by macrophages has been described (Citation36–38), which could be explained by cytosine-phosphate-guanosine oligodeoxynucleotides (CpG-ODN)-directed binding to TLR9 that enhances IFN-γ production (, pathway 3) (Citation39).
IDO activation via a TCR-CD74 route
In the second hypothetical route, signal transmission propagates from TCR to CD74 (2.4-fold) (, pathway 2). To best of our knowledge, participation of TCR-CD74 in IDO activation has not been described previously, but since CD74 is a part of MHCII and therefore a major player in signal transmission between APCs and T cells, we hypothesize that this route may be important in IDO activation. It must also be emphasized that activated CD74 likely contributes to signals transmitted via CD80/86, which then amplify IDO activation in a synergistic manner.
TLR9-mediated IDO activation via naive APCs
The third hypothetical pathway for IDO activation affects APCs directly. This pathway is initiated by CpG-ODN binding to TLR9 on the surface of naive APC (Citation40), which then activates a signaling pathway for IDO promotion. In our study, TLR9 expression was insignificant (0.9-fold) in advanced atherosclerotic lesions, and therefore we speculated that this pathway does not play an important role in the regulation of IDO.
This conclusion is supported by previous studies in which only naive APCs are activated by this route (Citation40), even though some evidence for lesional IDO+ APC activation by naive APCs also exists (Citation40). Therefore, due to the limited amount of information the presence of TLR9 on IDO+ APCs cannot be totally excluded. It should also be noted that TLR9 activation initiates the production of a selection of proinflammatory cytokines and co-stimulatory molecules, including CD80 and CD86 (Citation40). It is therefore possible that TLR9 participate in IDO+ APC activation via some alternative, yet currently unknown, routes.
Genes in the IDO-related pathway function in co-operating manner
The pathway analysis clearly indicated that the IDO pathway-forming gene-set functions in a co-operating manner (FDR < 0.07). Based on previously published information of the genes involved in transmitting signals in the regulation of relationships between T cells and APCs, and on the basis of our expression results, we constructed a hypothetical model of Treg cell-driven pathways leading to suppression of T cell activity and possible inhibition of vascular atherosclerosis. The outcome demonstrates that expression results of the genes in this self-constructed IDO pathway presented in are not independent events but rather function in a co-operative and complex manner throughout the pathway.
Limitations of the study
The internal thoracic artery from coronary artery by-pass patients was used as a non-atherosclerotic control tissue. This artery type was selected since no microscopical signs of atherosclerosis could be detected. The collection of control arterial samples from healthy subjects is restricted by ethical reasons.
The most obvious limitation of our study was that we do not have a cell-specific localization of all IDO-related pathway markers. Further studies are warranted to clarify these issues.
There is also evidence that tryptophan-derived catabolites, such as kynurenine and anthranilic acid derivatives, play a role in modification of T cell activity by shifting the cytokine balance towards a Th2 pattern in activated invariant natural killer T (iNKT) cells (Citation41). These catabolites have been previously shown to have an impact on allograft survival in rat models (Citation42) but were not focused on further in the present study.
In addition, mechanistic insights regarding the presented hypothesis of the IDO pathway activation in atherosclerosis were not discussed in this article. To provide such data would require more specific functional studies in the form of in vitro experiments, which were impossible to organize with the present set-up. Since this is the first study to describe the IDO-related activation mechanism in atherosclerotic plaques, mechanistic investigations will be one of the main topics of the novel follow-up studies.
Clinical implications
Our present findings demonstrate the significance of IDO in atherosclerotic plaques and its central role in the APC-directed pathway controlling the progression of atherosclerosis. Based on these results, several potential therapeutic targets to intervene in this pathway exist. Traditionally, IFN-γ, being a strong up-regulator of IDO, has been presented as one of the key targets for therapeutic intervention (Citation43). Our results support a defined role for IFN-γ in atherosclerosis (Citation36) but at the same time indicate that its participation in the activation of IDO might be rather insignificant compared to the other genes. It should also be emphasized that the precise function of IFN-γ is yet to be elucidated since both pro- and antiatherogenic actions of this cytokine have been identified (Citation2,Citation36,Citation38). Similarly, due to the fact that IDO is affected by a wide array of signals from various genes, blocking one or some of these sources is not necessarily effective. Therefore, we conclude that in order to control IDO regulation and function it is necessary to develop a mechanism that directly and selectively affects IDO through activation or suppression. In fact, first experiments using gene delivery of IDO in a murine model support this assumption and show that IDO prolongs cardiac allograft survival by shaping the types of T cell responses (Citation20).
Conclusions
In humans IDO and IDO-related pathway are important mediators of the immunoinflammatory responses in advanced atherosclerosis offering previously uncharacterized viable therapeutic targets and possibilities for the development of novel antiatherogenic immunosuppressive therapies.
Acknowledgements
This study was supported with grants from the European Union 7th Framework Program, grant number 201668, AtheroRemo, Medical Research Fund of Tampere University Hospital, the Emil Aaltonen Foundation (T.L., P.N.), the Pirkanmaa Regional Fund of the Finnish Cultural Foundation, the Research Foundation of Orion Corporation, the Jenny and Antti Wihuri Foundation, and the Academy of Finland (Grant no. 104821). Niku Oksala was supported by grants from the Finnish Angiology association, Maire Taponen Foundation, and Paavo Nurmi Foundation.
The authors wish to thank Mrs Ulla Jukarainen and Nina Peltonen for their skilful technical assistance.
Declaration of interest: The authors report no conflicts of interest. The authors alone are responsible for the content and writing of the paper. Petri Niinisalo, Niku Oksala, and Mari Levula contributed equally.
References
- Ross R. Atherosclerosis—an inflammatory disease. N Engl J Med. 1999; 340:115–26.
- Hansson GK, Robertson AK, Söderberg-Nauclér C. Inflammation and atherosclerosis. Annu Rev Pathol. 2006; 1:297–329.
- Hansson GK, Libby P. The immune response in atherosclerosis: a double-edged sword. Nat Rev Immunol. 2006; 6:508–19.
- Nilsson J, Hansson GK. Autoimmunity in atherosclerosis: a protective response losing control? J Intern Med. 2008; 263:464–78.
- Lusis AJ, Mar R, Pajukanta P. Genetics of atherosclerosis. Annu Rev Genomics Hum Genet. 2004; 5:189–218.
- de la Maza LM, Peterson EM. Dependence of the in vitro antiproliferative activity of recombinant human gamma-interferon on the concentration of tryptophan in culture media. Cancer Res. 1988; 48:346–50.
- Hwu P, Du MX, Lapointe R, Do M, Taylor MW, Young HA. Indoleamine 2,3-dioxygenase production by human dendritic cells results in the inhibition of T cell proliferation. J Immunol. 2000; 164:3596–9.
- Terness P, Bauer TM, Röse L, Dufter C, Watzlik A, Simon H, . Inhibition of allogeneic T cell proliferation by indoleamine 2,3-dioxygenase-expressing dendritic cells: mediation of suppression by tryptophan metabolites. J Exp Med. 2002; 196:447–57.
- Mellor AL, Munn DH. Tryptophan catabolism and regulation of adaptive immunity. J Immunol. 2003; 170:5809–13.
- Mellor AL, Munn DH. IDO expression by dendritic cells: tolerance and tryptophan catabolism. Nat Rev Immunol. 2004; 4:762–74.
- Ozaki Y, Edelstein MP, Duch DS. Induction of indoleamine 2,3-dioxygenase: a mechanism of the antitumor activity of interferon gamma. Proc Natl Acad Sci U S A. 1988; 85:1242–6.
- Pfefferkorn ER. Interferon gamma blocks the growth of Toxoplasma gondii in human fibroblasts by inducing the host cells to degrade tryptophan. Proc Natl Acad Sci U S A. 1984; 81:908–12.
- Wirleitner B, Rudzite V, Neurauter G, Murr C, Kalnins U, Erglis A, . Immune activation and degradation of tryptophan in coronary heart disease. Eur J Clin Invest. 2003; 33:550–4.
- Rudzite V, Sileniece G, Liepina D, Dalmane A, Zirne R. Impairment of kynurenine metabolism in cardiovascular disease. Adv Exp Med Biol. 1991; 294:663–7.
- Pertovaara M, Raitala A, Juonala M, Lehtimäki T, Huhtala H, Oja SS, . Indoleamine 2,3-dioxygenase enzyme activity correlates with risk factors for atherosclerosis: the Cardiovascular Risk in Young Finns Study. Clin Exp Immunol. 2007; 148:106–11.
- Niinisalo P, Raitala A, Pertovaara M, Oja SS, Lehtimäki T, Kähönen M, . Indoleamine 2,3-dioxygenase activity associates with cardiovascular risk factors: The Health 2000 study. Scand J Clin Lab Invest. 2008; 68:1–4.
- Oksala N, Levula M, Airla N, Pelto-Huikko M, Ortiz RM, Järvinen O, . ADAM-9, ADAM-15, and ADAM-17 are upregulated in macrophages in advanced human atherosclerotic plaques in aorta and carotid and femoral arteries—Tampere Vascular Study. Ann Med. 2009; 41:279–90.
- Mahnke K, Bedke T, Enk AH. Regulatory conversation between antigen presenting cells and regulatory T cells enhance immune suppression. Cell Immunol. 2007; 250:1–13.
- Boasso A, Herbeuval JP, Hardy AW, Winkler C, Shearer GM. Regulation of indoleamine 2,3-dioxygenase and tryptophanyl-tRNA-synthetase by CTLA-4-Fc in human CD4+ T cells. Blood. 2005; 105:1574–81.
- Yu G, Dai H, Chen J, Duan L, Gong M, Liu L, . Gene delivery of indoleamine 2,3-dioxygenase prolongs cardiac allograft survival by shaping the types of T-cell responses. J Gene Med. 2008; 10:754–61.
- Li M, Zhang X, Zheng X, Lian D, Zhang ZX, Sun H, . Tolerogenic dendritic cells transferring hyporesponsiveness and synergizing T regulatory cells in transplant tolerance. Int Immunol. 2008; 20:285–93.
- Thebault P, Condamine T, Heslan M, Hill M, Bernard I, Saoudi A, . Role of IFNgamma in allograft tolerance mediated by CD4+CD25+ regulatory T cells by induction of IDO in endothelial cells. Am J Transplant. 2007; 7:2472–82.
- Li J, Meinhardt A, Roehrich ME, Golshayan D, Dudler J, Pagnotta M, . Indoleamine 2,3-dioxygenase gene transfer prolongs cardiac allograft survival. Am J Physiol Heart Circ Physiol. 2007; 293:H3415–23.
- Stary HC, Chandler AB, Dinsmore RE, Fuster V, Glagov S, Insull W Jr, . A definition of advanced types of atherosclerotic lesions and a histological classification of atherosclerosis. A report from the Committee on Vascular Lesions of the Council on Arteriosclerosis, American Heart Association. 1995; 92:1355–74.
- Laaksonen R, Katajamaa M, Päivä H, Sysi-Aho M, Saarinen L, Junni P, . A systems biology strategy reveals biological pathways and plasma biomarker candidates for potentially toxic statin-induced changes in muscle. PLoS One. 2006; 1:e97.
- Shi SR, Key ME, Kalra KL. Antigen retrieval in formalin-fixed, paraffin-embedded tissues: an enhancement method for immunohistochemical staining based on microwave oven heating of tissue sections. J Histochem Cytochem. 1991; 39:741–8.
- Subramanian A, Tamayo P, Mootha VK, Mukherjee S, Ebert BL, Gillette MA, . Gene set enrichment analysis: a knowledge-based approach for interpreting genome-wide expression profiles. Proc Natl Acad Sci U S A. 2005; 102:15545–50.
- Carreno BM, Collins M. The B7 family of ligands and its receptors: new pathways for costimulation and inhibition of immune responses. Annu Rev Immunol. 2002; 20:29–53.
- Collins M, Ling V, Carreno BM. The B7 family of immune-regulatory ligands. Genome Biol. 2005; 6:223.
- Greenwald RJ, Freeman GJ, Sharpe AH. The B7 family revisited. Annu Rev Immunol. 2005; 23:515–48.
- Fontenot JD, Gavin MA, Rudensky AY. Foxp3 programs the development and function of CD4+CD25+ regulatory T cells. Nat Immunol. 2003; 4:330–6.
- Nik Tavakoli N, Hambly BD, Sullivan DR, Bao S. Forkhead box protein 3: Essential immune regulatory role. Int J Biochem Cell Biol. 2008; 40:2369–73.
- Mallat Z, Ait-Oufella H, Tedgui A. Regulatory T-cell immunity in atherosclerosis. Trends Cardiovasc Med. 2007; 17:113–8.
- Ling V, Wu PW, Finnerty HF, Agostino MJ, Graham JR, Chen S, . Assembly and annotation of human chromosome 2q33 sequence containing the CD28, CTLA4, and ICOS gene cluster: analysis by computational, comparative, and microarray approaches. Genomics. 2001; 78:155–68.
- Yoshinaga SK, Whoriskey JS, Khare SD, Sarmiento U, Guo J, Horan T, . T-cell co-stimulation through B7RP-1 and ICOS. Nature. 1999; 402:827–32.
- Harvey E, Ramji DP. Interferon-gamma and atherosclerosis: pro- or anti-atherogenic? Cardiovasc Res. 2005; 67:11–20.
- Schroder K, Hertzog PJ, Ravasi T, Hume DA. Interferon-gamma: an overview of signals, mechanisms and functions. J Leukoc Biol. 2004; 75:163–89.
- Schroecksnadel K, Fuchs D. Interferon-gamma for counteracting T-cell activation. Trends Immunol. 2006; 27:398.
- Krieg AM, Kline JN. Immune effects and therapeutic applications of CpG motifs in bacterial DNA. Immunopharmacology. 2000; 48:303–5.
- Fallarino F, Puccetti P. Toll-like receptor 9-mediated induction of the immunosuppressive pathway of tryptophan catabolism. Eur J Immunol. 2006; 36:8–11.
- Molano A, Illarionov PA, Besra GS, Putterman C, Porcelli SA. Modulation of invariant natural killer T cell cytokine responses by indoleamine 2,3-dioxygenase. Immunol Lett. 2008; 117:81–90.
- Bauer TM, Jiga LP, Chuang JJ, Randazzo M, Opelz G, Terness P. Studying the immunosuppressive role of indoleamine 2,3-dioxygenase: tryptophan metabolites suppress rat allogeneic T-cell responses in vitro and in vivo. Transpl Int. 2005; 18:95–100.
- Leon ML, Zuckerman SH. Gamma interferon: a central mediator in atherosclerosis. Inflamm Res. 2005; 54:395–411.