Abstract
Background. An increase in total peripheral resistance (TPR) usually increases arterial wave reflection. During passive head-up tilt (HUT), however, arterial wave reflection decreases with increasing TPR. This study addressed whether arterial wave reflection gradually decreases during HUT. Methods. In 10 healthy volunteers (22–39 years, nine males), we recorded finger arterial pressures in supine position (0°), and 30° and 70° degrees HUT and active standing (90°). Aortic pressure was constructed from the finger pressure signal and hemodynamics were calculated. Arterial wave reflection was quantified as the augmentation index (AIx) and the reflection magnitude (RM). Results. During HUT, heart rate increased (p < 0.001), stroke volume and cardiac output decreased (p < 0.001 and p < 0.01), diastolic blood pressure increased (p < 0.001), whereas systolic blood pressure did not change. TPR increased from 0.9 dyn s/cm5 at 0° to 1.2, 1.4 and 1.4 dyn s/cm5 at 30°, 70° and 90° (p < 0.001). AIx fell gradually from 25% at 0° to 16%, −1% and −10% at 30°, 70° and 90° (p < 0.001). The RM decreased from 0.572 at 0° to 0.456 at 90° (p < 0.001). Conclusion. From supine to upright, arterial wave reflection represented as AIx and RM gradually decreases in the presence of increasing TPR.
Introduction
The augmentation index (AIx) is used as a marker of arterial stiffness and arterial wave reflection and has been shown to predict the survival of end-stage renal disease patients (Citation1) and cardiovascular events in coronary patients (Citation2). There is, however, discussion over whether the AIx primarily reflects arterial stiffness. Pulse wave velocity (PWV) is a more direct measure of arterial stiffness and is considered the gold standard of non-invasive arterial stiffness measurements (Citation3). Both PWV and AIx increase with age, but the AIx seems to reach a plateau around 60 years (Citation4). So, the AIx is only partially determined by arterial stiffness. The other two factors that influence the AIx are heart rate and the time of return of the reflected wave. The AIx has a negative linear relation with heart rate (Citation5) and is therefore usually presented after correction for a heart rate of 75 beats/min.
The location and the contribution of the site of reflection to the AIx have been more difficult to establish. Besides major bifurcations, the main reflection sites are thought to be high-resistance small arteries and arterioles (Citation6,Citation7). Indeed, angiotensin II or noradrenalin-induced vasoconstriction cause an increase in AIx and total peripheral resistance (TPR) (Citation8), whereas vasodilators, such as nitroprusside and calcium-channel blockers lower AIx and TPR (Citation9). These pharmacologically induced changes in arterial wave reflection are independent of arterial stiffness (Citation10).
Thus an increase in TPR usually causes an increase in arterial wave reflection as quantified by the AIx. AIx and TPR, however, do not always react in parallel. It has been shown that passive head-up tilt (HUT) of 60° decreased the AIx compared with supine position while increasing TPR (Citation11,Citation12). Humans usually are not in supine position for the majority of the day and arterial wave reflection decreases during HUT of 60° but it is unknown at which HUT angle arterial wave reflection is minimal. We therefore studied AIx and TPR during different angles of passive tilt and active standing. A second aim of the study was to disentangle the contribution of forward and backward waves during HUT and standing.
Methods
Study subjects and design
We studied 10 healthy volunteers, aged 22–39 years, nine were male. The participants were non-smokers, used no medication and had no history of orthostatic fainting. Informed consent was obtained from all participants and the study was approved by the ethics committee of Copenhagen (KF01-120/96) and performed in accordance with the Declaration of Helsinki. The study protocol has been described previously (Citation13). In short, after instrumentation the subjects rested in supine (0°) position for 30 min. Participants were subjected to a passive tilt (with foot support) protocol, which included 30° and 70° HUT and an active standing (90°) period, all preceded and followed by a period of supine rest (0°) to minimize the crossover effect of tilt. We used the first supine period as a baseline measurement and compared the different tilt angles with this baseline measurement.
Hemodynamics
Non-invasive finger arterial pressure was recorded with a TNO Finapres Model 5, sampled at 100 Hz and analyzed off-line. We selected 20 consecutive beats before and 1 min after each change body position for analysis. From the beat selection, an aortic pressure was constructed with a generalized pressure transfer filter (Citation14) to obtain beat-to-beat systolic blood pressure (SBP), diastolic blood pressure (DBP), pulse pressure (PP) and mean arterial pressure (MAP). Subsequently aortic stroke volume (SV) was calculated with a pulse contour method using the three-element Windkessel model (Citation15,Citation16). Heart rate (HR) was the inverse of the interbeat interval. Cardiac output (CO) was the product of SV and HR, and TPR is MAP divided by CO.
Arterial wave reflection
Arterial wave reflection was quantified from the pressure signal as the AIx, defined as the ratio of augmented pressure and the pulse pressure. Also, the constructed aortic pressure was separated into forward (Pf) and backward (Pb) waves by waveform analysis as described by Westerhof and colleagues (Citation17). For wave separation, the flow wave from the Windkessel was used. This allows the calculation of the reflection magnitude (RM) as the ratio of Pb and Pf. Calculations were performed in Mathematica (Wolfram Research Inc., Mathematica, Version 4, Champaign, IL, USA).
Statistical analysis
Differences in hemodynamics and arterial wave reflection between supine and standing position and the different angles of tilt were calculated using Friedman's ANOVA. The outcomes of different angles of tilt and standing were post hoc compared with supine with Wilcoxon signed rank test. Data are expressed as median. A p-value < 0.05 was considered significant. Data were analyzed using SPSS software version 16.0.1 (SPSS Inc., Chicago, IL, USA).
Results
Hemodynamic and arterial wave reflection data are shown in and in and . Compared with supine position, aortic DBP and HR increased, whereas SV and CO decreased during stepwise increased angles of passive HUT and active standing. Aortic SBP did not significantly change. TPR increased from 0.9 dyn s/cm5 at 0° to 1.2, 1.4 and 1.4 dyn s/cm5 at 30°, 70° and 90° (p < 0.001). AIx fell from 25% at 0° to 16, − 1 and − 10% at 30°, 70° and 90° (p < 0.001). The RM also decreased from 0.572 at 0° to 0.551, 0.469 and 0.456 at 30°, 70° and 90° (p < 0.001). In multivariate analyses heart rate was an important contributor of AIx or RM (beta for heart rate −0.66, p < 0.001 and −0.65, p < 0.001 respectively), but the tilt angle remained a significant and independent predictor of AIx or RM after correction for heart rate (beta for tilt angle −0.27, p = 0.02 and −0.31, p < 0.01).
Figure 1. Hemodynamics during different angles of tilt and standing. Data shown are mean ± standard error (SE) for aortic systolic blood pressure (SBP), diastolic blood pressure (DBP), heart rate (HR), stroke volume (SV), cardiac output (CO), total peripheral resistance (TPR).

Figure 2. Arterial wave reflection during different angles of tilt and standing. Data shown are mean ± standard error (SE) for augmentation index (AIx) and reflection magnitude (RM).
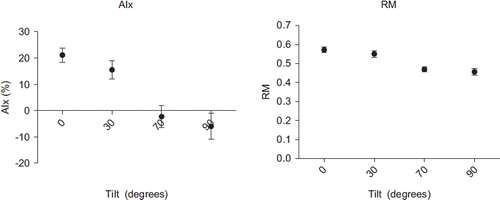
Table I. Hemodynamic and arterial wave reflection data.
shows the average measured aortic pressures and the derived forward and backward pressure. The amplitude of the forward pressure waves increased from supine to vertical postural position, while the backward waves decrease. The measured pressure wave, which is the sum of Pf and Pb, does not significantly change in amplitude, but because of the decrease in arterial wave reflection the shape of the pressure wave does change from a Murgo type A to a type C (Citation18).
Discussion
In this study, we show in a group of healthy subjects that from supine to upright the AIx and RM decrease gradually and from the onset on, while TPR increases in response to increasing angles of passive HUT and active standing. The arterial wave reflection was lowest and TPR highest in the standing position.
Tahvanainen and colleagues have shown that the AIx compared with supine position reproducibly decreases upon 60° HUT independent of age (Citation11,Citation12). Our study extends these findings by showing that there is a stepwise decrease in AIx during tilting and that this response is also present with active standing.
The AIx response upon tilting and standing seems counterintuitive, since usually an increase in TPR coincides with an increase in arterial wave reflection (Citation8,Citation19,Citation20). We will briefly discuss the current concept of arterial wave reflection as used to describe the behavior of forward and backward waves in supine position and we will propose an explanation for our contrasting results after postural change. At any place in the arterial system, the arterial pressure wave is the resultant of a forward and a backward pressure wave. The forward pressure wave is formed by contraction of the left ventricle against the arterial load in the proximal aorta. The forward wave travels down the elastic large arteries, the aorta and its main branches, where at places of impedance mismatch the wave reflects and travels back to the heart. The backward wave that arrives in the proximal aorta is the resultant of all individual reflecting waves and augments the forward pressure wave to form the pressure wave as “seen” by the heart. The main sites of reflection are thought to be high-resistance arteries and arterioles (Citation6,Citation7). Indeed, pharmacologically induced vasoconstriction increases TPR and arterial wave reflection (Citation8), whereas vasodilation decreases TPR and arterial wave reflection (Citation9). In response to passive HUT or active standing, the postural change causes a gravitational shift of blood to the lower body. This pooling of blood causes a decrease in cardiac preload, which in turn reduces cardiac output. To counteract the initial drop in blood pressure, the baroreflex increases vasomotor tone by an increase in sympathetic output, which accelerates HR and increases TPR (Citation21). Since small resistance arteries and arterioles are thought to be the major reflection site of arterial pressure waves, the higher vasomotor tone is thought to increase arterial wave reflection. Sympathetic nervous system-induced increases in vascular resistance by cold pressure test (Citation19) or isometric fatiguing handgrip (Citation20) have shown to increase arterial wave reflection. Clearly other factors influence arterial wave reflection as well, since a decrease in arterial wave reflection and a concomitant increase in TPR during HUT has previously been shown (Citation11,Citation12). It is difficult to disentangle all the anatomical, functional and hemodynamic changes upon postural change and its relation to arterial wave reflection. The change in body position per se could have an effect on arterial diameter and function and could thereby influence arterial wave reflection. Lower body negative pressure (LBNP) mimics the hemodynamic response to HUT, but does this without changing body position. Comparable with the response to tilting, LBNP causes a decrease in SV, an increase in HR and TPR and also causes a decrease in arterial wave reflection (Citation20). So, changes in body position alone cannot fully explain the decrease in arterial wave reflection. Instead, the baroreflex mediated increase in sympathetic outflow upon postural change, and leads to vasoconstriction of resistance arteries but this might not be the case for all arterial beds. As part of the fright and flight response, catecholamines cause beta2 receptor-dependent dilation of arterioles of the skeletal musculature. It could be that arterial wave reflection during passive tilting and active standing is partially dependent on these beta2 receptor-dependent arterioles. In line with this is the observation that stimulation of the beta2 receptor with salbutamol in supine position causes an endothelium-dependent vasodilation and subsequently a decrease in arterial wave reflection (Citation22). Alternatively, the shift of blood volume to the lower body upon tilting or standing might cause the aorta and lower arteries to expand leading to alterations in impedance mismatch and decreased arterial wave reflection. Finally, the decrease in SV might influence the forward pressure wave and thereby influence arterial wave reflection. However, the principal differences in the pressure wave were observed in the backward wave. Furthermore, we found no relation between SV and AIx (data not shown). Therefore, it is unlikely that the observed decrease in SV could explain the decrease in arterial wave reflection.
A limitation of our study is that we did not correct the AIx for the increase in HR with an earlier proposed method (Citation5). We did, however, correct for heart rate in a multivariate analysis and found that changes in arterial wave reflection during tilting remain significant after correction for changes in HR. We are therefore convinced that the decrease in arterial wave reflection is not solely caused by the increase in HR. Also, we studied young healthy volunteers making it difficult to extrapolate our findings to older subjects or patients with cardiovascular risk factors or previous cardiovascular disease. Nevertheless, the AIx response to HUT does not significantly differ between younger and older persons (Citation12). Finally, we used a model to derive aortic flow to be able to perform wave separation, and not directly measure aortic flow, which will have introduced errors.
In conclusion, we have shown that from supine to upright arterial wave reflection decreases gradually with a lowest value in the standing position.
Perspectives
Whether this response is different in specific patient groups and whether this differentially affects central blood regulation needs further study. The reaction of arterial wave reflection and thereby central blood pressure upon standing might be different for patients with a disturbed baroreflex, with increased arterial stiffness or with an activated sympathetic system, such as heart failure patients. Arterial wave reflection is normally assessed in supine position and it is thought that increased arterial wave reflection augments central systolic pressure thereby increasing left ventricular load, which might cause an increase in left ventricular mass. Since humans usually spend most of their time in an upright position it is relevant to know whether organ damage such as left ventricular mass is associated with the AIx during tilting or standing and whether this relation is different from supine measured AIx.
Disclosures
Berend E. Westerhof is an employee and owns shares of the BMEYE company. The BMEYE company develops, manufactures and markets monitors for non-invasive hemodynamic monitoring.
References
- London GM, Blacher J, Pannier B, Guerin AP, Marchais SJ, Safar ME. Arterial wave reflections and survival in end-stage renal failure. Hypertension. 2001;38:434–438.
- Weber T, O'Rourke MF, Lassnig E, Porodko M, Ammer M, Rammer M, . Pulse waveform characteristics predict cardiovascular events and mortality in patients undergoing coronary angiography. J Hypertens. 2010;28:797–805.
- Laurent S, Cockcroft J, Van BL, Boutouyrie P, Giannattasio C, Hayoz D, . Expert consensus document on arterial stiffness: Methodological issues and clinical applications. Eur Heart J. 2006;27:2588–2605.
- McEniery CM, Yasmin, Hall IR, Qasem A, Wilkinson IB, Cockcroft JR. Normal vascular aging: Differential effects on wave reflection and aortic pulse wave velocity: The Anglo-Cardiff Collaborative Trial (ACCT). J Am Coll Cardiol. 2005; 46:1753–1760.
- Wilkinson IB, MacCallum H, Flint L, Cockcroft JR, Newby DE, Webb DJ. The influence of heart rate on augmentation index and central arterial pressure in humans. J Physiol. 2000; 525:263–270.
- O'Rourke MF. Vascular impedance in studies of arterial and cardiac function. Physiol Rev. 1982;62:570–623.
- Hashimoto J, Ito S. Some mechanical aspects of arterial aging: Physiological overview based on pulse wave analysis. Ther Adv Cardiovasc Dis. 2009;3:367–378.
- Wilkinson IB, MacCallum H, Hupperetz PC, van Thoor CJ, Cockcroft JR, Webb DJ. Changes in the derived central pressure waveform and pulse pressure in response to angiotensin II and noradrenaline in man. J Physiol. 2001;530:541–550.
- Noda T, Yaginuma T, O'Rourke MF, Hosoda S. Effects of nifedipine on systemic and pulmonary vascular impedance in subjects undergoing cardiac catheterization. Hypertens Res. 2006;29:505–513.
- Kelly RP, Millasseau SC, Ritter JM, Chowienczyk PJ. Vasoactive drugs influence aortic augmentation index independently of pulse-wave velocity in healthy men. Hypertension. 2001;37:1429–1433.
- Tahvanainen A, Koskela J, Tikkakoski A, Lahtela J, Leskinen M, Kahonen M, . Analysis of cardiovascular responses to passive head-up tilt using continuous pulse wave analysis and impedance cardiography. Scand J Clin Lab Invest. 2009;69: 128–137.
- Tahvanainen A, Leskinen M, Koskela J, Ilveskoski E, Nordhausen K, Oja H, . Ageing and cardiovascular responses to head-up tilt in healthy subjects. Atherosclerosis. 2009;207:445–451.
- Westerhof BE, Gisolf J, Karemaker JM, Wesseling KH, Secher NH, van Lieshout JJ. Time course analysis of baroreflex sensitivity during postural stress. Am J Physiol Heart Circ Physiol. 2006;291:H2864-H2874.
- Stok WJ, Westerhof BE, Karemaker JM. Changes in finger-aorta pressure transfer function during and after exercise. J Appl Physiol. 2006;101:1207–1214.
- Wesseling KH, Jansen JR, Settels JJ, Schreuder JJ. Computation of aortic flow from pressure in humans using a nonlinear, three-element model. J Appl Physiol. 1993;74:2566–2573.
- Jellema WT, Wesseling KH, Groeneveld AB, Stoutenbeek CP, Thijs LG, van Lieshout JJ. Continuous cardiac output in septic shock by simulating a model of the aortic input impedance: A comparison with bolus injection thermodilution. Anesthesiology. 1999;90:1317–1328.
- Westerhof BE, Guelen I, Westerhof N, Karemaker JM, Avolio A. Quantification of wave reflection in the human aorta from pressure alone: A proof of principle. Hypertension. 2006;48:595–601.
- Murgo JP, Westerhof N, Giolma JP, Altobelli SA. Aortic input impedance in normal man: Relationship to pressure wave forms. Circulation. 1980;62:105–116.
- Casey DP, Braith RW, Pierce GL. Changes in central artery blood pressure and wave reflection during a cold pressor test in young adults. Eur J Appl Physiol. 2008;103:539–543.
- Lydakis C, Momen A, Blaha C, Herr M, Leuenberger UA, Sinoway LI. Changes of elastic properties of central arteries during acute static exercise and lower body negative pressure. Eur J Appl Physiol. 2008;102:633–641.
- Fu Q, Shook RP, Okazaki K, Hastings JL, Shibata S, Conner CL, . Vasomotor sympathetic neural control is maintained during sustained upright posture in humans. J Physiol. 2006; 577:679–687.
- Hayward CS, Kraidly M, Webb CM, Collins P. Assessment of endothelial function using peripheral waveform analysis: A clinical application. J Am Coll Cardiol. 2002;40:521–528.