Abstract
Resistant hypertensive (RHTN) patients have endothelial dysfunction and aldosterone excess, which contribute to the development of resistance to antihypertensive treatment and cardiovascular complications. Biophysical forces within the arterial wall provide functional regulation of arterial stiffness. Carotid–femoral pulse wave velocity (PWV) and flow-mediated brachial artery dilation (FMD) can be used to evaluate vascular stiffness and endothelial function. Although both techniques have been used in several studies in hypertensive patients, it is unknown whether endothelial dysfunction is also associated with vascular stiffness in RHTN patients. Methods. One hundred and ninety-three consecutive subjects were divided in three groups: 44 RHTN, 35 well-controlled hypertensive patients (HTN) and 25 normal healthy volunteers (NT). FMD was measured by high-resolution ultrasound and PWV was calculated from measurements of the pulse transit time and the distance traveled by the pulse between carotid and femoral arteries. Results. No significant differences were observed in respect to body mass index, age or other biochemical variables among the three groups. FMD (NO-dependent) values were statistically different when comparing RHTN and well controlled HTN patients (respectively, 8.3 ± 4.7% and 10.1 ± 5.9%) and 12.3 ± 6.3% in normal subjects (p < 0.05). One-way analysis of variance (ANOVA) showed a significant difference in BP-adjusted PWV between RHTN and HTN (13.9 ± 1.0 and 11.5 ± 1.1 m/s, respectively; p < 0.05). FMD (NO-dependent) and PWV-adjusted values were strongly correlated in well-controlled HTN and NT subjects (r = − 0.74 and − 0.83, respectively). Although statistically significant, this correlation was lower in RHTN patients (r = − 0.43). Conclusion. We found a close relationship among high BP levels, endothelial dysfunction and vascular rigidity in hypertensive patients, demonstrated by a significantly higher increase in carotid–femoral PWV and a decrease in brachial artery FMD in RHTN when compared with well-controlled hypertensive patients. Although this study was not designed to test the prognostic, the vascular damage differences observed between patients with controlled vs uncontrolled hypertension suggest that the latter group may have a worse cardiovascular prognosis, requiring prospective assessment tests.
Introduction
Resistant hypertension (RHTN) is defined as a systolic (SBP) or a diastolic blood pressure (DBP) that remains above goal, despite adherence to treatment with full doses of at least three antihypertensive medications, including one diuretic (Citation1). RHTN patients have a higher risk of complications including stroke, acute aortic dissection, myocardial infarction, congestive heart failure and renal failure when compared with other types of hypertensive patients, such as those with responder, false resistant and masked hypertension (Citation2–4). In these patients, an imbalance between the endothelial production of vasodilating and vasoconstricting substances and aldosterone excess contribute to the development of resistance to antihypertensive treatment, cardiovascular complications and chronic kidney disease (Citation5–7). The endothelial dysfunction observed in hypertension is likely to be a consequence of high blood pressure (Citation8–10) and, possibly, facilitates the maintenance of elevated peripheral resistance associated with increased arterial stiffness in late stages of the disease, favoring the occurrence of several complications (Citation11,Citation12). Large artery stiffness depends on three main factors: structural elements within the arterial wall, such as elastin and collagen, distending pressure and vascular smooth muscle tone. Changes in smooth muscle tone alter the distribution of forces within the arterial wall, providing functional regulation of arterial stiffness (Citation13). Experimental studies have shown that the removal of the vascular endothelium alters arterial elasticity in animals (Citation14). Conversely, Schmitt et al. (Citation15) recently demonstrated that blocking nitric oxide (NO) synthesis increases local arterial stiffness, suggesting that endothelium-derived NO contributes to the regulation of large artery elasticity (Citation15). Arterial stiffening has the direct effect of decreased aortic compliance (Citation16). Simultaneously, there is an indirect effect with increased arterial stiffness increasing the speed of pressure waves travelling along the walls of the aorta and other major arteries. Thus, an increased pulse wave velocity (PWV) is indicative of arterial stiffness (Citation17). The long-established technique to measure the PWV using carotid–femoral PWV, as described by Young in 1804 and by Bramwell & Hill in 1922, is usually still considered the gold standard to evaluate central arterial stiffness (Citation18,Citation19). Carotid–femoral PWV has been used in epidemiological studies to show the predictive value of aortic stiffness for cardiovascular events (Citation20–22). Endothelium NO-dependent and independent vasodilatation can be assessed by ultrasound examination of the brachial artery, with changes in vessel diameter serving as an indicator of endothelial function (Citation23,Citation24). Although both techniques have been used in several studies in hypertensive patients, it is unknown whether endothelial dysfunction is also associated with vascular stiffness in RHTN patients.
The current study was designed to compare and correlate endothelial function assessed by flow-mediated dilation (FMD) and arterial wall stiffness evaluated by PWV in resistant and well- controlled hypertensive patients and in normal healthy subjects.
Methods
Study population
One hundred and ninety-three consecutive subjects referred to our specialized tertiary hypertension center as “resistant hypertensive patients” (RHTN) were submitted for screening based on AHA Statement for RHTN (Citation1). All patients had complete case histories and underwent physical examinations, electrocardiograms and laboratory tests. Patients with secondary forms of hypertension, impaired renal function, ischemic heart disease, liver disease, strokes, peripheral vascular disease, dyslipidemia, diabetes, smokers or with any other major diseases were excluded. They were followed and treated for a period of approximately 6 months with scheduled appointments on a regular basis before being considered resistant to treatment.
This study comprised 104 subjects divided into three groups: 44 resistant hypertensive (RHTN – 12 males and 32 females, mean age 52.6 ± 9.0 years), 35 well-controlled hypertensive patients (HTN – seven males and 28 females, mean age 53.8 ± 12.3 years), according to the VII JNC guidelines (Citation25), and 25 normal healthy volunteers (NT – seven males and 18 females, mean age 50.5 ± 11.9 years) from a hospital outpatient clinic. The right arm blood pressure (SBP, DBP and mean blood pressure) was measured during the morning by a trained healthcare professional with the patient in the seated position and using an appropriate cuff size.
Exclusion criteria included the presence of ischemic heart disease, valvular disease, heart failure, significant arrhythmia, dyslipidemia, diabetes mellitus and evidence of hepatic, renal or hematological dysfunctions and the use of drugs such as statins, prostaglandin inhibitors, vitamins, contraceptives that affect vascular function and acetylsalicylic acid.
RHTN patients took a mean of 3.6 medications daily. During optimization of the therapeutic regime, the most frequently prescribed medications were diuretics (94.8%): calcium-channel blockers (CCBs) (84.6%), angiotensin II receptor blockers (ARBs) (79.5%), angiotensin-converting enzyme inhibitors (ACEIs) (25.6%) and beta-blockers (53.8%). HTN patients took a mean of 2.5 prescribed medications daily, as follows: diuretics (93.7%), CCBs (28.1%), ARBs (31.2%), ACEIs (40.6%) and beta-blockers (46.9%).
This study was approved by the Research Ethics Committee of the Faculty of Medical Sciences, University of Campinas (Campinas, Brazil) and each participant provided signed written consent.
Study protocol
Flow-mediated dilation technique. Brachial artery dilation was measured by ultrasound, using a high-resolution linear vascular transducer (7–12 MHz, Toshiba Powervision 6000, Tokyo, Japan) coupled to a computer-assisted analysis software and an automated brachial analyzer software device (MIA, LLC, Coralville, IA, USA).
Initially, the brachial artery responses to endothelial-dependent (FMD) and independent (glyceryl-trinitrate [GTN]-mediated) stimuli were determined using a modification of the technique described by Celermajer et al. (Citation23), and following the guidelines of the International Brachial Artery Reactivity Task Force published in 2002 by Corretti et al. (Citation26). The subjects rested quietly for 15 min before the first scan and remained in the supine position throughout the study. The brachial artery was scanned longitudinally 5–10 cm above the elbow. When a satisfactory position had been found, a special probe holder was fixed around the arm to secure the ultrasound transducer in the same position for all measurements (Citation10). A polyurethane cuff (Hokanson, Inc., Bellevue, WA, USA), placed around the arm above the transducer, was inflated under a pressure of 250 mmHg for 5 min and then rapidly deflated. The resulting FMD, i.e. the increased brachial artery diameter, was recorded from 45 to 120 s after cuff deflation. Changes in the brachial artery diameter were expressed as the percentage change relative to the vessel diameter immediately before cuff inflation. The brachial artery was then allowed to recover for 10–15 min, after which another baseline scan was taken. The response to GTN was used as a measurement of endothelium-independent vasodilation. After recording the second baseline scan, 0.4 mg of GTN (Nitrostat, Parke-Davis, Morris-Plains, NJ, USA) was given sublingually and 4–5 min later, the brachial artery was imaged. The brachial artery diameter response to GTN was expressed as the percentage change relative to the vessel diameter immediately before drug administration.
Pulse wave velocity. PWV was calculated from measurements of the pulse transit time and the distance traveled by the pulse between two recording sites: PWV = distance (meters)/transit time (in seconds). Among the different signals for measuring PWV (Doppler, pressure, diameter), pressure signals recorded with a pressure-sensitive transducer are the most commonly used. For automatic measurement of PWV, pressure waveforms are digitized at different rates, according to the distance between the recording sites; the sampling acquisition frequency is 500 Hz for carotid–femoral PWV and 800 Hz for other recording sites, including carotid–radial PWV. The two pressure waveforms are stored in a recirculating memory buffer. Preprocessing analyses automatically adjusts the gain of each waveform for an equality of the two signals. A maximum of 588 data points per waveform are displayed at any given time, i.e. the display will cover a period from 0.735 to 1.47 s. This is sufficient always to capture at least one complete cardiac pressure upstroke (Citation27–29). Carotid–femoral PWV was measured using the Complior SP PWV evaluation system (Arthec Medical, Pantin, France). As different BP levels alter vascular compliance, PWV mean values were adjusted for mean arterial pressure (MAP).
Statistical analysis
Descriptive statistics are given as means ± standard deviation. The sample size for FMD and PWV were calculated for a power of 0.80. The one-way analysis of variance (ANOVA) and Bonferroni's multiple comparison tests were used to evaluate the clinical characteristics and biochemical variables, as well as to compare changes in brachial artery dilatation and PWV between the study groups. Multiple linear regressions were performed to determine the effect of the different variables on FMD and arterial stiffness (PWV). The association between PWV and FMD was expressed by Pearson's correlation coefficient. All calculations were performed using SPSS for Windows, version 17.0 (SPSS Inc., Chicago, IL, USA) and the GraphPad Prism 5 Statistical package (CA) was used for all analyses. A p-value < 0.05 indicated significance.
Results
Characteristics of the study groups
The general characteristics of the RHTN and well-controlled HTN and NT subjects are summarized in . SBP and DBP were significantly higher in the RHTN group when compared with the other two groups (p < 0.001). No significant differences were observed in respect to body mass index (BMI), age or other biochemical variables [blood sugar, total cholesterol, low-density lipoprotein (LDL)-cholesterol, high-density lipoprotein (HDL)-cholesterol, triglycerides, and plasma sodium, potassium and creatinine levels, as well as 24-h urinary sodium excretion] among the three groups.
Table I. Clinical characteristics of resistant (RHTN), well-controlled (HTN) hypertensive patients and normotensive subjects (NT).
Multiple linear regressions adjusted for BMI, total cholesterol, LDL-cholesterol, HDL-cholesterol, triglycerides and plasma sodium, potassium and creatinine levels showed no significant relationship among these risk factors concerning endothelial function measured by FMD and arterial wall stiffness evaluated by PWV in RHTN individuals.
The FMD and PWV results of the three studied groups are summarized in .
Table II. Results of arterial profile readings in pulse wave velocity (PWV), brachial artery flow-mediated dilation (FMD) and nitroglycerin-induced (NTG) of resistant hypertensive (RHTN), well-controlled (HTN) hypertensive patients and normotensive studied subjects (NT).
The carotid–femoral PWV adjusted for systolic (SAP, mmHg), diastolic (DAP, mmHg) and mean arterial (MAP) pressures among the three groups are resumed in .
Table III. Comparison of pulse wave velocity mean values (PWV C-F, m/s) adjusted for systolic (SAP, mmHg), diastolic (DAP, mmHg) and mean arterial (MAP) pressures among the three groups (RHTN, HTN and NT).
The endothelium-dependent (FMD) and independent (GTN-induced) vasodilation in resistant and well-controlled hypertensive patients and normal subjects are showed in . FMD (NO-dependent) values were statistically different when comparing RHTN and well-controlled HTN patients (respectively, 8.3 ± 4.7% and 10.1 ± 5.9%) and 12.3 ± 6.3% in normal subjects (p < 0.05). No differences were found for GTN-induced (endothelium-independent) responses (27.6 ± 7.7% in RHTN patients, 28.7 ± 10.2% in HTN patients and 29.7 ± 10.5% in NT subjects).
Figure 1. Results of readings of the brachial artery flow-mediated dilation (FMD) and nitroglycerin-induced (NTG) of the resistant hypertensive (RHTN), well-controlled (HTN) hypertensive patients and the normotensive studied subjects (NT).
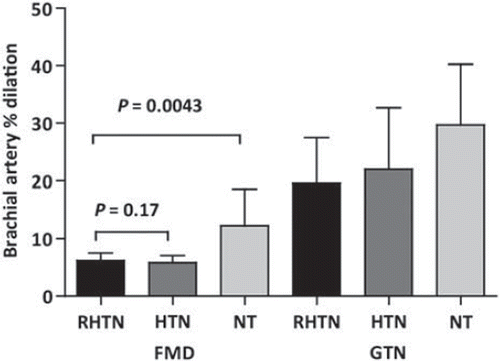
summarizes PWV results in resistant and well-controlled hypertensive patients (13.3 ± 1.2 and 11.5 ± 1.5 m/s, respectively) and in normal subjects (8.5 ± 0.9 m/s). One-way ANOVA showed a significant difference in PWV among RHTN, HTN and NT groups (p < 0.05). As the three groups had different BP levels, PWV mean values were adjusted for SAP, DAP and MAP to be sure that the vessel wall properties differ among the groups ().
Figure 2. Results of readings of the arterial profile in pulse wave velocity (PWV) of the resistant hypertensive (RHTN), well-controlled (HTN) hypertensive patients and the normotensive studied subjects (NT).
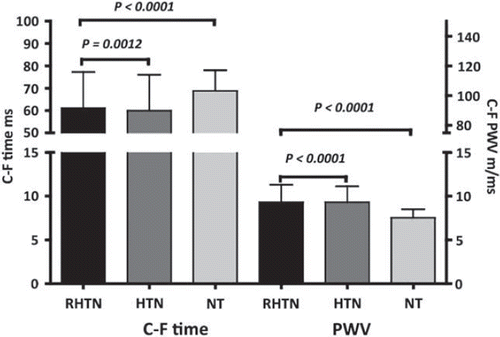
Finally, FMD (NO-dependent) and PWV adjusted values were strongly correlated in well-controlled hypertensive and normotensive subjects (r = −0.74 and −0.83, respectively). Although statistically significant, this correlation was lower in RHTN patients (r =−0.43) ().
Figure 3. (A) Correlation between flow-mediated dilation (FMD; NO-dependent) and pulse wave velocity (PWV)-adjusted values in resistant hypertensive (RHTN); (B) correlation between FMD (NO-dependent) and PWV-adjusted values in well-controlled (HTN) hypertensive patients; (C) correlation between FMD (NO-dependent) and PWV-adjusted values in normotensive studied subjects (NT).
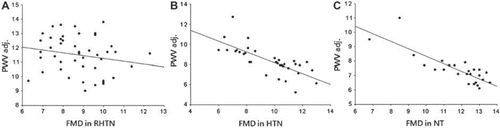
Discussion
The results of the current study show a close relationship among high blood pressure levels, endothelial dysfunction and vascular stiffness in hypertensive patients, demonstrated by a significantly greater increase in carotid–femoral PWV and a decrease in brachial artery FMD in RHTN when compared with well-controlled hypertensive patients. Arterial stiffening occurs as a consequence of structural changes in connective tissue proteins within the tunica media, endothelial and smooth muscle cells of the arterial wall, thus potentially related to risk for the development and progression of atherosclerosis (Citation30), and is unequivocally correlated with cardiovascular events in hypertensive patients and in the general population with other risk factors (Citation28).
The data from this study demonstrating increased carotid–femoral PWV that reflects arterial stiffening as a result of structural changes in the arterial wall, as well as reduced FMD of the brachial artery, which is a marker of NO bioavailability in endothelial cells, suggest that endothelial function may be an important determinant of central hemodynamics and stiffness of large arteries, giving further support to the importance of NO in regulating large artery stiffness in vivo.
Results correlating endothelial dysfunction with various index of arterial stiffness have recently been described in hypertensive patients and among patients with coronary artery disease (Citation13,Citation31). These reports suggest that impaired NO bioavailability was probably involved in arterial stiffness and endothelial dysfunction (Citation32). Because arterial stiffness is a dynamic parameter (Citation33) and without a doubt the greatest indicator of arterial stiffness, it can clearly be modulated by structural and functional changes in the arterial wall, such as blood pressure values (Citation34). Moreover, it is known that endothelial function correlates more strongly with central pressure than peripheral pulse pressure and is independent and inversely correlated with augmentation index, an estimate of systemic arterial (elastic plus muscular) stiffness (Citation13).
However, studies involving the relationship between endothelial function and arterial stiffness in RHTN patients have not previously been reported.
We found that aortic PWV was significantly and inversely correlated with FMD in the brachial artery in the three studied groups. This correlation was higher in well-controlled patients than in RHTN patients. Arterial stiffening related to hypertension is an insidious and progressive process associated with a number of adverse hemodynamic effects and conditions associated with endothelial dysfunction (Citation12,Citation18,Citation31). It also sets up a vicious cycle in which subtle early damage serves to accelerate the rise in systolic pressure, which, in turn, causes further degeneration of aortic function (Citation32–34). This sets the stage for the mid-life rise in systolic pressure, progressing to isolated systolic hypertension and resistant systolic hypertension (Citation35–37). From this point, endothelial dysfunction has limited participation and self-perpetuating pressure-mediated mechanical stress is the key factor for elastin fragmentation, collagen deposition and fibrosis, which cause progressive arterial rigidity (Citation38,Citation39). Thus, a reduced correlation between impaired endothelial function and enhanced vascular stiffness occurs, as we observed in the RHTN group.
Poorly controlled hypertension undoubtedly leads to progressive vascular damage. This effect sets up a vicious cycle, during which increasing vascular stiffness leads to an increase in BP, contributing in turn to further arterial stiffening. This sets the stage for the progressive worsening of hypertension and an increasing need for more and more BP medications (Citation28,Citation36). Accordingly, the current data support the hypothesis that the progression from early to severe stages of hypertension and difficult to control hypertension is characterized by progressive large arterial rigidity (Citation29,Citation36,Citation40). Recognition of this progression is clinically important, as it may allow indexing of vascular stiffness to facilitate early identification of patients at risk of developing RHTN.
In addition to making effective lifestyle changes, early intervention with appropriate pharmacological agents may slow or even prevent the development and progression of RHTN. Recent evidence linking NO to the functional regulation of stiffness in large arteries provides a novel therapeutic target for drugs, aiming to slow down or reverse the effects of premature vascular aging frequently seen in cardiovascular disease (Citation41–43). In spite of using ACEIs and/or ARBs, and CCBs, in similar frequencies, which enhance basal NO release and thereby help to preserve endothelial function (Citation29), the FMD testing was impaired in both hypertensive groups. This could be explained in part by previous findings of our group, which showed that combined therapy and an ACE inhibitor + ARB can induce “aldosterone escape” in hypertensive patients after 12 weeks of treatment, which would also explain the increased vascular stiffness in both RHTN and well-controlled patients (Citation40). In addition, evidence is emerging that mineralocorticoid receptor blockade is useful mainly in treating RHTN patients (Citation4,Citation6,Citation8). As few patients in the RHTN (6%) and well-controlled groups (1.4%) were taking aldosterone antagonists, it is tempting to speculate that this aldosterone escape contributed to endothelial dysfunction and increased arterial stiffness in both hypertensive groups. Recently, other investigators have demonstrated that the initial improvement in endothelial dysfunction caused by ACEIs and ARBs in mild to moderate hypertension is not maintained after 1 year (Citation44).
Notwithstanding the limitations, the results of this study are similar to those obtained in the CAFE study (Citation45) and to other published reports, which considered the PWV method to be reliable as an auxiliary tool to decide on the most appropriated antihypertensive therapy required to improve arterial elasticity in RHTN patients. These results were independent of age, baseline BP, previous antihypertensive drug therapy as well as traditional cardiovascular risk factors.
Clinical implications
In view of the fact that FMD and PWV are correlated to each other, PWV provides supplementary information related to the data obtained by brachial BP measurement. The combination of these measurements will be of stronger clinical relevance to improve cardiovascular risk stratification (Citation22,Citation46).
Finally, although this study was not designed to test this hypothesis, the vascular damage differences observed between patients with controlled vs uncontrolled hypertension suggest that the latter group may have a worse cardiovascular prognosis, requiring prospective assessment tests.
Conclusion
We have demonstrated that RHTN patients have worse endothelial dysfunction and vascular stiffness than well-controlled hypertensive patients. Thus, increases in PWV reflect upon arterial stiffness and are closely correlated with reduced values of brachial artery FMD, a surrogate clinical marker of endothelial dysfunction, and both alterations have straight correlation with BP levels. It seems to be reasonable that strict BP control should be obtained to prevent severe functional and structural vascular changes during the time course of the hypertensive disease. We also propose that non-invasive modalities used to evaluate vascular stiffness (PWV) and endothelial function of the brachial artery (FMD) should be used in the clinical practice to stratify cardiovascular risk, even in well-controlled patients, in order to improve arterial stiffness, increase NO in the arterial wall and target abnormal arterial structures.
Acknowledgments
This study was supported by FAPESP, SP, Brazil. H. Moreno Jr. and V. Nasser Figueiredo were supported by Conselho Nacional de Pesquisa (CNPq) and CAPES (Brazil), respectively.
Competing interests
Leandro Boer-Martins is employee of Novartis Biociências S.A. (Brazil).
References
- Calhoun DA, Jones D, Textor S, Goff DC, Murphy TP, Toto RD, . Resistant hypertension: Diagnosis, evaluation, and treatment. A scientific statement from the American Heart Association Professional Education Committee of the Council for High Blood Pressure Research. Circulation. 2008;117:e510–e526.
- Hall WD. Resistant hypertension, secondary hypertension, and hypertensive crises. Cardiol Clin. 2002;20:281–219.
- Pierdomenico SD, Lapenna D, Bucci A, Di Tommaso R, Di Mascio R, Manente BM, . Cardiovascular outcome in treated hypertensive patients with responder, masked, false resistant, and true resistant hypertension. Am J Hypertens. 2005;18:1422–1428.
- Papadopoulos DP, Papademetriou V. Resistant hypertension: Diagnosis and management. J Cardiovasc Pharmacol Ther. 2006;11:113–118.
- Sowers JR, Whaley-Connell A, Epstein M. Narrative review: The emerging clinical implications of the role of aldosterone in the metabolic syndrome and resistant hypertension. Ann Intern Med. 2009;150:776–783.
- Ubaid-Girioli S, Adriana de Souza L, Yugar-Toledo JC, Martins LC, Ferreira-Melo S, Coelho OR, . Aldosterone excess or escape: Treating resistant hypertension. J Clin Hypertens (Greenwich). 2009;11:245–252.
- Pimenta E, Calhoun DA, Oparil S. Sleep apnea, aldosterone, and resistant hypertension. Prog Cardiovasc Dis. 2009;51:371–380.
- Landmesser U, Drexler H. Endothelial function and hypertension. Curr Opin Cardiol. 2007;22:316–320.
- Perticone F, Ceravolo R, Pujia A, Ventura G, Iacopino S, Scozzafava A, . Prognostic significance of endothelial dysfunction in hypertensive patients. Circulation. 2001;104:191–196.
- Yugar-Toledo JC, Tanus-Santos JE, Sabha M, Sousa MG, Cittadino M, Tácito LH, . Uncontrolled hypertension, uncompensated type II diabetes, and smoking have different patterns of vascular dysfunction. Chest. 2004;125:823–830.
- Wilkinson IB, Franklin SS, Cockcroft JR. Nitric oxide and the regulation of large artery stiffness: From physiology to pharmacology. Hypertension. 2004;44:112–116.
- Zieman SJ, Melenovsky V, Kass DA. Mechanisms, pathophysiology, and therapy of arterial stiffness. Arterioscler Thromb Vasc Biol. 2005;25:932–943.
- McEniery CM, Wallace S, Mackenzie IS, McDonnell B, Yasmin, Newby DE, . Endothelial function is associated with pulse pressure, pulse wave velocity, and augmentation index in healthy humans. Hypertension. 2006;48:602–608.
- Safar M, Chamiot-Clerc P, Dagher G, Renaud JF. Pulse pressure, endothelium function, and arterial stiffness in spontaneously hypertensive rats. Hypertension. 2001;38: 1416–1421.
- Schmitt M, Avolio A, Qasem A, McEniery CM, Butlin M, Wilkinson IB, . Basal NO locally modulates human iliac artery function in vivo. Hypertension. 2005;46:227–231.
- O’Rourke MF, Pauca A, Jiang XJ. Pulse wave analysis. Br J Clin Pharmacol. 2001;51:507–522.
- O’Rourke MF. Wave travel and reflection in the arterial system. J Hypertens Suppl. 1999;17:S45–S47.
- Laurent S, Cockcroft J, Van Bortel L, Boutouyrie P, Giannattasio C, Hayoz D, . Expert consensus document on arterial stiffness: Methodological issues and clinical applications. Eur Heart J. 2006;27:2588–2605.
- O’Rourke MF, Staessen JA, Vlachopoulos C, Duprez D, Plante GE. Clinical applications of arterial stiffness; definitions and reference values. Am J Hypertens. 2002;15:426–44.
- Roman MJ, Devereux RB, Kizer JR, Lee ET, Galloway JM, Ali T, . Central pressure more strongly relates to vascular disease and outcome than does brachial pressure: The Strong Heart Study. Hypertension. 2007;50:197–203.
- Topouchian J, El Feghali R, Pannier B, Wang S, Zhao F, Smetana K, . Arterial stiffness and pharmacological interventions – The TRanscend arterial stiffNess Substudy (TRANS study). Vasc Health Risk Manag. 2007;3:381–387.
- Protogerou A, Blacher J, Stergiou GS, Achimastos A, Safar ME. Blood pressure response under chronic antihypertensive drug therapy: The role of aortic stiffness in the REASON (Preterax in Regression of Arterial Stiffness in a Controlled Double-Blind) study. J Am Coll Cardiol. 2009;53:445–451.
- Celermajer DS, Sorensen KE, Gooch VM, Spiegelhalter DJ, Miller OI, Sullivan ID, . Non-invasive detection of endothelial dysfunction in children and adults at risk of atherosclerosis. Lancet. 1992;340:1111–1115.
- Sorensen KE, Celermajer DS, Spiegelhalter DJ, Georgakopoulos D, Robinson J, Thomas O, . Non-invasive measurement of human endothelium dependent arterial responses: Accuracy and reproducibility. Br Heart J. 1995;74:247–253.
- Chobanian AV, Bakris GL, Black HR, Cushman WC, Green LA, Izzo JL Jr, . Seventh report of the Joint National Committee on Prevention, Detection, Evaluation, and Treatment of High Blood Pressure. Hypertension. 2003;42:1206–1252.
- Corretti MC, Anderson TJ, Benjamin EJ, Celermajer D, Charbonneau F, Creager MA, Deanfield J, . Guidelines for the ultrasound assessment of endothelial-dependent flow-mediated vasodilation of the brachial artery: A report of the International Brachial Artery Reactivity Task Force. J Am Coll Cardiol. 2002;39:257–265.
- Asmar R, Benetos A, Topouchian J, Laurent P, Pannier B, Brisac AM, . Assessment of arterial distensibility by automatic pulse wave velocity measurement. Validation and clinical application studies. Hypertension. 1995;26:485–490.
- Blacher J, Asmar R, Djane S, London GM, Safar ME. Aortic pulse wave velocity as a marker of cardiovascular risk in hypertensive patients. Hypertension. 1999;33:1111–1117.
- Safar ME, Blacher J, Protogerou A, Achimastos A. Arterial stiffness and central hemodynamics in treated hypertensive subjects according to brachial blood pressure classification. J Hypertens. 2008;26:130–137.
- Duprez DA, Cohn JN. Arterial stiffness as a risk factor for coronary atherosclerosis. Curr Atheroscler Rep. 2007;9:139–144.
- Laurent S, Boutouyrie P. Recent advances in arterial stiffness and wave reflection in human hypertension. Hypertension. 2007;49:1202–1206.
- Ahlund C, Pettersson K, Lind L. Pulse wave analysis on fingertip arterial pressure: Effects of age, gender and stressors on reflected waves and their relation to brachial and femoral artery blood flow. Clin Physiol Funct Imaging. 2008;28:86–95.
- London GM, Marchais SJ, Guerin AP, Pannier B. Arterial stiffness: Pathophysiology and clinical impact. Clin Exp Hypertens. 2004;26:689–699.
- O’Rourke MF. Clinical assessment of arterial stiffness. Am J Hypertens. 2007;20:839.
- Williams B. The aorta and resistant hypertension. J Am Coll Cardiol. 2009;53:452–454.
- Martins LC, Figueiredo VN, Quinaglia T, Boer-Martins L, Yugar-Toledo JC, Martin JF, . Characteristics of resistant hypertension: Ageing, body mass index, hyperaldosteronism, cardiac hypertrophy and vascular stiffness. J Hum Hypertens. 2011;25:532–538.
- Mackenzie IS, McEniery CM, Dhakam Z, Brown MJ, Cockcroft JR, Wilkinson IB. Comparison of the effects of antihypertensive agents on central blood pressure and arterial stiffness in isolated systolic hypertension. Hypertension. 2009;54:409–413.
- Thorin E, Thorin-Trescases N. Vascular endothelial ageing, heartbeat after heartbeat. Cardiovasc Res. 2009;84:24–32.
- Wilkinson IB, McEniery CM. Arterial stiffness, endothelial function and novel pharmacological approaches. Clin Exp Pharmacol Physiol. 2004;31:795–799.
- Safar ME, Levy BI, Struijker-Boudier H. Current perspectives on arterial stiffness and pulse pressure in hypertension and cardiovascular diseases. Circulation. 2003;107:2864–2869.
- Protogerou AD, Papaioannou TG, Lekakis JP, Blacher J, Safar ME. The effect of antihypertensive drugs on central blood pressure beyond peripheral blood pressure. Part I: (Patho)-physiology, rationale and perspective on pulse pressure amplification. Curr Pharm Des. 2009;15:267–271.
- Protogerou AD, Stergiou GS, Vlachopoulos C, Blacher J, Achimastos A. The effect of antihypertensive drugs on central blood pressure beyond peripheral blood pressure. Part II: Evidence for specific class-effects of antihypertensive drugs on pressure amplification. Curr Pharm Des. 2009;15: 272–289.
- Yasmin, O’Shaughnessy KM. Genetics of arterial structure and function: Towards new biomarkers for aortic stiffness? Clin Sci (Lond). 2008;114:661–677.
- Jatoi NA, Jerrard-Dunne P, Feely J, Mahmud A. Impact of smoking and smoking cessation on arterial stiffness and aortic wave reflection in hypertension. Hypertension. 2007;49:981–985.
- Williams B, Lacy PS, Thom SM, Cruickshank K, Stanton A, Collier D, . Differential impact of blood pressure-lowering drugs on central aortic pressure and clinical outcomes: Principal results of the Conduit Artery Function Evaluation (CAFE) study. Circulation. 2006;113:1213–1225.
- Boos CJ, Lane DA, Karpha M, Beevers DG, Haynes R, Lip GY. Circulating endothelial cells, arterial stiffness, and cardiovascular risk stratification in hypertension. Chest. 2007;132:1540–1547.