Abstract
Renin is the rate-limiting step of the renin–angiotensin system (RAS) and can induce hypertension and cardiovascular diseases (CVDs) through the over-activated renin–angiotensin-converting enzyme (ACE)–angiotensin (Ang) II–Ang II type 1 receptor (AT1R) axis. Prorenin and renin bound to the (pro)renin receptor [(P)RR] not only increase the catalytic conversion of angiotensinogen (AGT) to Ang I, but also upregulate the expression of profibrotic genes. This review will discuss the inhibition of renin and the (P)RR system pharmacologically and nutritionally.
Introduction
In mammals, the renin–angiotensin system (RAS) plays a key role in maintaining blood pressure homeostasis and fluid and salt balance, and involves in tissue pathphysiological processes (Citation1). The classical axis angiotensin-converting enzyme (ACE)–angiotensin (Ang) II–Ang II type 1 receptor (AT1R) had been regarded as the only system of the RAS for a long time. Its hyperactivity is associated with hypertension and cardiovascular disease (CVDs), such as cardiac hypertrophy, heart failure, stroke, coronary artery disease and end-stage renal disease (Citation2). To control this axis, over 20 clinical drugs have been developed but they are not totally without side-effects. Being non-specific for the RAS and preventing inactivation of bradykinin and substance P (Citation3), ACE inhibitors can result in dry cough that may lead to the discontinuation of treatment and angioedema that can be life-threatening (Citation4). Exposure to ACE inhibitors during pregnancy may place the infant at an increased risk of fetopathy and major congenital malformations (Citation5). Sipahi et al. suggested in 2010 that AT1R blockers (ARBs) are associated with a modestly increased risk of new cancer diagnosis, although conclusions about the exact risk of cancer associated with each particular drug have not been drawn (Citation6). Based on this study, the US FDA is conducting a review to assess the possible link between the use of ARBs and cancer. In addition, both ACE inhibitors and ARBs lead to a substantial compensatory rise in circulating active renin and Ang peptides that may eventually limit their therapeutic potential (Citation3). The increased Ang I can subsequently be converted to Ang II by non-ACE pathways, mediated by chymase and chymotrypsin-like Ang-generating enzyme (Citation7). In humans, these alternate pathways may be responsible for up to one third of Ang II (Citation8). Antihypertensive therapy by vasopeptidase inhibition may also parallelly upregulate renin and the (pro)renin receptor [(P)RR] in vivo (Citation9). Over the last decade, a number of food-derived compounds have been shown to have in vitro ACE inhibitory activity. Among them, food-derived ACE inhibitory peptides have been the most widely studied (Citation10,Citation11). Intestinal digestion and absorption generally affect the bioavailability of ACE inhibitory peptides (Citation11). Also, in vitro ACE inhibitory peptides may exert antihypertension not through an Ang-mediated effect of ACE inhibition in humans (Citation12). Alternative mechanisms include opioid-like activities, increased availability of endogenous bradykinin, inhibition of the release of the vasoactive substances such as the vasoconstrictor endothelin-1, eicosanoids and nitric oxide (Citation13). So further investigation into in vivo and clinical antihypertensive effects and mechanisms of ACE inhibitory peptides is necessary. Products containing lactotripeptides VPP and/or IPP have been commercialized (such as AmealPeptide® and tensVida ). However, studies in Dutch subjects by Engberink et al. (Citation14), van der Zander et al. (Citation15) and van Mierlo et al. (Citation16) showed neither systolic nor diastolic blood pressure lowering effects of lactotripeptide-containing products.
The discovery of the ACE analogue ACE2 and its pertinent axis ACE2–Ang–(1-7)-Mas has established a new concept for the RAS. The ACE2–Ang–(1-7)-Mas axis counterregulates the vasoconstrictive, proliferative, fibrotic and proinflammatory effects of the ACE–Ang II–AT1R axis (Citation17), and thus could serve as a new direction for improved therapeutics for hypertension and CVDs. Mas activators AVE 0991, hydroxyprophl-β-cyclodextrin/Ang-(1-7), CGEN856 and CGEN857 are under investigation (Citation2). The ACE2 activator XNT (CAS# 86456-22-6) found in 2008 (Citation18), however, has limitations for preclinical levels because XNT is a hydrophobic compound and very hard for any toxicological and dose response experiments, and its synthesis is tremendously complex and expensive. The newer ACE2 activator diminazine aceturate (DIZE) (Citation19) is a veterinary antibabesial agent with serious side-effects (Citation20), and therefore a challenge to be developed into an effective therapy for human hypertension and CVDs. ACE2 is a ubiquitous enzyme, and in addition to the Ang II system it may catalyze other peptide systems, such as bradykinin and apelin. ACE2 is also an essential receptor for severe acute respiratory syndrome (SARS) (Citation21) virus and the human coronavirus NL63 (Citation22). So the effects of ACE2 activators on the immune competence of animals and their vulnerability to SARS infection must be tested before these molecules are ready for preclinical trials (Citation2). Lactotripeptides VPP and IPP inhibit in vitro ACE2 activity at millimolar concentrations (Citation23).
Direct renin inhibition (DRI) may be a more optimal approach to the RAS inhibition. Renin, prorenin and their receptor form the new (P)RR system that shows an Ang-dependent function related to increased generation of Ang I and an Ang-independent aspect related to the intracellular signaling. This review will discuss the inhibition of renin and the (P)RR system pharmacologically and nutritionally and thus provide a perspective for food-derived bioactives related to the two targets.
Direct renin inhibition
Renin is the first and rate-limiting step of the RAS. The active renin found in the circulation of mammals is predominantly produced by the kidney juxtaglomerular (JG) cells. Under physiological conditions, several enzymes, including proconvertase 1 and cathepsin B, irreversibly cleave the prosegment of prorenin, which allows the conversion of prorenin into mature renin before renin is released from the JG cells upon demands (Citation24). Like most other aspartic proteases, Renin is composed of two mainly β-sheet domains with one catalytic Asp carboxylate from each domain. The enzyme pocket for substrate binding is located between the two domains of the molecule. Renin is a highly specific enzyme and has only one known substrate AGT. It cleaves the peptide at the Leu10–Val11 bond and leads to the formation of Ang I, which is next converted by ACE into Ang II, the major effector and mediator of RAS physiological actions (Citation25).
DRI could lead to more complete blockade of the RAS. It significantly reduces plasma renin activity (PRA) and prevents the downstream formation of Ang I, Ang II and aldosterone, causes vasodilation, increases sodium and water excretion and therefore lowers the blood pressure. The reduction of PRA despite the increased plasma renin concentrations (PRC) (from loss of the Ang II negative feedback of suppressing the renin release) is regarded to be beneficial since increased PRA is independently and directly associated with increased incident myocardial infarction and cardiovascular events (Citation26). DRI does not affect kinin metabolism and hence would not be expected to cause dry cough and angioedema as mediated by ACE inhibitors. ARBs increase levels of Ang II and indirectly stimulate Ang II type 2 receptor (AT2R), which may contribute to cardiac fibrosis (Citation3). DRI avoids such unexpected effects of AT2R activation.
Renin inhibitors
The concept of blocking the RAS at its origin by inhibiting renin has existed for at least 50 years. The first-generation renin inhibitors were peptide analogues of AGT and the second-generation compounds were peptidomimetic agents that are dipeptide transition-state analogue inhibitors of the active site (Citation7). In these peptidic inhibitor–enzyme complexes, the inhibitors bind in an extended conformation occupying the active sites of six pockets S4, S3, S2, S1, S1′ and S2′(Citation27). These renin inhibitors, however, had limited clinical use due to poor metabolic stability and oral bioavailability, short duration of action, weak antihypertensive activity and high cost of synthesis (Citation7).
Molecular modeling and X-ray crystallographic analysis of active sites of renin led to the development of aliskiren, a new non-peptide, low molecular weight, orally active renin inhibitor. Aliskiren acts as a transition state mimetic, inhibiting renin via hydrogen bonding of the central hydroxyl group and amino function to the catalytic Asp32 and Asp215 residues (Citation28). Additionally, the central amino group is also hydrogen bonded to the C = O of Gly217; the primary CONH forms hydrogen bonds with NH of Ser76 and C = O of Gly34; the terminal NH2 interacts with C = O of Arg74 (Citation29). These hydrogen bonds further contribute to the strengthening of aliskiren–renin interactions (Citation30). In particular, the interaction of methoxy group of the alkoxy side-chain with NH of Tyr14 in the non-substance S3sp subpocket provides additional potency and specificity against other aspartic proteases and is essential for high binding affinity (Citation29). Aliskiren occupies five active site pockets S3sp, S3, S1, S1′ and S2′, and without large P4–P2 spacing backbone it does not interact with the S4 or S2 binding sites of renin (Citation27). Compared with the earlier renin inhibitors, aliskiren is more resistant to intestinal degradation and possesses significantly improved oral bioavailability. The terminal half-life ranges from 23 to 40 h, which makes it suitable for once-daily dosing. Aliskiren monotherapy displays an antihypertensive efficacy similar if not superior to that of other first-line antihypertensive drugs, with a safety and tolerability profile similar to that of ARBs. Combined with various antihypertensive agents aliskiren exhibits synergistic effects (Citation7,Citation31). From 2007, aliskiren was approved by regulatory bodies in both Europe and the USA, for use alone and with other agents in the treatment of hypertension (Citation3). The greater reactive increase in PRC seen with aliskiren, however, might be a problem in the long-term control of blood pressure especially for those hypertensive patients who have hyperreactive renin systems (Citation32).
Studies have yielded mixed results on the effect of aliskiren on end organ protection (). In the Aliskiren in the Evaluation of Proteinuria in Diabetes (AVOID) trial, the addition of aliskiren to full-dose losartan significantly reduced the urine albumin to creatinine ratio in diabetic patients with concomitant hypertension and nephropathy (Citation33). In the Aliskiren Observation of Heart Failure Treatment (ALOFT) study, the add-on treatment with aliskiren was associated with significant reductions in brain natriuretic peptide, N-terminal pro-brain natriuretic peptide (NT-proBNP) and urinary aldosterone levels in patients with New York Heart Association class II–IV heart failure (Citation34). In the Aliskiren Left Ventricular Hypertrophy (ALLAY) trial&UnknownEntity1;a significant reduction in left ventricular (LV) mass index was achieved either by the combination of aliskiren and losartan or by aliskiren alone, and both were as effective as losartan in reducing LV mass index (Citation35). However, in the Aliskiren and Valsartan to Reduce NT-proB-type natriuretic peptide via Renin–Angiotensin– Blockade (AVANT GARDE)-TIMI 43 Trial, early inhibition of the RAS with valsartan, aliskiren, or their combination did not resulted in a benefit compared with placebo with respect to a reduction in NT-proBNP and was associated with more adverse events (Citation36). In the Aliskiren Study in Post Myocardial Infarction Patients to Reduce Remodeling (ASPIRE), adding aliskiren to a standard optimal medical regimen did not result in further attenuation of LV remodeling and was associated with more adverse events in high risk post-myocardial infarction patients with LV systolic dysfunction (Citation37). In December 2011, the pharmaceutical company Novartis announced the early termination of the Aliskiren Trial in Type 2 Diabetes Using Cardio-Renal Endpoints (ALTITUDE) and advised that dual aliskiren and ACE inhibitors or ARBs should not be used in patients with both hypertension and diabetes or moderate to severe renal dysfunction, following the conclusion from the overseeing Data Monitoring Committee that study patients were unlikely to benefit from aliskiren and there was a higher incidence of adverse events related to non-fatal stroke, renal complications, hyperkalemia and hypotension after 18–24 months of combined therapy in type 2 diabetic patients at high risk of fatal and non-fatal cardiovascular and renal events (Citation38). Although this unexpected finding of the ALTITUDE is provoking concern and discussion, Aliskiren has been shown to be a very safe antihypertensive following over 20 000 patient-years of data. As we have seen in the ONgoing Telmisartan Alone and in combination with Ramipril Global Endpoint Trial (ONTARGET) that the combination of telmisartan (an ARB) and ramipril (an ACE inhibitor) is associated with more adverse events without an increase in benefit in patients who have vascular disease or high-risk diabetes without heart failure (Citation39), the combination of multiple safe drugs in this class may not be a promising strategy any longer.
Table I. Trials of aliskiren.
The evolution of non-peptide renin inhibitors interacting with non-substrate recognition sites of the enzyme, such as aliskiren and the 4-aryl-piperidines, has encouraged an increasing number of pharmaceutical studies to develop new generations of renin inhibitors (Citation40). The lead compound from Speedel Holding Ltd., SPP635, has completed the phase IIa clinical trial, and showed good safety and strong efficacy of lowering blood pressure with a significant decrease of the PRA in patients with mild-to-moderate hypertension. In the pre-clinical studies, SPP635 showed long half-time, high oral bioavailability and good efficacy in lowering blood pressure and preventing renal damage in animal models (Citation41). More data about SPP635 should be available soon.
Inhibition/blockade of the (P)RR system
The traditional assumptions of prorenin being just an “inactive” proenzyme and of renin being just an enzyme responsible for the cleavage of AGT were challenged by the cloning of a human (P)RR in 2002 (renin was found in 1898) (Citation25), and thereafter a novel research field of the (P)RR system was founded. The (P)RR is a single transmembrane-domain protein of 350 amino acids with a large unglycosylated and highly hydrophobic N-terminal domain responsible for renin and prorenin binding and a short cytoplasmic tail of about 20 amino acids involved in the intracellular signaling (Citation42). The “hinge” region (S149QGVLKEDVF158 ) of the mature renin, located near the bottom part of the active site of renin and present commonly both in renin and prorenin molecules, has a crucial role in the binding of renin and prorenin to the (P)RR (Citation43). Two regions in the human prorenin prosegment, namely T7PFKR10P (a gate that is not accessible by its specific antibodies until it is loosened from the active site cleft) and I11PFLKR15P (a handle, a protruding pentameric segment), are also crucial for the non-proteolytic activation of prorenin (Citation44). So renin has a single and prorenin has multiple high affinity binding domains for the (P)RR with the KD of mature renin being almost four times higher than that of prorenin (Citation43). The nanomolar affinity for renin and prorenin is several orders of magnitude above their actual (picomolar) levels in blood and thus the (P)RR interaction is limited to (pro)renin synthesizing organs (Citation45). Binding of prorenin induces a conformational change in the molecule, increasing its enzymatic activity from virtually zero to values similar to those of active renin in solution without proteolytic removal of the prosegment (Citation24). Renin bound to the (P)RR displays a three- to five-fold increase in the catalytic efficiency of AGT conversion to Ang I compared with free renin (Citation42). In addition to the Ang-dependent function on the cell surface related to the increased catalytic activity of receptor-bound (pro)renin (renin activity of activated prorenin and increased activity of renin), the (P)RR system also has Ang- independent intracellular effects that are not necessarily related to the RAS (Citation46). In the Ang-independent signaling pathways, prorenin-induced activation of mitogen-activated protein kinase (MAPK) p38 and subsequent phosphorylation of heat shock protein (HSP) 27 result in actin polymerization, while (pro)renin-induced activation of the extracellular signal-regulated kinase (ERK) 1/2 (p44/p42) signaling cascade leads to the intracellular expression of profibrotic genes, giving rise to the synthesis of transforming growth factor (TGF-β), plasminogen-activator inhibitor-1 (PAI-1), collagen 1 and fibronectin (). These effects potentially increase cardiac and renal hypertrophy and fibrosis (Citation42,Citation47–49). Renin binding to the (P)RR also activates promyelocytic zinc finger protein (PLZF)-phosphatidylinositol-3kinase (PI3K)-p85α pathway, which can lead to an increase in proliferation and a decrease in apoptotic activity, and PLZF translocation into the nucleus upon renin stimulation represses transcription of the (P)RR itself as a negative feedback loop () (Citation50).
Figure 1. Pathways mediated by the (pro)renin receptor. (See text for explanations and abbreviations).
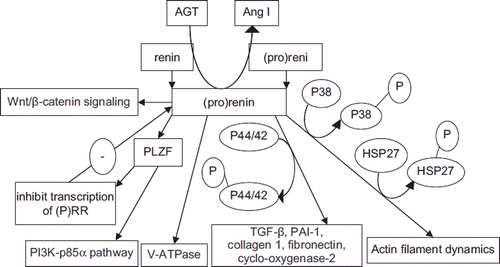
Renin inhibitor aliskiren has no specific binding to the (P)RR and thus is not a receptor antagonist (Citation51). It binds to the active site of (pro)renin and competitively inhibits renin activities of (P)RR-bound renin and prorenin (Citation51,Citation52). There are controversies over effects of aliskiren on the binding of renin to the (P)RR and the receptor expression. A study conducted with U397 cells, showed that aliskiren did not interfere with renin or prorenin binding to the (P)RR and did not inhibit renin and prorenin-induced ERK1/2 phosphorylation and kinase activity (Citation53). Also, aliskiren did not prevent renin and prorenin-induced ERK1/2 phosphorylation in vascular smooth muscle cells (Citation54). MAPK kinase 1/2 inhibitor PD98059 did inhibit both (Citation53,Citation54). In human mesangial cells, aliskiren did not inhibit binding of renin to the (P)RR, nor did it alter the activation of ERK1/2 by renin pre-incubated with aliskiren or affect gene expression of the (P)RR (Citation52). In HEK293 cells, aliskiren did not affect the (P)RR expression after stimulation with either renin or prorenin and did not alter PI3K activation, ATP concentration, or BrdU incorporation induced in response to (P)RR activation (Citation55). However, binding of aliskiren to the active site of renin led to 1000 times lower affinity of the renin-aliskiren complex to (R)PP than that of free renin directly to the receptor, probably due to a modification of the local conformation along with the hinge region of renin after the combination of aliskiren and renin (Citation51). In human aortic smooth muscle cells with endogenous secretion of (pro)renin, aliskiren significantly reduced expressions of (R)PP, TGF-β, PAI-1 and collagen 1 after cell stimulation simultaneously with both prorenin and AGT. In this study, no AT1 or AT2 receptor blockers were used (Citation56). In streptozotocin-diabetic TG(mRen-2)27 rats, aliskiren treatment significantly reduced renal gene expressions of (R)PP, TGF-β and collagen 1 (Citation52).
Based on the idea that the prosegment contains a handle region that binds to the (R)PP and allows prorenin to become active in a non-proteolytic manner (Citation57), an antagonist, (also known as “hand region peptide”, HRP), consisting of 10 animo acids (NH2-RILLKKMPSV-COOH) and resembling the handle region (I11PLLKK15P) of rat prorenin prosegment, was designed to bind to the (P)RP as a decoy peptide and inhibit the receptor-mediated actions of prorenin (Citation58). The HRP inhibited mesangial cell proliferation, ERK1/2 phosphorylation and TGF-β1 expression, and increased the matrix metalloproteinase-2 activity (Citation59). Treatment with the HRP in diabetic mice and rats decreased the renal content of Ang I and II, and inhibited the development of nephropathy without affecting hyperglycemia (Citation60,Citation61). In stroke-prone spontaneously hypertensive rats (SHRs), continuous subcutaneous administration of the HRP inhibited activation of tissue RAS without affecting circulating RAS or arterial pressure and significantly attenuated the development and progression of proteinuria, glomerulosclerosis and cardiac fibrosis (Citation62,Citation63). Infusion of the HRP in human (P)RR-transgenic rats significantly inhibited the development of glomerulosclerosis, proteinuria, MAPK activation, and TGF-β expression in the kidneys (Citation64). The HRP exerted significant cardiovascular and renal benefit in SHRs with cardiovascular damage produced by salt excess (Citation65). In the non-clipped kidney of two kidney, one-clip hypertensive rats at 12 weeks after clipping, activated prorenin levels, angiotensin II levels and TGF-β mRNA levels of HRP-treated rats were significantly lower than those of vehicle-treated rats (Citation66). Furthermore, beneficial effects of the HRP in vivo were shown in a model of metabolic syndrome (Citation67) as well as regarding diabetic retinopathy (Citation68,Citation69). Future research should be able to determine to what degree the beneficial in vivo effects of HRP are due to prorenin blockade, and are related to the interference with renin binding and the RAS (Citation57). Decoy effects of the HRP, however, were not confirmed by other research groups. Although the HRP bound to U937 cells, it did not interfere with the binding of renin or prorenin to (P)RR nor did it inhibit renin or prorenin-induced ERK1/2 activation (Citation53). In rat vascular smooth muscle cells overexpressing the human (P)RR, the HRP blocked neither prorenin binding nor angiotensin generation (Citation70). The HRP did not prevent renin and prorenin-induced ERK1/2 phosphorylation in vascular smooth muscle cells, and in rats overexpressing renin and AGT it failed to ameliorate target-organ damage in contrast to aliskiren (Citation54). Blockade of the (P)RR with HRP does not improve cardiac hypertrophy and renal damage in renovascular hypertensive rats (Citation71). These contradictory results might probably be explained by different models, experimental methods, or degradation/insufficient bioavailability of the decoys (Citation72). More confusing was the fact that HRP could bind to cells lacking (P)RR on their surface, which indicates an alternative mechanism for its actions (Citation73). Some authors suggested that HRP might even be a partial agonist on the (P)RR (Citation74).
The “hinge” region peptide (S149QGVLKEDVF158), inhibited the binding of renin and prorenin to (P)RR (Citation43). The development of a non-peptide antagonist could shed more light on the potential of (P)RR therapeutic inhibition (Citation72,Citation75). Moreover, the (P)RR is a multifunctional receptor (Citation76). (P)RR activation by (pro)renin can increase the activity of vacuolar H + -ATPase (V-ATPase) (Citation77), a vital enzyme in many physiological and biochemical processes by control of the cellular and intracellular vesicle pH (Citation76). In addition, Wnt/β-catenin signaling is important in stem cell biology, embryonic development and diseases, including cancer. As a component of the Wnt receptor complex, (P)RR interacts with V-ATPase and thus acts as a specific adaptor between the Wnt receptor and the enzyme (). The proton gradient produced by V-ATPase induces Wnt receptor phosphorylation and hence β-catenin activation (Citation78). Therefore, (P)RR blockers, including the HRP, may also have effects on Wnt/β-catenin signaling and V-ATPase and PLZF associated functions (Citation76).
Food-derived non-peptide renin inhibitors
Success of aliskiren has encouraged the search of food-derived non-peptide renin inhibitors. Methanol extracts of rice, buckwheat, rye and barnyard millet showed in vitro human renin inhibitory activity and further analysis indicated that effective compounds of the extracts are oleic acid (18:1) and linoleic acid (18:2) (Citation79). Both fatty acids competitively inhibit human renin activity and other unsaturated free fatty acids, including cis-vaccenic acid (18:1), linolenic acid (18:3), arachidonic acid (20:4), 5,8,11,14,17-eicosapentanoic acid (EPA, 20:5) and 4,7,10,13,16,19-docosahexanoic acid (DHA, 22:6), also possess in vitro inhibitory potency (Citation80). Among them, EPA and DHA have been reported to reduce the systolic blood pressure in a model of human renin hypertension rats (Citation81). Nine minor legume extracts showed in vitro human renin inhibitory activity, and among them those from Vigna angularis, Vigna anguiculata subsp. sesquipendalis and Phascolus vulgaris had higher inhibitory potency (Citation82). Soybean embryo extract showed about three-fold higher human renin inhibitory activity than cotyledon extract and the active compound was proved to be soyasaponin I. Kinetic analysis indicated partial noncompetitive inhibition with a Ki value (Citation83) comparable with that for the synthesized tetradecanoyl acetal sodium sulfate, an analogue of sodium houttuynin (Citation84). Soyasaponin I had very little effect on porcine pepsin or cysteine proteinases (papain and bromeline), and no effect on serine proteinases (bovine trypsin and human kallikrein) or metallo proteinases (rabbit ACE and porcine aminopeptidase), which indicates that soyasaponin I is a renin-specific inhibitor (Citation83). Following simple structure and activity analysis of fourteen saponins and sapogenols, the 3-O-β-d-glucopyranosiduronic moiety in saponins was found to be essential for renin inhibition. In addition to soyasaponin I, soyasaponin II also contains such a structure and has a similar human renin inhibitory activity (Citation85). Oral administration of commercially purified soybean saponin in SHRs significantly decreased blood pressure and led to reductions in plasma levels of low-density lipoprotein cholesterol, aspartate aminotransferase, alanine aminotransferase and blood urea nitrogen, which indicates that soybean saponin may possibly exert its liver and kidney protective functions by lowering the blood pressure level (Citation86).
Compared with RAS regulatory drugs, food-derived bioactives appear more natural and safer to the consumer and may result in a much lower healthcare cost. Food-derived RAS regulators could be applied in the prevention of hypertension and as initial treatment in mildly hypertensive individuals (Citation11). Research would be able to further determine antihypertensive mechanisms and activities of renin inhibitory saponins in vivo/clinically and their effects on the (P)RR system biochemically and physiologically.
Acknowledgments
This work was supported by the Research Program of State Key Laboratory of Food Science & Technology (Jiangnan University, No. SKLF-ZZB-201208), Fundamental Research Funds for the Central Universities (No. JUSRP211A06) and the Self- Determined Research Program of Jiangnan University (No. 5815210232110800).
Conflict of interest: None.
References
- Nguyen G, Contrepas A. Physiology and pharmacology of the (pro)renin receptor. Curr Opin Pharmacol.2008;8:127–132.
- Ferreira AJ, Santos RAS, Bradford CN, Mecca AP, Sumners C, Katovich MJ, . Therapeutic implications of the vasoprotective axis of the ras in cardiovascular diseases. Hypertension. 2010;55:207–213.
- Tabassum N. Aliskiren: A new renin inhibitor as anti-hypertensive. J Appl Pharm Sci. 2011;1:30–33.
- Acharya KR, Sturrock ED, Riordan JF, Ehlers MR. ACE revisited: A new target for structure-based drug design. Nat Rev Drug Discov. 2003;2:891–902.
- Cooper WO, Hernandez-Diaz S, Arbogast PG, Dudley JA, Dyer S, Gideon PS, . Major congenital malformations after first-trimester exposure to ACE inhibitors. N Engl J Med. 2006;354:2443–2453.
- Sipahi I, Debanne SM, Rowland DY, Simon DI, Fang JC. Angiotensin-receptor blockade and risk of cancer: Meta-analysis of randomised controlled trials. Lancet Oncol. 2010;11:627–636.
- Fogari R, Zoppi A. New class of agents for treatment of hypertension: Focus on direct renin inhibition. Vasc Health Risk Manag. 2010;6:869–882.
- Alreja G, Joseph J. Renin and cardiovascular disease: Worn-out path, or new direction? World J Cardiol. 2011;3:72–83.
- Krebs C, Hamming I, Sadaghiani S, Steinmetz OM, Meyer-Schwesinger C, Fehr S, . Antihypertensive therapy upregulates renin and (pro)renin-receptor in the clipped kidney of Goldblatt hypertensive rats. Kidney int. 2007;72:725–730.
- Murray BA, FitzGerald RJ. Angiotensin converting enzyme inhibitory peptides derived from food proteins: Biochemistry, bioactivity and production. Curr Pharm Des. 2007;13: 773–791.
- Guang C, Phillips RD. Plant food-derived angiotensin I converting enzyme inhibitory peptides. J Agric Food Chem. 2009;57:5113–5120.
- Boelsma E, Kloek J. IPP-rich milk protein hydrolysate lowers blood pressure in subjects with stage 1 hypertension, a randomized controlled trial. Nutr J. 2010;9:52–58.
- Boelsma E, Kloek J. Lactotripeptides and antihypertensive effects: A critical review. Br J Nutr. 2009;101:776–786.
- Engberink MF, Schouten EG., Kok FJ, van Mierlo LA, Brouwer IA, Geleijnse JM. Lactotripeptides show no effect on human blood pressure. Results from a double-blind randomized controlled trial. Hypertension. 2008;51:399–405.
- van der Zander K, Bots ML, Bak AAA, Koning MM, de Leeuw PW. Enzymatically hydrolyzed lactotripeptides do not lower blood pressure in mildly hypertensive subjects. Am J Clin Nutr. 2008;88:1697–1702.
- van Mierlo LAJ, Koning MMG, van der Zander K, Draijer R. Lactotripeptides do not lower ambulatory blood pressure in untreated whites: Results from 2 controlled multicenter crossover studies. Am J Clin Nutr. 2009;89:617–623.
- Bradford C, Ely DR, Raizada MK. Targeting the vasoprotective axis of the renin–angiotensin system: A new strategic approach to pulmonary hypertensive therapy. Curr Hypertens Rep. 2010;12:212–219.
- Hernandez Prada JA, Ferreira AJ, Katovich MJ, Shenoy V, Qi Y, Santos RA, . Structure based identification of small-molecule angiotensin-converting enzyme 2 activators as novel antihypertensive agents. Hypertension. 2008;51:1312–1317.
- Gjymishka A, Kulemina LV, Shenoy V, . Diminazene aceturate is an ACE2 activator and a novel antihypertensive drug. FASEB J. 2010;24:1032–1033.
- Goo Y, Terkawi MA, Jia H, Aboge GO, Ooka H, Nelson B, . Artesunate, a potential drug for treatment of Babesia infection. Parasitol Int. 2010;59:481–486.
- Kuba K, Imai Y, Ohto-Nakanishi T, Penninger JM. Trilogy of ACE2: A peptidase in the renin–angiotensin system, a SARS receptor, and a partner for amino acid transporters. Pharm Ther. 2010;128:119–128.
- Glowacka I, Bertram S, Herzog P, Pfefferle S, Steffen I, Muench MO, . Differential downregulation of ACE2 by the spike proteins of severe acute respiratory syndrome coronavirus and human coronavirus NL63. J Virol. 2010;84: 1198–1205.
- Luhtala S. Activities of angiotensin-converting enzymes (ACE1, ACE2) and inhibition by bioactive lactotripeptides (IPP, VPP, LPP) in porcine ocular tissues. Master's thesis. University of Jyvaskyla; 2009.
- Castrop H, Hocherl K, Kurtz A, Schweda F, Todorov V, Wagner C. Physiology of kidney renin. Physiol Rev. 2010;90: 607–673.
- Jan Danser AH, Deinum J. Renin, prorenin and the putative (pro)renin receptor. Hypertension. 2005;46:1069–1076.
- Schmieder RE. Usefulness of direct renin inhibition with aliskiren as antihypertensive treatment. Hipertens riego vasc. 2010;27:245–248.
- Rahuel J, Rasetti V, Maibaum J, Rüeger H, Göschke R, Cohen NC, . Structure-based drug design: The discovery of novel nonpeptide orally active inhibitors of human renin. Chem Biol. 2000;7:493–504.
- Wood JM, Maibaum J, Rahuel J, Grütter MG, Cohen NC, Rasetti V, . Structure-based design of aliskiren, a novel orally effective renin inhibitor. Biochem Bioph Res Co. 2003;308:698–705.
- Politi A, Durdagi S, Moutevelis-Minakakis M, Kokotos G, Mavromoustakos T. Development of accurate binding affinity predictions of novel renin inhibitors through molecular docking studies. J Mol Graph Model. 2010;29:425–435.
- Politi A, Leonis G, Tzoupis H, Ntountaniotis D, Papadopoulos MG, Grdadolnik SG, . Conformational properties and energetic analysis of aliskiren in solution and receptor site. Mol Inf. 2011;30:973–985.
- Jadhav M, Yeola C, Zope G, Nabar A. Aliskiren, the first direct renin inhibitor for treatment of hypertension: The path of its development. J Postgrad Med. 2012;58:32–38.
- Sealey JE, Laragh JH. Aliskiren, the first renin inhibitor for treating hypertension: Reactive renin secretion may limit its effectiveness. Am J Hypertens. 2007;20:587–597.
- Parving HH, Persson F, Lewis JB, Lewis EJ, Hollenberg NK. Aliskiren combined with losartan in type 2 diabetes and nephropathy. N Engl J Med. 2008;358:2433–2446.
- McMurray JJ, Pitt B, Latini R, Maggioni AP, Solomon SD, Keefe DL, . Effects of the oral direct renin inhibitor aliskiren in patients with symptomatic heart failure. Circ Heart Fail. 2008;1:17–24.
- Solomon SD, Appelbaum E, Manning WJ, Verma A, Berglund T, Lukashevich V, . Effect of the direct renin inhibitor aliskiren, the angiotensin receptor blocker losartan, or both on left ventricular mass in patients with hypertension and left ventricular hypertrophy. Circulation. 2009;119:530–537.
- Scirica BM, Morrow DA, Bode C, Ruzyllo W, Ruda M, Oude Ophuis AJ, . Patients with acute coronary syndromes and elevated levels of natriuretic peptides: The results of the AVANT GARDE-TIMI 43 Trial. Eur Heart J. 2010;31: 1993–2005.
- Solomon SD, Shin SH, Shah A, Skali H, Desai A, Kober L, . Effect of the direct renin inhibitor aliskiren on left ventricular remodeling following myocardial infarction with systolic dysfunction. Eur Heart J. 2011;32:1227–1234.
- McMurray JJ, Abraham WT, Dickstein K, Køber L, Massie BM, Krum H. Aliskiren, ALTITUDE, and the implications for ATMOSPHERE. Eur J Heart Fail. 2012;14:341–343.
- The ONTARGET Investigators. Telmisartan, ramipril, or both in patients at high risk for vascular events. N Engl J Med. 2008;358:1547–1559.
- Yokokawa F, Maibaum J. Recent advances in the discovery of non-peptidic direct renin inhibitors as antihypertensives: New patent applications in years 2000–2008. Expert Opin Ther.2008;18:581–602.
- Speedel Holding Ltd. New pre-clinical and clinical data for SPP635 and SPP301. Proceedings of the Hypertension 2008 Medical Congress, 14–19 June 2008, Berlin, Germany.
- Nguyen G, Delarue F, Burckle C, Bouzhir L, Giller T, Sraer JD. Pivotal role of the renin/prorenin receptor in angiotensin II production and cellular responses to renin. J Clin Invest. 2002;109:1417–1427.
- Nabi AH, Biswas KB, Nakagawa T, Ichihara A, Inagami T, Suzuki F. Prorenin has high affinity multiple binding sites for (pro)renin receptor. Biochim Biophys Acta. 2009;1794: 1838–1847.
- Suzuki F, Hayakawa M, Nakagawa T, Nasir UM, Ebihara A, Iwasawa A, . Human prorenin has “gate and handle” regions for its non-proteolytic activation. J Biol Chem. 2003;278:22217–22222.
- Batenburg WW, Jan Danser AH. (Pro)renin and its receptors: Pathophysiological implications. Clin Sci. 2012;123: 121–133.
- Nguyen G, Danser AH. Prorenin and (pro)renin receptor: A review of available data from in vitro studies and experimental models in rodents. Exp Physiol. 2008;93:557–563.
- Huang Y, Wongamorntham S, Kasting J, McQuillan D, Owens RT, Yu L, . Renin increases mesangial cell transforming growth factor-beta1 and matrix proteins through receptor-mediated, angiotensin II-independent mechanisms. Kidney Int. 2006;69:105–113.
- Saris JJ, ‘t Hoen PAC, Garrelds IM, Dekkers DH, den Dunnen JT, Lamers JM, . Prorenin induces intracellular signalling in cardiomyocytes independently of angiotensin II. Hypertension. 2006;48:564–571.
- Huang Y, Noble NA, Zhang J, Xu C, Border WA. Renin-stimulated TGF-beta1 expression is regulated by a mitogen-activated protein kinase in mesangial cells. Kidney Int. 2007;72:45–52.
- Schefe JH, Menk M, Reinemund J, Effertz K, Hobbs RM, Pandolfi PP, . A novel signal transduction cascade involving direct physical interaction of the renin/prorenin receptor with the transcription factor promyelocytic zinc finger protein. Circ Res. 2006;99:1355–1366.
- Biswas KB, Nabi AHMN, Arai Y, Nakagawa T, Ebihara A, Ichihara A, . Aliskiren binds to renin and prorenin bound to (pro)renin receptor in vitro. Hypertension Res. 2010;33:1053–1059.
- Feldman DL, Jin L, Xuan H, Contrepas A, Zhou Y, Webb RL, . Effects of aliskiren on blood pressure, albuminuria, and (pro)renin receptor expression in diabetic TG(mRen-2)27 rats. Hypertension. 2008;52:130–136.
- Feldt S, Batenburg WW, Mazak I, Maschke U, Wellner M, Kvakan H, . Prorenin and renin-induced extracellular signal-regulated kinase 1/2 activation in monocytes is not blocked by aliskiren or the handle-region peptide. Hypertension. 2008;51:682–688.
- Feldt S, Maschke U, Dechend R, Luft FC, Muller DN. The putative (pro)renin receptor blocker HRP fails to prevent (pro)renin signaling. J Am Soc Nephrol. 2008;19:743–748.
- Schefe JH, Neumann C, Goebel M, Danser J, Kirsch S, Gust R, . Prorenin engages the (pro)renin receptor like renin and both ligand activities are unopposed by aliskiren. J Hypertens. 2008;26:1787–1794.
- Ferri N, Greco CM, Maiocchi G, Corsini A. Aliskiren reduces prorenin receptor expression and activity in cultured human aortic smooth muscle cells. J Renin-Angio-Aldo S. 2011; 12:469–474.
- Jan Danser AH, Batenburg WW, van Esch JHM. Prorenin and the (pro)renin receptor-an update. Nephrol Dial Transplant. 2007;22:1288–1292.
- Ichihara A, Hayashi M, Kaneshiro Y, Suzuki F, Nakagawa T, Tada Y, . Inhibition of diabetic nephropathy by a decoy peptide corresponding to the “handle” region for nonproteolytic activation of prorenin. J Clin Invest. 2004;114:1128–1135.
- He M, Zhang L, Shao Y, Wang X, Huang Y, Yao T, . Inhibition of renin/prorenin receptor attenuated mesangial cell proliferation and reduced associated fibrotic factor release. Eur J Pharmacol. 2009;606:155–161.
- Ichihara A, Suzuki F, Nakagawa T, Kaneshiro Y, Takemitsu T, Sakoda M, . Prorenin receptor blockade inhibits development of glomerulosclerosis in diabetic angiotensin II type 1a receptor-deficient mice. J Am Soc Nephrol. 2006;17: 1950–1961.
- Takahashi H, Ichihara A, Kaneshiro Y, Inomata K, Sakoda M, Takemitsu T, . Regression of nephropathy developed in diabetes by (pro)renin receptor blockade. J Am Soc Nephrol. 2007;18:2054–2061.
- Ichihara A, Kaneshiro Y, Takemitsu T, Sakoda M, Nakagawa T, Nishiyama A, . Contribution of nonproteolytically activated prorenin in glomeruli to hypertensive renal damage. J Am Soc Nephrol. 2006;17:2495–2503.
- Ichihara A, Kaneshiro Y, Takemitsu T, Sakoda M, Suzuki F, Nakagawa T, . Nonproteolytic activation of prorenin contributes to development of cardiac fibrosis in genetic hypertension. Hypertension. 2006;47:894–900.
- Kaneshiro Y, Ichihara A, Sakoda M, Takemitsu T, Nabi AH, Uddin MN, . Slowly progressive, angiotensin II- independent glomerulosclerosis in human (pro)renin receptor-transgenic rats. J Am Soc Nephrol. 2007;18:1789–1795.
- Susic D, Zhou X, Frohlich ED, Lippton H, Knight M. Cardiovascular effects of prorenin blockade in genetically spontaneously hypertensive rats on normal and high-salt diet. Am J Physiol Heart Circ Physiol. 2008;295:H1117–H1121.
- Ryuzaki M, Ichihara A, Ohshima Y, Sakoda M, Kurauchi-Mito A, Narita T, . Involvement of activated prorenin in the pathogenesis of slowly progressive nephropathy in the non-clipped kidney of two kidney, one-clip hypertension. Hypertens Res. 2011;34:301–307.
- Nagai Y, Ichihara A, Nakano D, Kimura S, Pelisch N, Fujisawa Y, . Possible contribution of the non-proteolytic activation of prorenin to the development of insulin resistance in fructose-fed rats. Exp Physiol. 2009;94:1016–1023.
- Satofuka S, Ichihara A, Nagai N, Koto T, Shinoda H, Noda K, . Role of nonproteolytically activated prorenin in pathologic, but not physiologic, retinal neovascularization. Invest Ophthalmol Vis Sci. 2007;48:422–429.
- Satofuka S, Ichihara A, Nagai N, Noda K, Ozawa Y, Fukamizu A, . (Pro)renin receptor-mediated signal transduction and tissue renin–angiotensin system contribute to diabetes-induced retinal inflammation. Diabetes. 2009;58: 1625–1633.
- Batenburg WW, Krop M, Garrelds IM, de Vries R, de Bruin RJ, Burcklé CA, . Prorenin is the endogenous agonist of the (pro)renin receptor. Binding kinetics of renin and prorenin in rat vascular smooth muscle cells overexpressing the human (pro)renin receptor. J Hypertens. 2007;25: 2441–2453.
- Muller DN, Klanke B, Feldt S, Cordasic N, Hartner A, Schmieder RE, . (Pro)renin receptor peptide inhibitor “handle-region” peptide does not affect hypertensive nephrosclerosis in Goldblatt rats. Hypertension. 2008;51: 676–681.
- Unger T, Paulis L, Sica DA. Therapeutic perspectives in hypertension: Novel means for renin–angiotensin–aldosterone system modulation and emerging device-based approaches. Eur Heart J. 2011;32:2739–2747.
- Maschke U, Muller DN. The (pro)renin receptor and the mystic HRP—is there a role in cardiovascular disease? Front Biosci (Elite Ed). 2010;2:1250–1253.
- Akunuri S, Christofi F, Wijetunge S, Hughes AD. Effects of prorenin-derived peptides on the proliferation of human cultured saphenous vein smooth muscle cells. In: Abstract Book of the 15th annual European Council for Cardiovascular Research meeting, La Colle sur Loup, France, 8-10.10.2010. p. 41.
- Funke-Kaiser H, Zollmann FS, Schefe JH, Unger T. Signal transduction of the (pro)renin receptor as a novel therapeutic target for preventing end-organ damage. Hypertens Res. 2010;33:98–104.
- Li Q, Raizada MK. Is (pro)renin receptor a multifunctional receptor? Hypertension. 2010;55:1308–1309.
- Advani A, Kelly DJ, Cox AJ, White KE, Advani SL, Thai K, . The (pro)renin receptor: Site-specific and functional linkage to the vacuolar H + -ATPase in the kidney. Hypertension. 2009;54:261–269.
- Cruciat CM, Ohkawara B, Acebron SP, Karaulanov E, Reinhard C, Ingelfinger D, . Requirement of prorenin receptor and vacuolar H + -ATPase-mediated acidification for Wnt signaling. Science. 2010;327:459–463.
- Takahashi S, Tokiwano T, Suzuki N, Kodama I, Yoshizawa Y, Gotoh T. Renin inhibitory activity in rice and cereals. J Biol Macromol. 2010;10:83–91.
- Takahashi S, Tokiwano T, Hata K, Kodama I, Hokari M, Suzuki N, . The occurrence of renin inhibitor in rice: Isolation, identification, and structure-functional relationship. Biosci Biotechnol Biochem. 2010;74:1713–1715.
- Fischer R, Dechend R, Qadri F, Markovic M, Feldt S, Herse F, . Dietary n-3 polyunsaturated fatty acids and direct renin inhibition improve electrical remodeling in a model of high human renin hypertension. Hypertension. 2008;51:540–546.
- Takahashi S, Hori K, Kumagai M, Wakabayashi A. Human renin inhibitory activity in legumes. J Biol Macromol. 2007;7:49–54.
- Takahashi S, Hori K, Shinbo M, Hiwatashi K, Gotoh T, Yamada S. Isolation of human renin inhibitor from soybean: Soyasaponin I is the novel human renin inhibitor in soybean. Biosci Biotechnol Biochem. 2008;72:3232–7236.
- Yuan L, Wu J, Aluko RE, Ye X. Kinetics of renin inhibition by sodium houttuyfonate analogs. Biosci Biotechnol Biochem. 2006;70:2275–2280.
- Takahashi S, Hori K, Hokari M, Gotoh T, Sugiyama T. Inhibition of human renin activity by saponins. Biomed Res. 2010;31:155–159.
- Hiwatashi K, Shirakawa H, Hori K, Yoshiki Y, Suzuki N, Hokari M, . Reduction of blood pressure by soybean saponins, renin inhibitors from soybean, in spontaneously hypertensive rats. Biosci Biotechnol Biochem. 2010;74: 2310–2312.