Abstract
Background. Sodium overload is related to the development of primary hypertension and its complications. Methods. In 131 (65 female) treated hypertensives (average blood pressure 144/82 mmHg and duration of hypertension 11.7 years), we measured peripheral and central arterial pressures, peripheral (AIxP) and central (AIxC1, AIxC2) augmentation indices, pulse-wave velocity (PWV) and daily urinary sodium excretion, and conducted genetic studies of ACE D/I and CYP11B2 C-344T polymorphisms. Proximal (FELi) and distal (FDRNa) sodium reabsorption measurements were performed using endogenous lithium clearance. Results. We found statistically significant interactions between FELi and ACE D/I polymorphism with respect to AIxC2 (PINT = 0.05) and between FELi and CYP11B2 C-344T polymorphism with respect to AIxC1 (PINT = 0.01), AIxC2 (PINT = 0.04) and AIxP (PINT = 0.01). In the group of ACE I allele carriers compared with DD homozygotes, the AIxC1 (154.1 vs 140.6%; p = 0.02), AIxC2 (33.3 vs 26.9%; p = 0.02) and AIxP (94.6 vs 85.2%; p = 0.01) were higher in the subgroup with FELi below the median value (FELi1), but not in the subgroup with FELi above the median value (FELi2). In the group of CYP11B2 TT homozygotes compared with C allele carriers, we observed higher values of AIxC1 (158.5 vs 146.4%; p = 0.03), AIxC2 (36.0 vs 29.4%; p = 0.01) and AIxP (99.0 vs 88.7%; p = 0.005) in the FELi1 but not the FELi2 subgroup. Conclusions. In the population with assumed high dietary sodium intake and long-standing history of hypertension, the relation between proximal sodium reabsorption and the development of arterial stiffness depends on the genetic context of the selected genetic polymorphisms of the renin—angiotensin—aldosterone system, independent of blood pressure.
Introduction
Interaction between genetic and environmental factors may predispose to the development of hypertension and related target organ complications. Genetic predisposition to certain types of sodium-sensitive hypertension may result from mutations of cytoskeleton proteins, ion channels or hormones taking part in sodium metabolism, and thus in the regulation of extracellular fluid volume, leading to impaired glomerular filtration rate (GFR) or increased tubular reabsorption of sodium (Citation1,Citation2). Sodium overload contributes, independently of blood pressure, to the development of cardiovascular complications, including arterial stiffness, which is assessed using pulse-wave velocity (PWV) (Citation3), aortic pulse-wave augmentation index (AIx) (Citation4), left ventricular (LV) hypertrophy (Citation5) and LV diastolic dysfunction (Citation6). As regards the genetic factors, much research interest has been focused on the relations between selected polymorphisms of the genes encoding key enzymes involved in the synthesis of the products of the renin—angiotensin—aldosterone (RAA) system, and parameters of blood pressure and cardiovascular remodeling, particularly in the context of the effects of sodium on modulation of gene expression and combined phenotypic effects (Citation7–9). This particularly included D/I polymorphism of the ACE gene encoding angiotensin-converting enzyme (ACE) and C-344T polymorphism of the CYP11B2 gene encoding aldosterone synthase.
Endogenous lithium clearance has been used as a measure of sodium reabsorption in proximal and distal renal tubules. Serum and urinary lithium levels calculated on the basis of spectrophotometric measurements allow estimation of fractional urinary lithium excretion (FELi). As lithium ions are transported across the proximal nephron cell membrane using the same cellular mechanisms as sodium ions, FELi is an accurate marker of sodium reabsorption in proximal tubules. The values of FELi and fractional urinary sodium excretion (FENa) also allow the calculation of fractional sodium reabsorption in distal tubules (FDRNa) (Citation10). Numerous studies based on the assessment of endogenous lithium clearance methodology have demonstrated a strict correlation between the increased sodium reabsorption in the proximal tubules and blood pressure measured using conventional methods (Citation10,Citation11), ambulatory blood pressure monitoring (ABPM) (Citation12), parameters of stiffness of the walls of large arteries (Citation13) and LV hypertrophy (Citation14).
The aim of this study was to assess the relation of the ACE D/I polymorphism and CYP11B2 C-344T polymorphism with blood pressure and arterial stiffness parameters, as well as to assess the relation and combined effects of genetic factors and sodium metabolism parameters measured using endogenous lithium clearance with blood pressure and arterial stiffness parameters.
Materials and methods
Study population
The study was approved by the Bioethical Committee of the Jagiellonian University (KBET/141/B/2009). From April 2010 to May 2013, within the framework of a National Research Centre grant (no. N N402 533239), we enrolled 140 hypertensive patients followed up at the reference hypertension clinic of the University Hospital in Krakow. All patients were requested to complete a standard epidemiological questionnaire including detailed medical history, use of antihypertensive medications, and socioeconomic and demographic characteristics. The patients provided written informed consent to participate in the study. Patients with a history of malignancy, decompensated chronic diseases, cardiomyopathy of unknown etiology, hemodynamically significant valvular heart disease or secondary hypertension were excluded from the study. To avoid interference between sodium excretion/reabsorption and the use of diuretic agents, only patients not receiving long-term diuretic treatment or patients in whom hypertension was sufficiently well controlled to allow discontinuation of diuretic treatment at least 5 days before the enrollment were included in the study. The 5 day washout period was deemed sufficient to eliminate an influence of diuretics on the analyzed parameters (Citation15).
Nine patients were excluded from the statistical analysis: four because of diuretic treatment within 5 days before the 24 h urine collection, three because of failure to complete the urine collection and two because of high serum lithium levels (> 2.0 μmol/l) and urinary lithium levels (> 25.0 μmol/l) that may have indicated external contamination or high dietary lithium intake. Thus, 131 subjects were included in the statistical analysis.
Blood pressure and vascular stiffness measurements
Blood pressures were measured using the Omron HEM-705 device (Omron Healthcare, Kyoto, Japan). In every patient the measurements were performed on the non-dominant arm in a sitting position, after a minimum 10 min rest; blood pressure values were calculated as the mean of three measurements. Pulse pressure (PP) was calculated as the difference between the mean systolic blood pressure (SBP) and the mean diastolic blood pressure (DBP), and the mean blood pressure (MBP) was calculated as the sum of DBP and one-third of PP.
Arterial properties were measured by one observer, after the patient had had a 15 min rest, and included central pulse-wave analysis (PWA) (PWA module of the SphygmoCor 6.31 device; AtCor Medical, West Ryde, New South Wales, Australia) and PWV (PWV module of the Complior device; Colson, Paris, France). PWA recordings were made in 8 s periods using peripheral arterial tonometry. The measurements were taken on the radial artery of the non-dominant upper extremity using an SPC-301 micromanometer (Millar Instruments, Houston, TX, USA). The following parameters were considered in the statistical analyses: blood pressure parameters, including central systolic blood pressure (SBPC), central diastolic blood pressure (DBPC), central mean blood pressure (MBPC) and central pulse pressure (PPC); and arterial stiffness parameters, including aortic pulse-wave augmentation (AG) and peripheral (AIxP) and central (AIxC) aortic pulse-wave augmentation indices. AG (mmHg) was calculated as the difference between the first and second systolic peaks on the ascending arm of the aortic pulse wave. AIxP (%) and AIxC1 (%) were calculated as the quotient of the first and second systolic peaks on the ascending arm of the radial and aortic pulse wave, and AIxC2 (%) was calculated as the product of AG and PPC. The averaged pulse waves showing less than 5% difference in the height and width of systolic and diastolic portions or greater than 80 mV amplitude of the wave signal were classified as normal. PWV (m/s) was measured between the carotid and femoral arteries. Mean values of 10 consecutive measurements were included in the statistical analysis.
Echocardiography
Structural and functional measurements of LV myocardium were performed using transthoracic, two-dimensional echocardiography with Doppler measurements (Vivid 4, General Electric, Wauwatosa, WI, USA), with a 2.5–3.5 MHz transducer. Pulsed-wave Doppler with a gate positioned on the mitral valve was used to assess the early (E) and late (A) mitral inflow, their proportion (E/A) and the E-wave deceleration time. The isovolumetric relaxation time was measured as the time from the end of aortic inflow to the beginning of the mitral inflow. The tissue Doppler was used for the measurements of the movements of the mitral annulus with a gate positioned on the basal segment of the ventricular septum. These measurements included early diastolic velocity (e′), maximum velocity after atrial contraction (a′) and their proportion (e′/a′); the E/e′ index was also calculated. The LV ejection fraction was assessed using the Simpson method. Stroke volume was calculated using planimetry in the four-chamber apical view.
Clinical measurements
Anthropometric measurements, including height, body weight, waist circumference and hip circumference, were obtained for every patient. Body mass index (BMI) was calculated as the ratio of the body weight (kg) and the square of the height (m). Waist/hip ratio (WHR) was calculated as the ratio of the waist and hip circumferences. On the morning of the cardiovascular measurements, a fasting blood sample was obtained from each patient for serum measurements of glucose, glycosylated hemoglobin A1c, serum lipid levels, N-terminal natriuretic propeptide B, sodium, potassium, creatinine and lithium. One day before, the patients recruited to the study completed a 24 h urine collection to measure 24 h excretion of sodium (Na24), potassium, creatinine, lithium and albumin.
Renal sodium handling and genetic studies
We measured serum and urinary lithium concentrations necessary for the calculations of lithium clearance with atomic absorption spectrometry (AAS) using the 5100ZL AAS equipment (Perkin-Elmer Inc., Waltham, MA, USA), and adapting the methods of analysis previously described (Citation19,Citation20). Urinary lithium levels were detected using the flame injection AAS (FI-AAS) and the graphite furnace AAS (GF-AAS) for urine measurements in the case of lithium concentrations below 10 μg/l. Serum lithium concentrations were detected with GF-AAS. The analytic parameters of FI-AAS in urine were: limit of detection (LOD), 1.4 μg/l; precision at Li concentration of 25.0 μg/l, 5%; recovery, 95–100%. The analytic parameters of GF-AAS for urine samples were: LOD, 0.9 μg/l; precision at Li concentration of 10.0 g/l, 5; recovery, 94%; and for serum: LOD, 0.4–0.8 μg/l; precision at Li concentration of 5.0 g/l, 9%; recovery, 96%. Creatinine, sodium and lithium clearances (C) were calculated using the formula: Cx = V*Ux/Px, where V (ml/min) is 24 h urine volume calculated per minute, and Ux and Px are molar creatinine, sodium or lithium concentrations in urine and serum, respectively. High lithium clearance or a small difference between the lithium and sodium clearances indicates increased capacity for renal excretion of excessive sodium loads. Fractional sodium excretion (FENa) and fractional lithium excretion (FELi) were expressed as percentages derived from the product of sodium or lithium clearance, respectively, and creatinine clearance: FEx (%) = 100*Cx//CCR, where x is lithium or sodium. Since lithium is transported across the membranes of proximal renal tubular cells using the same pathways as sodium ions, FELi is a very accurate marker of GFR-dependent sodium reabsorption in proximal tubules. Low FELi indicates higher sodium and water load reabsorbed in the proximal tubule, which results in a lower sodium and water load filtered to the distal tubule. Measurements of both FELi and FENa allow for estimating the fractional distal sodium reabsorption (FDRNa) using the formula: FDRNa = [(FELi–FENa)/FELi] × 100. FDRNa is an estimate of sodium that is not reabsorbed in the proximal tubule, but undergoes reabsorption in the post-proximal segments of the renal tubules. High FDRNa indicates higher sodium and water load reabsorbed in the distal tubule, which results in a lower sodium and water load that is filtered and subsequently excreted with urine (Citation16).
Genetic studies were performed at the Department of Neurogenetics, Chair of Neurology of the Jagiellonian University using peripheral blood collected on ethylenediaminetetraacetic acid (EDTA). Genomic DNA was isolated using the QIAGEN QIAamp DNA Blood Mini Kit, Germantown, MD, USA. The patients were genotyped for ACE D/I polymorphism by the polymerase chain reaction (PCR) method described by Lindpaintner et al. (Citation17). The CYP11B2 C-344T polymorphism was assessed by real-time PCR using the ViiA 7 Real Time PCR System (Life Technologies, Carlsbad, CA, USA).
Statistical analysis
Database management and statistical analyses were performed using the SAS System 9.3 software (SAS Institute, Cary, NC, USA). The distributions of the analyzed quantitative variables were compared with the normal distribution using the Shapiro—Wilk and Kolmogorov—Smirnov tests. In the descriptive statistics the quantitative data were expressed as mean values and standard deviations (for data with a normal distribution) or as median values and interquartile ranges (for data that did not fulfill the criteria of the normal distribution). The qualitative data were expressed as proportions. For comparisons of the mean values in the groups of patients the Student's t test was used. Alternatively, Wilcoxon and median tests were used in the case of the skewed distribution of quantitative variables in the subgroups. The chi-squared Pearson's test or Fisher's test was used for qualitative variables. The correlations of quantitative sodium parameters were analyzed using the standardized Spearman's correlation analysis. Simple linear regression analysis was performed to identify the factors associated with the analyzed sodium parameters (FELi, FDRNa, Na24).
The conformance of the distributions of the analyzed genotypes with the distributions estimated using the Hardy—Weinberg principle was confirmed using the chi-squared Pearson's test. The selected parameters of blood pressure and arterial stiffness were compared for the ACE D/I polymorphism and the CYP11B2 C-344T polymorphism using variance analysis in the whole study group and separately for male and female patients. Then, a multifactorial regression model (PROC GENMOD module of the SAS software) (Citation18) was applied to include the dependent variables and to calculate the standardized mean values and standard measurement error for the respective genotypes and allele carriers. In the subsequent analyses, a multifactorial regression model was applied to analyze the interactions between genetic and environmental factors with respect to blood pressure and parameters of arterial stiffness.
Results
Characteristics of the study population
The study population consisted of 131 patients, including 66 men (50.3%) and 65 women (49.7%). The mean time from establishing the diagnosis of hypertension to enrollment in the study was 11.7 years. The 24 h sodium intake assessed based on 24 h urine collection was 193.9 ± 95.3 mmol. Clinical, biochemical and medication characteristics of the study group are summarized in , and Supplementary Table 1 (see supplementary material online to be found online at http://informahealthcare.com/doi/abs/10.3109/08037051.2015.1070476), respectively.
Table I. Clinical characteristics and arterial stiffness parameters in the study population.
Table 2. Biochemical studies in serum and 24 h urine and the parameters obtained using endogenous lithium clearance in the study population.
Factors determining the peripheral and central blood pressure values and arterial stiffness parameters including PWV, AIxC1, AIxC2 and AIxP were established using simple linear regression. In the study group, PWV showed positive relations with age (β = 0.09, SE = 0.03; p = 0.01), WHR (β = 0.06, SE = 0.03; p = 0.03), SBP (β = 0.07, SE = 0.01; p = 0.001) and LV dysfunction (β = 1.19, SE = 0.56; p = 0.03). AIxC1 showed positive relations with age (β = 0.70, SE = 0.27; p = 0.01), female gender (β = 12.25, SE = 3.87; p = 0.002) and SBP (β = 0.18, SE = 0.09; p = 0.05), and a negative relation with WHR (β = –0.58, SE = 0.23; p = 0.01). AIxC2 showed positive relations with age (β = 0.35, SE = 0.12; p = 0.004), female gender (β = 5.37, SE = 1.69; p = 0.002) and SBP (β = 0.09, SE = 0.04; p = 0.04), and a negative relation with WHR (β = –0.29, SE = 0.10; p = 0.04). AIxP showed positive relations with age (β = 0.42, SE = 0.16; p = 0.008), female gender (β = 8.23, SE = 2.23; p = 0.0003) and SBP (β = 0.12, SE = 0.05; p = 0.02), and a negative relation with WHR (β = –0.42, SE = 0.13; p = 0.002).
The distributions of the analyzed polymorphisms of the ACE and CYP11B2 genes were consistent with the Hardy—Weinberg principle: ACE D/I polymorphism (p = 0.52) and CYP11B2 C-344T polymorphism (p = 0.54). The respective frequencies of the genotypes of the ACE D/I polymorphism were as follows: DD genotype (27.5%), DI genotype (45.8%) and II genotype (26.7%), while the respective frequencies of the D and I allele were 50.7% and 49.3%, respectively. The respective frequencies of the genotypes of the CYP11B2 C-344T polymorphism were as follows: CC genotype (25.2%), CT genotype (54.2%), TT genotype (20.6%), C allele (52.3%) and T allele (47.7%).
Relationships of the ACE D/I polymorphism with peripheral and central blood pressures and arterial stiffness parameters
For ACE D/I polymorphism, we found no statistically significant differences between the patients with various genotypes of the polymorphisms and the peripheral (all p ≥ 0.06) and central (all p ≥ 0.10) blood pressures. Nevertheless, statistically significant differences between the analyzed genotypes were observed with respect to AIxC1 and AIxC2 in male patients (Supplementary Table 2 to be found online at http://informahealthcare.com/doi/abs/10.3109/08037051.2015.1070476). Comparative analyses of the respective genotypes revealed significantly higher values of AIxC1 in the whole study group (155.3 ± 3.6 vs 143.3 ± 3.5%; p = 0.02) and in male patients (154.3 ± 4.9 vs 136.5 ± 4.7%; p = 0.009), AIxC2 (33.9 ± 1.6 vs 29.0 ± 1.5%; p = 0.02) in the whole study group and in male patients (33.1 ± 2.2 vs 25.7 ± 2.1%; p = 0.01), and AIxP in male patients (90.3 ± 2.6 vs 82.6 ± 3.6%; p = 0.04), in patients with genotype II compared with patients with genotype DD.
No statistically significant interactions between the respective polymorphisms and gender were found in the study group with respect to peripheral blood pressures (PINT ≥ 0.73), central blood pressures (PINT ≥ 0.84) and arterial stiffness parameters (PINT ≥ 0.43).
Relationships between the ACE D/I polymorphism and sodium parameters with respect to peripheral and central blood pressures and arterial stiffness parameters
To demonstrate the relationships between sodium parameters and the genotypes of the ACE D/I polymorphism and their combined influence on blood pressure and arterial stiffness parameters, we analyzed the interactions between the ACE D/I polymorphism and sodium parameters, including FELi, FDRNa and Na24. In the study group we revealed statistically significant relationships between the ACE D/I polymorphism and FELi with respect to AIxC2 (PINT = 0.05), but not with respect to PWV (PINT = 0.19), AIxC1 (PINT = 0.17) and AIxP (PINT = 0.91), or to peripheral (PINT = 0.61) and central blood pressures (PINT = 0.07). Moreover, in the analyses of the relationships with RNadist (PINT ≥ 0.15) and Na24 (PINT ≥ 0.12), no statistically significant interactions were observed with respect to arterial stiffness parameters.
Thus, the subsequent analyses of the relationships between arterial stiffness parameters and the ACE D/I polymorphism were conducted using multifactorial regression analysis for the subgroups with FELi below and above the median value (Supplementary Table 3 to be found online at http://informahealthcare.com/doi/abs/10.3109/08037051.2015.1070476). In patients with the II genotype compared with patients with the DD genotype, for the subgroups with FELi below the median value (representing the patients with increased proximal sodium reabsorption), significantly higher values of AIxC1 (156.6 ± 5.1 vs 140.7 ± 4.6%; p = 0.02), AIxC2 (34.7 ± 2.5 vs 27.0 ± 2.2%; p = 0.02) and AIxP (95.4 ± 3.5 vs 85.2 ± 3.1%; p = 0.03) were observed, in contrast to the subgroup with FELi above the median value. The analyses of the relationships of arterial stiffness parameters in the ACE DD homozygotes and the I allele carriers in the whole study group and in the subgroups with FELi below and above the median value are presented in . In the subgroup with FELi below the median value, AIxC1 was 13.5% higher (p = 0.02), AIxC2 was 6.4% higher (p = 0.02) and AIxP was 9.4% higher (p = 0.01) in the I allele carriers compared with the DD homozygotes.
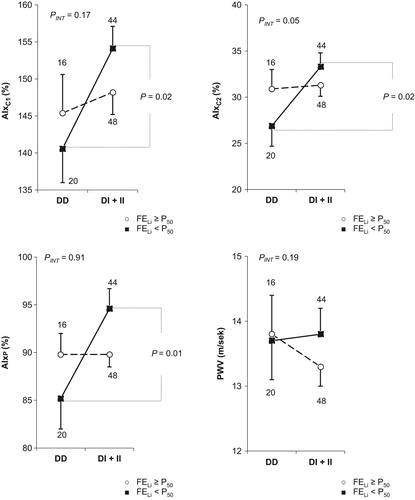
Comparative analyses of the relationships between arterial stiffness parameters and the ACE D/I polymorphism were also conducted in the subgroups with FNadist and Na24 below and above the median values, and no significant differences were observed (Supplementary Tables 4 and 5 to be found online at http://informahealthcare.com/doi/abs/10.3109/08037051.2015.1070476).
Relationships of the CYP11B2 C-344T polymorphism with peripheral and central blood pressures and arterial stiffness parameters
The analyses of the relationships of the CYP11B2 C-344T polymorphism, conducted both in the whole study group and in the subgroups of male and female patients, revealed no significant differences between the patients with various genotypes of the polymorphism with respect to the peripheral (p ≥ 0.17) and central (p ≥ 0.55) blood pressures or to PWV (p ≥ 0.10), AIxC1 (p ≥ 0.24), AIxC2 (p ≥ 0.46) and AIxP (p ≥ 0.16) values. Moreover, in the analyses comparing TT homozygotes and C allele carriers, both in the whole study group and in the subgroups of male and female patients, no statistically significant differences in blood pressures and arterial stiffness parameters were found, except for the marginally higher AIxP values in TT homozygotes both in the whole study group and in male patients (Supplementary Table 6 to be found online at http://informahealthcare.com/doi/abs/10.3109/08037051.2015.1070476).
Relationships between CYP11B2 C-344T polymorphism and sodium parameters with respect to peripheral and central blood pressures and arterial stiffness parameters
To demonstrate the relationships between sodium parameters and the expression of the CYP11B2 C-344T polymorphism and their combined influence on blood pressure and arterial stiffness parameters, we analyzed the interactions between the CYP11B2 C-344T polymorphism (analyzed as TT homozygotes and C allele carriers) and sodium parameters, including FELi, RNadist and Na24. In the study group, we revealed statistically significant interactions between the TT homozygote status and FELi with respect to AIxC1 (PINT = 0.01), AIxC2 (PINT = 0.04) and AIxP (PINT = 0.01), but not with respect to PWV (PINT = 0.55) and peripheral (PINT = 0.20) and central (PINT = 0.24) blood pressures. Moreover, the analyses of the relationships between the CYP11B2 TT homozygote status and RNadist revealed statistically significant interactions with respect to AIxC2 (PINT = 0.05) and AIxP (PINT = 0.02), but not with respect to AIxC1 (PINT = 0.12), PWV (PINT = 0.16) or peripheral (PINT = 0.48) and central (PINT = 0.09) blood pressures. The analyses of the interactions with Na24 revealed no statistically significant interactions with respect to arterial stiffness parameters (PINT ≥ 0.41).
Thus, the subsequent analyses of the relationships between arterial stiffness parameters and the TT homozygote status and the C allele carrier status were conducted using multifactorial regression analysis for the subgroups with FELi below and above the median value. In the subgroups with FELi below the median value (representing the patients with increased proximal sodium reabsorption), AIxC1 values were 12.1% higher (158.5 ± 4.7 vs 146.4 ± 2.9%; p = 0.03), AIxC2 values were 6.6% higher (36.0 ± 2.2 vs 29.4 ± 1.4%; p = 0.01) and AIxP values were 10.3% higher (99.0 ± 3.1 vs 88.7 ± 1.9%; p = 0.005) in the TT homozygotes compared with the C allele carriers, in contrast to the subgroup with FELi above the median value. The analyses of the relationships of arterial stiffness parameters in the TT homozygotes and the C allele carriers in the whole study group and in the subgroups with FELi below and above the median value are presented in .
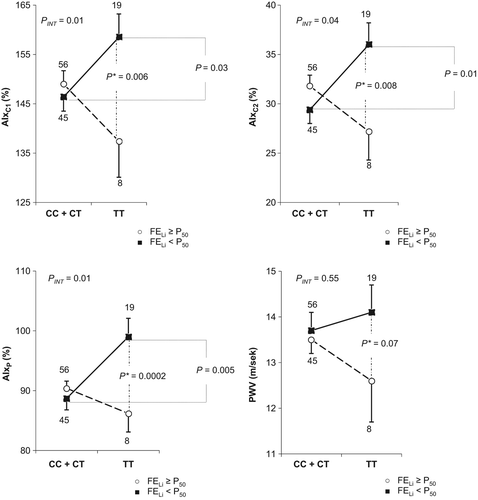
Similar analyses were conducted the subgroups with FDRNa below and above the median values. After the standardization for the dependent variables, in the subgroups with FDRNa below the median value (representing the patients with decreased distal sodium reabsorption), significantly higher AIxC1 (155.1 vs 143.7%; p = 0.02), AIxC2 (34.2 vs 28.3%; p = 0.01) and AIxP (95.9 vs 85.4%; p = 0.001) values were observed in the TT homozygotes compared with the C allele carriers, in contrast to the subgroup with FDRNa above the median value. The analyses of the relationships between the parameters of arterial stiffness in the TT homozygotes and C allele carriers in the whole study group and in the subgroups with FDRNa below and above the median value are presented in Supplementary Tables 7 to be found online at http://informahealthcare.com/doi/abs/10.3109/08037051.2015.1070476.
Despite the absence of statistically significant interactions between the CYP11B2 C-344T polymorphism and Na24 with respect to arterial stiffness parameters, we conducted comparative analyses of the subgroups with Na24 above and below the median values. After standardization of the dependent variables, no significant differences with respect to arterial stiffness parameters in the TT homozygotes compared with the C allele carriers were observed in the subgroups with Na24 above and below the median value (Supplementary Tables 8 to be found online at http://informahealthcare.com/doi/abs/10.3109/08037051.2015.1070476).
Discussion
This study, conducted in a population characterized by high dietary sodium intake and long-standing history of hypertension, revealed that increased proximal sodium reabsorption may show a relation with the development of arterial stiffness that is related to the expression of the ACE D/I polymorphism and the CYP11B2 C-344T polymorphism and is independent of blood pressure values. In the subgroup of patients with FELi below the median value we observed significantly higher AIxC1, AIxC2 and AIxP in the ACE I allele carriers compared with DD homozygotes; this was not observed in the subgroup of patients with FELi above the median value. Moreover, in the subgroup of patients with FELi below the median value we observed significantly higher AIxC1, AIxC2 and AIxP in the CYP11B2 TT homozygotes compared with C allele carriers. This was not observed in the subgroup of patients with FELi above the median value.
To date, only a few publications that addressed the issues of cardiovascular remodeling and target organ complications of hypertension included endogenous lithium clearance in the methodology. Seidlerová et al. (Citation13), in a study including 1069 untreated subjects from the general population, analyzed the relationship between the proximal sodium reabsorption (FPRNa) and FDRNa values and blood pressures as well as elastic properties (lumen diameter, compliance, distensibility) of the brachial, femoral and carotid arteries. They observed a correlation between the increasing diameter of the femoral and brachial arteries and increased FPRNa, as well as between the impaired compliance and distensibility of the femoral artery and increased FDRNa. In another study, Jin et al. (Citation14) analyzed the parameters of LV hypertrophy in the context of sodium parameters. They revealed an increased left ventricular mass index (LVMI) and an increased 24 h urinary sodium excretion that were independent of other factors. They also analyzed the effects of increased FPRNa and FDRNa in the context of increased LVMI and found no statistically significant correlations; however, the increased FDRNa was related to the increase in the indirect parameters of LV hypertrophy.
Among the analyzed polymorphisms, the most abundant data were obtained for the ACE D/I polymorphism. Numerous studies have provided unequivocal data on the relationship between the ACE D/I polymorphism and the levels of ACE, revealing a strong positive correlation between the presence of the D allele and the serum and tissue ACE levels. Serum ACE levels are approximately 60% higher in the DD homozygotes than in the II homozygotes, with intermediate values found in heterozygotes (Citation19). Ueda et al. (Citation20) demonstrated that intravenous AG I infusion was associated with a significantly higher increase in blood AG II levels in the DD homozygotes compared with the II homozygotes, which is an indirect confirmation of the elevated plasma ACE levels in the DD homozygotes. Some authors argue that the increased serum and tissue ACE levels may predispose to the increased conversion of AG I to AG II, which may result in increased vasoconstriction and remodeling (Citation21). Data obtained in our study group, including patients with a long-standing history of hypertension and high dietary sodium intake, suggest a negative impact of the I allele of the ACE D/I polymorphism on the remodeling of the arterial wall. We demonstrated that this effect is associated with the interaction between the ACE D/I polymorphism and proximal sodium reabsorption. In the subgroup of patients with FELi below the median value, we observed significantly higher values of arterial stiffness parameters in the I allele carriers compared with the DD homozygotes, which was not observed in the subgroup with FELi above the median value. Such interactions were not observed with respect to distal sodium reabsorption and 24 h urinary sodium excretion.
The relationships between the ACE D/I polymorphism and sodium-sensitive hypertension have been analyzed in various populations. In a group of sodium-sensitive Japanese patients with primary hypertension, a significantly higher incidence of the I allele compared with non-sodium-sensitive subjects was demonstrated. Moreover, it was revealed that the II genotype was associated with a significantly higher risk of sodium-sensitive hypertension compared with the DD and DI genotypes (Citation22). Similar data were obtained in a group of Spanish patients with hypertension. Moreover, in subjects consuming a high-sodium diet, the increase in 24 h SBP and DBP was significantly higher in the II homozygotes compared with the DD homozygotes; the increase was intermediate in heterozygotes (Citation7). In the literature, there is one study conducted in three European populations in the framework of the European Project on Genes in Hypertension (EPOGH) project, which revealed no correlation between the ACE D/I polymorphism and 24 h urinary sodium excretion with respect to arterial stiffness parameters (Citation8). Nevertheless, in the subgroup of patients selected from the Polish population, which was characterized by the highest dietary sodium intake (245 ± 87 mmol/day), analysis of the relationships between blood pressure and arterial stiffness parameters and the ACE D/I polymorphism, conducted in the tertiles of 24 h urinary sodium excretion, significantly higher SBP and PP values (obtained by traditional measurements, ABPM and pulse waveform analysis) in the II homozygotes compared with the D allele carriers were observed in the third tertile of 24 h sodium excretion, but not in the first and second tertiles (Citation23). In one of the analyses of the EPOGH, Kuznetsova et al. (Citation9) demonstrated that the relationship between the ACE D/I polymorphism and LVMI was different in various European populations, probably owing to different regulatory mechanisms caused by the differences in dietary salt consumption assessed on the basis of 24 h urinary sodium excretion (subjects of Slavic ethnicity 229 mmol/day vs Italian subjects 186 mmol/day). The authors revealed that in the general population of Slavic ethnicity compared with Italian subjects, the LVMI values were higher in the II homozygotes, while in the Italian population a reverse trend was observed. Moreover, in the offspring of the Slavic population another very interesting relationship was observed, which included an increase in LVMI in the II homozygotes along with the increasing sodium intake; this is consistent with the proximal sodium reabsorption values observed in the analyzed population. Multiple studies have revealed that the increase in proximal sodium reabsorption is related to the sodium sensitivity of hypertension (Citation10–12) and its target organ complications (Citation13,Citation14). In our study group, we also demonstrated (Citation24) that increased proximal sodium reabsorption may be related to the development of systolic LV dysfunction by its influence on blood pressure and arterial stiffness parameters.
Our results concerning the CYP11B2 C-344T polymorphism also indicate the relationship between this polymorphism and sodium sensitivity of hypertension and cardiovascular remodeling. Most of the data available in the literature support the correlation between the T allele and increased serum (Citation25) and urinary (Citation26) aldosterone levels, and plasma renin activity was found to be 64.3% higher in T allele carriers than in C allele carriers (Citation27).
The data obtained in our study group, consisting of patients with a long-standing history of hypertension and high dietary sodium intake, indicate the protective effect of the C allele on arterial wall remodeling. We demonstrated that this effect results from the interaction between the CYP11B2 C-344T polymorphism and proximal sodium reabsorption. In the subgroup of patients with FELi below the median value, we observed significantly higher values of arterial stiffness parameters in the TT homozygotes compared with the C allele carriers, which were not observed in the subgroup with FELi below the median value. This interaction was also observed with respect to distal sodium reabsorption, but not for 24 h urinary sodium excretion. The results obtained in the subgroup with FELi below the median value seem to be coherent and consistent with our current knowledge, while the results obtained in the subgroup with FDRNa below the median value are unclear. Nevertheless, these results may be explained by the analyses of the correlation between FELi and FDRNa conducted in the subgroups with FELi and FDRNa below and above the median values. In the subgroup of patients with FDRNa below the median value, reflecting the subjects in whom less sodium is reabsorbed in the distal tubule because of the increased proximal sodium reabsorption, the correlation between FELi and FDRNa disappeared (r = 0.19; p = 0.16). On the other hand, in the group with FDRNa above the median value, reflecting the subjects in whom more sodium was reabsorbed in the distal tubule because of the decreased proximal sodium reabsorption, the correlation between FELi and FDRNa became stronger (r = 0.74; p < 0.0001). These results indicate that the differences with respect to arterial stiffness parameters observed in the subgroup with FDRNa below the median value may be directly related to, and secondary to the changes in FELi, and thus they were observed in the group with decreased distal sodium reabsorption and not, as might have been expected, in the group with increased distal sodium reabsorption.
The results of numerous studies evaluating the relationships between the CYP11B2 C-344T polymorphism and a predisposition to the development of hypertension and its target organ complications are inconsistent. A meta-analysis of 42 studies published by Sookoian et al. (Citation27) revealed that the CYP11B2 T allele carrier status was associated with a 17% higher risk of hypertension compared with the CC homozygotes. The same authors, in a meta-analysis of 14 studies, demonstrated a correlation between T allele carrier status and LV hypertrophy and increased LV mass (Citation28). Nevertheless, in the study cited above, Wojciechowska et al. (Citation8) analyzed the correlation between the CYP11B2 C-344T polymorphism and 24 h urinary sodium excretion with respect to peripheral and central blood pressures and parameters of arterial stiffness, and confirmed the statistically significant interaction of AIxP and AIxC with deleterious effects of the C allele in the subgroup with 24 h sodium excretion above the median value. In our study, we obtained different results and we observed no interaction between the analyzed polymorphism and 24 h urinary sodium excretion. These results may indicate that in patients with hypertension the endogenous lithium clearance may provide data that are better correlated with parameters of arterial stiffness, and thus more useful for the estimation of cardiovascular risk, than 24 h urinary sodium excretion. Further studies are necessary to confirm our results in other populations of patients with hypertension and high dietary sodium intake.
Our study should be interpreted in the context of its limitations and strengths. The main limitation relating to the enrollment of patients with long-standing hypertension to studies on renal sodium regulation lies in the fact that they are treated with antihypertensive drugs. To avoid the interference of diuretics with renal sodium excretion and reabsorption, the patients enrolled in the study were required to be receiving no long-term diuretic therapy or to have their hypertension controlled effectively enough to allow the discontinuation of diuretics at least 5 days before the enrollment. Nevertheless, other drugs, including ACE inhibitors, angiotensin receptor blockers, calcium channel blockers and beta-blockers were not discontinued, for both ethical and medical reasons. This is certainly the most important limitation of our study. This issue is particularly important in the case of ACE inhibitors and angiotensin receptor blockers, as they increase renal blood flow and decrease both total and fractional sodium reabsorption. Nevertheless, in the case of ACE inhibitors it has been observed that this effect is more pronounced in people with high activity of the RAA system, which is observed in normal subjects with low dietary sodium intake (Citation29). The second limitation was a non-randomized enrollment, while the key inclusion criterion was homogeneous age, which allowed for the selection of two groups with virtually no age difference. The third limitation was the study group of only 131 subjects, which did not allow for measurements in the respective tertiles of FELi because of the limited number of patients in these groups. We did not perform strict power calculations, which may have had some bearing on our inferences. However, a lack of power would have rather blunted the significance in all our analyses. Conversely, we show divergent effects in individuals with high compared to low FELi, which proves otherwise.
Conclusions
The unfavorable effect of the ACE I allele on the development of arterial stiffness demonstrated in the study group of patients with hypertension and high dietary sodium intake is probably related to the decreased plasticity of the RAA system caused by the presence of the I allele, and particularly by II homozygote status. This effect is manifested by the impaired decrease in serum and tissue AG II levels in response to increased sodium load, which causes increased arterial stiffness due to the direct effect of both sodium and AG II. With respect to the CYP11B2 C-344T polymorphism, the elevated plasma renin activity may be an adaptation to a lack of an adequate response of the CYP11B2 promoter bearing the T allele to the angiotensin II-mediated stimulus, and therefore the putative elevated plasma AG II makes TT homozygotes more prone to hypertension and to its subsequent complications, such as arterial stiffness. Thus, our results confirm the hypothesis that the effects of allelic variants of the ACE D/I polymorphism and the CYP11B2 C-344T polymorphism on arterial stiffness parameters are in large part dependent on the additional modulating factors, including sodium overload.
Funding
This study was funded by the National Science Centre in Poland [grant no. N402 533239].
Supplemental material available online
iblo_a_1070476_sm5072.doc
Download MS Word (402.2 KB)Declaration of interest: The authors declare that they have no competing interests.
References
- Barlassina C, Lanzani C, Manunta P, Bianchi G. Genetics of essential hypertension: from families to genes. J Am Soc Nephrol. 2002;13:155–64.
- Staessen JA, Wang JG, Bianchi G, Birkenhager WH. Essential hypertension. Lancet. 2003;361:1629–41.
- Avolio AP, Deng FQ, Li WQ, Huang ZD, Xing LF, O’Rourke MF. Effects of aging on arterial distensibility in populations with high and low prevalence of hypertension: comparison between urban and rural communities in China. Circulation. 1985;71:202–10.
- Redelinghuys M, Norton GR, Scott L, Maseko MJ, Brooksbank R, Majane OH, et al. Relationship between urinary salt excretion and pulse pressure and central aortic hemodynamics independent of steady state pressure in the general population. Hypertension. 2010;56:584–90.
- Burnier M, Phan O, Wang Q. High salt intake: a cause of blood pressure-independent left ventricular hypertrophy? Nephrol Dial Transplant. 2007;22:2426–9.
- Langenfeld MR, Schobel H, Veelken R, Weihprecht H, Schmieder RE. Impact of dietary sodium intake on left ventricular diastolic filling in early essential hypertension. Eur Heart J. 1998;19:951–8.
- Giner V, Poch E, Bragulat E, Oriola J, González D, Coca A, et al. Renin–angiotensin system genetic polymorphisms and salt sensitivity in essential hypertension. Hypertension. 2000;35:512–7.
- Wojciechowska W, Staessen JA, Stolarz K, Nawrot T, Filipovský J, Tichá M, et al. European Project on Genes in Hypertension (EPOGH) Investigators. Association of peripheral and central arterial wave reflections with the CYP11B2-344C allele and sodium excretion. J Hypertens. 2004;22:2311–9.
- Kuznetsova T, Staessen JA, Stolarz K, Ryabikov A, Tikhonoff V, Olszanecka A, et al. European Project On Genes in Hypertension (EPOGH) Investigators. Relationship between left ventricular mass and the ACE D/I polymorphism varies according to sodium intake. J Hypertens. 2004;22:287–95.
- Burnier M, Biollaz J, Magnin JL, Bidlingmeyer M, Brunner HR. Renal sodium handling in patients with untreated hypertension and white coat hypertension. Hypertension. 1994;3:496–502.
- Chiolero A, Maillard M, Nussberger J, Brunner HR, Burnier M. Proximal sodium reabsorption: an independent determinant of blood pressure response to salt. Hypertension. 2000;36:631–7.
- Zou J, Li Y, Yan CH, Wei FF, Zhang L, Wang JG. Blood pressure in relation to interactions between sodium dietary intake and renal handling. Hypertension. 2013;62:719–25.
- Seidlerová J, Staessen JA, Maillard M, Nawrot T, Zhang H, Bochud M, et al. Association between arterial properties and renal sodium handling in a general population. Hypertension. 2006;48:609–15.
- Jin Y, Kuznetsova T, Maillard M, Richart T, Thijs L, Bochud M, et al. Independent relations of left ventricular structure with the 24-hour urinary excretion of sodium and aldosterone. Hypertension. 2009;54:489–95.
- Christensen S. Furosemide effect during volume expansion: evidence against lithium transport in the loop. Kidney Int Suppl. 1990;28:S45–51.
- Staessen JA, Kuznetsova T, Zhang H, Maillard M, Bochud M, Hasenkamp S, et al. Blood pressure and renal sodium handling in relation to genetic variation in the DRD1 promoter and GRK4. Hypertension. 2008;51:1643–50.
- Lindpaintner K, Pfeffer MA, Kreutz R, Stampfer MJ, Grodstein F, LaMotte F, et al. A prospective evaluation of an angiotensin-converting-enzyme gene polymorphism and the risk of ischemic heart disease. N Engl J Med. 1995;332:706–11.
- SAS Institute. The GENMOD procedure. SAS online doc version 7.1: SAS/STAT. Cary, NC: SAS Institute; 2000. p 1311–411.
- Tiret L, Rigat B, Visvikis S, Breda C, Corvol P, Cambien F, et al. Evidence, from combined segregation and linkage analysis, that a variant of the angiotensin I-converting enzyme (ACE) gene controls plasma ACE levels. Am J Hum Genet. 1992;51:197–205.
- Ueda S, Elliott HL, Morton JJ, Connell JM. Enhanced pressor response to angiotensin I in normotensive men with the deletion genotype (DD) for angiotensin-converting enzyme. Hypertension. 1995;25:1266–9.
- Morishita R, Gibbons GH, Ellison KE, Lee W, Zhang L, Yu H, et al. Evidence for direct local effect of angiotensin in vascular hypertrophy. In vivo gene transfer of angiotensin converting enzyme. J Clin Invest. 1994;94:978–84.
- Hiraga H, Oshima T, Watanabe M, Ishida M, Ishida T, Shingu T, et al. Angiotensin I-converting enzyme gene polymorphism and salt sensitivity in essential hypertension. Hypertension. 1996;27:569–72.
- Cwynar M, Wojciechowska W, Stolarz K, Wizner B, Kawecka-Jaszcz K, Staessen JA, et al. Influence of selected genetic polymorphisms of angiotensinogen, angiotensin- converting enzyme and type-1 angiotensin II receptor on arterial pressure and large artery stiffness parameters–depending on sodium intake. Arterial Hypertension. 2006;2:99–110. (In Polish.)
- Cwynar M, Gasowski J, Stompor T, Barton H, Wizner B, Dubiel M, et al. Blood pressure and arterial stiffness in patients with high sodium intake in relation to sodium handling and left ventricular diastolic dysfunction status. J Hum Hypertens. 2015;Jan 29. doi: 10.1038/jhh.2015.1. [Epub ahead of print]
- Paillard F, Chansel D, Brand E, Benetos A, Thomas F, Czekalski S, et al. Genotype–phenotype relationships for the renin–angiotensin–aldosterone system in a normal population. Hypertension. 1999;34:423–9.
- Davies E, Holloway CD, Ingram MC, Inglis GC, Friel EC, Morrison C, et al. Aldosterone excretion rate and blood pressure in essential hypertension are related to polymorphic differences in the aldosterone synthase gene CYP11B2. Hypertension. 1999;33:703–7.
- Sookoian S, Gianotti TF, González CD, Pirola CJ. Association of the C-344T aldosterone synthase gene variant with essential hypertension: a meta-analysis. J Hypertens. 2007;25: 5–13.
- Sookoian S, Gianotti TF, Pirola CJ. Role of the C-344T aldosterone synthase gene variant in left ventricular mass and left ventricular structure-related phenotypes. Heart. 2008;94: 903–10.
- Hollenberg NK, Meggs LG, Williams GH, Katz J, Garnic JD, Harrington DP. Sodium intake and renal responses to captopril in normal man and in essential hypertension. Kidney Int. 1981;20:240–5.