Abstract
Prehypertension is associated with increased cardiovascular events. While the “tracking phenomenon” is an important longitudinal characteristic of blood pressure (BP), changes in muscle sympathetic nerve activity (MSNA) over time remain unclear. This study tested the hypothesis that MSNA tracking contributes to BP trends in prehypertension. BP and MSNA were assessed in 13 prehypertensive males at rest, during hand grip and mental stressors at baseline and after 8 years. Baseline office BP averaged 127 ± 2/81 ± 2 mmHg and MSNA 24 ± 4 bursts/min. BP increased by 7 ± 2/5 ± 2 mmHg (P < 0.01) and MSNA by 11 ± 2 bursts/min (P < 0.001) at follow-up. SBP and DBP were interrelated at baseline (r = 0.65, P = 0.02) and at follow-up (r = 0.78, P = 0.002). MSNA tracking (r = 0.82; P < 0.001) was similar to BP. MSNA was strongly related to DBP at baseline (r = 0.73; P < 0.01) and follow-up (r = 0.64; P = 0.01), more so than SBP. BMI increased (P < 0.001) at follow-up but was unrelated to BP or MSNA. Despite comparable pressor and cardiac increases to handgrip and mental stressors, sympathetic responses were blunted, more pronounced to isometric test (P < 0.006) at follow-up. In conclusion, the trend in MSNA corresponds with BP changes over time suggesting that tonic sympathetic activation may contribute to time-related increase in resting BP and the development of sustained hypertension in prehypertension.
Introduction
Prehypertension is present in 25–50% of the adult healthy population globally and has been shown to progress to hypertension over time.[Citation1] The available evidence indicates that the stepwise increase in blood pressure (BP) from a threshold of 115/75 mmHg is strongly and directly related to cardiovascular (CV) mortality, mostly death from stroke and myocardial infarction.[Citation2,Citation3] The excess risk is also evident in subjects with prehypertension and increases through middle and old age.[Citation2–4] Subjects with prehypertension commonly display associated cardiovascular risk factors and target organ damage,[Citation5–8] with BP levels being a strong predictor of cardiovascular events.[Citation2,Citation4,Citation8] While the transition from prehypertension to hypertension [Citation9–11] and the strong association with poor cardiovascular outcomes are well recognized, present guidelines have limited the recommendations solely to lifestyle interventions including weight loss, dietary modifications with salt and calorie restriction, and an increase in physical activity in the prehypertensive state.[Citation12]
The pathophysiology of prehypertension is not completely understood. Stimulation of the renin–angiotensin system (RAS) and activation of the sympathetic nervous system appear to be predominantly implicated in the development of prehypertension. Increased plasma noradrenaline (norepinephrine) and renin levels have been documented in this cohort.[Citation13,Citation14] Therapy with candesartan reduced the risk of incident hypertension in prehypertensive in middle-aged subjects,[Citation15] suggesting that drugs acting on the RAS may attenuate neurohumoral activation in uncomplicated hypertension.[Citation16] While no clear-cut relationship has been documented between resting baseline MSNA and BP levels,[Citation17,Citation18] the contribution of increased noradrenaline and muscle sympathetic nerve activity (MSNA) to the development of established hypertension [Citation19–21] and its further augmentation in high-risk patients with resistant hypertension [Citation22] clearly exist. Resting single-unit and multi-unit MSNA is elevated in high–normal BP compared to optimal BP,[Citation23,Citation24] even in the presence of similar body weight.[Citation25] However, it is not known whether further increases in BP over the long term are associated with parallel increases in MSNA in subjects with prehypertension. Likewise, the long-term assessment of MSNA in normotensive subjects (office and ambulatory BP <140/90 mmHg) has not yet been elucidated. The underlying mechanisms linking excess cardiovascular mortality to BP levels in low-risk subjects with prehypertension are unknown. We therefore investigated whether the longitudinal changes in resting BP and MSNA are directly interrelated in prehypertension. We also examined changes in heart-rate variability (HRV), arterial stiffness, sympathetic and haemodynamic responses to laboratory stressors from baseline to follow-up in this cohort.
Methods
Subjects
The study was approved by the Institutional Ethics Committee, and written informed consent was obtained from all participants. In 2002, we recruited a total of 30 untreated healthy men, of whom only 13 subjects fulfilled the inclusion criteria for the BP level for study entry based on the classification from the Seventh Report of the Joint National Committee on Prevention, Detection, Evaluation, and Treatment of High Blood Pressure.[Citation26] Final assessment was performed in 13 non-smoking male subjects with prehypertension, which was defined as office-seated systolic blood pressure (SBP) of 120–139 mmHg and diastolic blood pressure (DBP) of 80–89 mmHg. None of the subjects was taking any medication or had a history of chronic disease before study enrolment.
Study protocol
All subjects were asked to refrain from alcoholic beverages for at least 48 h prior to study enrolment and were comprehensively examined in a quiet room and in a comfortable position. All measurements were obtained at baseline and again following an 8 year period. BP was measured as described below, followed by fasting biochemistry assessments. At the second visit when office BP measurement was performed, all participants underwent 24 h ambulatory blood pressure monitoring (ABPM). Following ABPM, all subjects underwent recordings of MSNA followed by pulse-wave velocity (PWV) measurement. MSNA and PWV were performed in the supine position, with all participants asked to empty their bladder before the study to minimize the possible effects of bladder distension on the test results.
Office-seated and ambulatory blood pressure
Office-seated BP was measured in the morning in both arms after a 5 min rest and was calculated as an average of three consecutive oscillometric measurements within a 2 min interval using an automated Omron 705 IT device (Omron Europe, Wegalaan, The Netherlands). The final assessment of BP level consisted of two consecutive weekly clinic visits during which BP was obtained, and therefore an average of six measurements was considered for inclusion. The arm with higher BP readings at baseline was used for the final assessment at the 8 year follow-up.
All participants underwent 24 h ABPM and heart-rate (HR) monitoring on the non-dominant arm using Spacelabs 90207 at baseline and 90217 recorders at follow-up (Spacelabs Healthcare, Issaquah, WA, USA), as described previously.[Citation27]
Muscle sympathetic nerve activity
HR was recorded concurrently from surface electrodes using a 12-lead switch box system (Dual Bio Amp, ADInstruments, Oxford, UK). BP was measured continuously by the Finometer Medical System (FMS, Amsterdam, the Netherlands). Respiration was measured by a respiratory belt transducer (ADInstruments, Oxford, UK). Sympathetic nerve activity to the muscle vascular bed was recorded continuously by obtaining multi-unit recordings of efferent postganglionic sympathetic nerve activity using microneurography (662C-3 Nerve Traffic Analysis System; Bioengineering of Iowa University, USA), as described previously.[Citation25,Citation28]
Pulse-wave velocity
Carotid–femoral PWV was measured using the Complior device (Alam Medical, Vincennes, France) [Citation29] at baseline. The SphygmoCor device (AtCor Medical, Sydney, Australia) was used at follow-up, since it had become available on the market.
Serum biochemistry
Pathology results were obtained from all subjects, who were assessed by the same biochemistry laboratory at Medical University of Gdansk at baseline and after 8 years. The methods used for the blood test measurements have not changed over the course of the study.
Protocol and procedures
Routine blood tests were performed in the fasting state following office BP measurements. MSNA and PWV were performed after a standard light breakfast. Subjects were studied in the supine position. HR, BP, respiration and MSNA were measured at rest and during physical and mental stressors (sustained handgrip and mental arithmetic). After 15 min of rest, baseline measurements were obtained over 15 min. Handgrip and mental stress tests were then conducted with a 15 min interval between each stressor. The isometric handgrip test was performed by asking the subject to sustain a handgrip of 30% of their maximum voluntary contraction for 3 min using a dynamometer. The mental stress test involved asking the subject to carry out serial subtractions as quickly as possible for 3 min. Changes in BP and MSNA during mental stress and handgrip tests were analysed individually. Average values obtained over a 3 min period before test initiation and during the test were used for analysis. The changes between these two time-points were expressed as a percentage. Use of this methodology and subsequent analysis is consistent with previously demonstrated studies, which is important when interpreting data and identifying the contributing mechanisms.[Citation25,Citation28] The same study protocol was performed at the 8 year follow-up.
Muscle sympathetic nerve activity analysis
Tracings of HR, BP, respiration and MSNA were recorded with the PowerLab data acquisition system (ADInstruments, Oxford, UK). The amplitude of each burst was determined and muscle sympathetic activity was calculated as burst frequency (bursts/min) and as burst incidence (bursts/100 heartbeats) and multiplied by mean burst amplitude and expressed as units/min. Changes in integrated MSNA allowed an evaluation of intrasubject changes in sympathetic traffic during the same recording session. A random code was attributed to all recordings and semi-automated analysis of MSNA “computerized detection” and confirmation by an operator was performed. The interindividual variability of MSNA recordings between two different visits to our laboratory (R2 = 0.97) was previously demonstrated.[Citation25]
Spectral analysis of heart-rate variability
HRV was analysed using the LabChart Module with automated software provided by ADInstruments (Oxford, UK), which allows for RR interval variability in analysis of the electrocardiogram. Data were analysed over a 20 min period of continuous stable HR recordings during microneurography. The following bands in the spectrum were assessed: very low-frequency (VLF), with frequency domain 0–0.04 Hz; low frequency (LF), between 0.04 and 0.15 Hz; and high frequency (HF), with 0.15–0.45 Hz ranges.
Statistical methods
Results are expressed as means ± SEM. The comparisons in parameters between the two visits were analysed using a paired t test. Associations between variables were evaluated by Pearson’s rank correlations. The sample size analysis indicated that 10 subjects would have 80% power for a paired t test to detect an increase in office SBP of 7 mmHg at the level of significance 0.05 and an estimated standard deviation (SD) of 7. This study, based on 13 patients with an SD of 7 and an increase in SBP by 7 mmHg, had 91% power. Additional analysis revealed that a study of 10 patients would have 94% power for a paired t test to detect an increase in MSNA of 11 bursts/min at the level of significance of 0.05 with an estimated SD of 8. Thirteen patients with an SD of 8 and an increase in MSNA of 11 bursts/min had 99% power. When two subjects who received antihypertensive medication were excluded from the analysis, the 11 remaining patients, with an SD of 6 and an increase in office SBP of 6 mmHg, had 85% power. These 11 subjects, with an SD of 9 and an increase in MSNA of 10 bursts/min, had 91% power. Statistical analysis was performed using SigmaStat version 3.5 (Systat Software, Point Richmond, CA, USA). A value of p < 0.05 was considered significant.
Results
The study cohort had a mean age of 39 ± 2 years, body mass index (BMI) was 27 ± 1 kg/m2, waist circumference 93 ± 3 cm and waist-to-hip ratio (WHR) 0.92 ± 0.02.
After 8 years of follow-up, office BP increased significantly for both SBP (127 ± 2 vs 134 ± 3 mmHg, p = 0.0002) and DBP (81 ± 2 vs 85 ± 2 mmHg, p = 0.01). A stronger association between baseline office SBP and SBP at follow-up (r = 0.77, p = 0.002) was observed than between baseline DBP and DBP after 8 years (r = 0.65, p = 0.02).
Resting MSNA increased by 11 ± 2 bursts/min (24 ± 4 vs 35 ± 4 bursts/min, p < 0.001) after 8 years (). Similar results were obtained at follow-up when MSNA was expressed as burst incidence (34 ± 5 vs 51 ± 5 bursts/100 heartbeats, p < 0.001) (). The tracking of MSNA from baseline to follow-up (r = 0.82, p < 0.001) was similar to that of BP.
Figure 1. Muscle sympathetic nerve activity (MSNA) in subjects with prehypertension, expressed as (a) bursts/min and (b) bursts/100 heartbeats, at baseline and after 8 years. Values are means ± SEM.
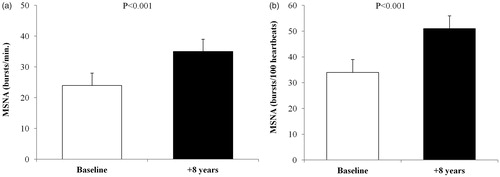
The correlations between baseline MSNA and BP were more pronounced for DBP at baseline (r = 0.73, p = 0.005) and at follow-up (r = 0.64, p = 0.01) than for SBP at baseline (r = 0.40, p = 0.17) and at follow-up (r = 0.62, p = 0.02). Forward stepwise regression analysis revealed that baseline MSNA was a predictor of SBP at follow-up (r2 = 0.38, p = 0.02). Multiple linear regression analysis adjusted for changes in SBP, DBP, BMI, waist circumference and age revealed that changes in MSNA from baseline to follow-up predicted PWV at follow-up (r2 = 0.97, standard coefficient = 3.78, p = 0.01), DBP (p = 0.04) and Δ DBP (p = 0.03) at follow-up.
Individual changes in MSNA and SBP from baseline to follow-up are shown in , respectively.
Figure 2. Individual changes in muscle sympathetic nerve activity (MSNA) in all subjects with prehypertension at baseline and after 8 years (Fig. ) and individual changes in office systolic blood pressure (SBP) at baseline and after 8 years (Fig. ). One subject (▪) developed hypertension and a myocardial infarction between baseline and follow-up. A separate subject (▴) developed hypertension and received antihypertensive treatment (beta-blocker) over the course of the study, and subsequently had a myocardial infarction two years after study completion (at 10 years of follow-up).
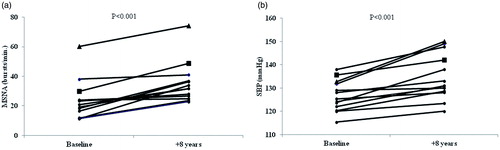
Office baseline HR remained unchanged after 8 years (77 ± 3 vs 82 ± 3 bpm, p = 0.29).
Although there were no statistical changes in 24 h BP and HR monitoring at follow-up (), the nocturnal dipping pattern observed at baseline was significantly reduced at follow-up (p < 0.05).
Table 1. Ambulatory blood pressure and heart-rate monitoring at baseline and after 8 years.
During the follow-up period, four subjects (31%) developed hypertension, including one subject from the study cohort who developed coronary artery disease with a myocardial infarction.
There were significant increases in all body composition measures, including an increase in BMI by 3 ± 0.6 kg/m2 (p < 0.001), waist circumference by 9 ± 2 cm (p < 0.001) and WHR by 0.05 ± 0.02 (p < 0.01) following an 8 year period. Changes in BMI from baseline to follow-up were not related to changes in SBP (r = 0.15, p = 0.62), DBP (r = 0.42, p = 0.15) or MSNA (r = 0.39, p = 0.19). Similarly, no direct association between an increase in waist circumference and changes in SBP (r = 0.006, p = 0.98), DBP (r = 0.18, p = 0.55) and MSNA (r = 0.30, p = 0.32) were observed. At 8 years of follow-up, BMI was related to DBP (r = 0.65, p = 0.016).
BP and HR increased significantly in response to acute handgrip and mental stress tests at baseline and after 8 years of follow-up (). There was a significant sympathetic response to acute handgrip test (p < 0.001) and mental stress (p < 0.05) at baseline. After 8 years, despite a significant increase in BP and HR during acute handgrip (p < 0.001) and mental stress (p = 0.002), there was a blunted sympathetic response to both isometric (12 ± 6%, p = 0.09) and arithmetic stress (9 ± 7%, p = 0.10) tests at follow-up ().
Table 2. Changes in systolic blood pressure (SBP), heart rate (HR) and muscle sympathetic nerve activity (MSNA) in response to handgrip and mental stress tests at baseline and after 8 years.
There were no significant changes in glucose level, lipid profile or kidney function from baseline to follow-up ().
Table 3. Changes in lipid and glucose profile at baseline and after 8 years.
At follow-up, carotid–femoral PWV was significantly related to MSNA (r = 0.64, p = 0.018), but not to SBP (r = 0.18, p = 0.56), DBP (r = 0.37, p = 0.21), HR (r = −0.11, p = 0.72) or BMI (r = 0.39, p = 0.19). Three out of 13 subjects had the cut-off value for carotid–femoral PWV > 10 m/s (between 10.3 and 11.3 m/s) at follow-up.
There was a significant reduction in VLF power of HRV from baseline to follow-up (1938.5 ± 642.5 vs 1355.0 ± 483.2 ms2, p< 0 .05), but not in LF (1168.8 ± 357.6 vs 1038.4 ± 369.1 ms2, p = 0.76), HF (944.8 ± 327.0 vs 1066.1 ± 509.3 ms2, p = 0.82) or LF/HR ratio (2.4 ± 0.6 vs 3.5 ± 1.0, p = 0.11).
Discussion
The present study assessed for the first time the longitudinal changes in BP and MSNA in subjects with prehypertension. The major finding is that the prehypertensive state is characterized by an augmentation of resting MSNA and corresponding BP changes at 8 years of follow-up. While pressor and cardiac responses to physical and arithmetic stressors were still present in subjects with prehypertension after 8 years, sympathetic responsiveness was blunted over this period. Parallel increases in body weight parameters observed over the longer term bore no direct relationship with changes in BP and MSNA. In addition, arterial stiffness reflecting target organ damage was directly related to MSNA over the course of the study.
Our findings support the concept that tonic sympathetic activation is a key contributor to BP elevation and accompanying arterial remodelling, which are likely to lead to adverse cardiovascular events commonly evident in subjects with prehypertension.
Previous studies on prehypertension were based on office rather than ambulatory BP measurements. The mechanisms underlying the selective MSNA-related increase in office but not ambulatory BP after 8 years are less clear. The only randomized controlled study in prehypertension to date which included subjects over 30 years of age (similar to our study cohort), although based on office BP measurements, clearly indicated that approximately 63% of patients with untreated prehypertension developed stage 1 hypertension after 4 years of follow-up.[Citation15] In addition, age-related differences between conventional and ambulatory BP measurements have been previously demonstrated in a cross-sectional study of a total of 1104 subjects with a less pronounced increment increase in BP with ageing, particularly in young and middle-aged subjects.[Citation30] The within-subject differences increased significantly with ageing and BMI.[Citation30] The difference in the model of ABPM recorders used (at baseline and follow-up) and antihypertensive medication required in the two subjects may to some extent influence ABPM results. We previously demonstrated that resting MSNA level is comparable between dipper and non-dipper patients with newly diagnosed untreated essential hypertension.[Citation27] In this study, we observed an increase in the night-to-day BP ratios from baseline to follow-up, suggesting the transition from the dipping pattern to the mild dipping profile. This may be of potential clinical relevance as the night-to-day ratio predicts all-cause mortality and cardiovascular events in patients with hypertension without a previous history of major cardiovascular events independently of 24 h BP.[Citation31] Whether the night-to-day ratio confers a heightened risk for cardiovascular events in prehypertension needs to be examined in future studies.
While there is evidence that sympathetic activity increases progressively with ageing when compared to resting MSNA levels across different age groups, it has been suggested that age is not a major determinant of MSNA.[Citation32] We previously demonstrated in a cohort of 216 healthy subjects that the age-mediated increase in MSNA is associated with increased BP in older subjects, predominantly in females.[Citation33] Unlike previous findings, this study is the first to demonstrate concomitant changes in BP and MSNA in the same individual over an 8 year period. While it appeared that age is unrelated to MSNA over the longer term, further studies need to investigate the impact of age on MSNA within the same subject.
Elevated HR is a common feature of hypertensive subjects and critically involved in the progression of hypertension and cardiovascular disease.[Citation34] However, there is evidence to suggest that HR is an unreliable marker of cardiac adrenergic activity or sympathetic drive.[Citation35] Previous studies, including ours, have shown that HR remained unaltered in the presence of increased MSNA in high–normal BP when compared to optimal BP [Citation24,Citation25], despite an impairment of baroreflex HR control.[Citation24] In the present study, resting HR was unchanged from baseline to follow-up despite substantial increases in BP and MSNA at follow-up, indicating the important contribution of increased sympathetic outflow to chronic BP elevation in middle-aged subjects with prehypertension. Along these lines, the magnitude of MSNA response to acute stress was not predicted by resting levels of sympathetic activation in high–normal BP, indicating that the relationship is mediated through tonic activation of the sympathetic nervous system, but not enhanced acute sympathetic responsiveness.[Citation25] The present study showed persistent sympathetic activation in prehypertension at follow-up despite comparable BP and HR increases in response to acute laboratory stressors. Furthermore, there was a blunted sympathetic responsiveness to laboratory stressors despite greater resting BP level. A possible explanation for the blunted MSNA response to laboratory stressors despite comparable BP rises may be an increase in arterial stiffness accompanying structural narrowing of the blood vessels over an 8 year period. Subsequently, in the presence of the structurally altered blood vessels, a certain level of vasoconstriction may potentially induce a further increase in vascular resistance and BP, thereby producing a higher BP with a lower degree of sympathetically mediated vasoconstriction. The blunted sympathetic responsiveness to stressors may suggest that chronic sympathetic overdrive in prehypertension further acts as a self-perpetuating mechanism contributing to adverse cardiovascular events.
In this context, an association between arterial stiffness and MSNA in prehypertension after 8 years that was independent of BP, HR, BMI, waist circumference and age is of clinical significance. While arterial stiffness is a hallmark of hypertension and increasingly recognized as an independent predictor of all-cause and cardiovascular morbidity and mortality,[Citation36] arterial stiffening also predicted the progression to established hypertension in subjects with high–normal BP.[Citation37] We previously demonstrated an independent relationship between MSNA and PWV in healthy subjects, indicating that sympathetic activation is a critical contributor to the short-term reduction of arterial wave reflection.[Citation38] More recently, we demonstrated that targeting sympathetic nerves with renal denervation resulted in rapid reduction of arterial stiffness and MSNA in patients with resistant hypertension and chronic kidney disease.[Citation39,Citation40] In view of these findings, the present data indicate that a sympathetically mediated increase in arterial stiffness is likely to be implicated in chronic BP elevation in prehypertension. Subsequently, early detection of reduced arterial compliance in individuals with prehypertension may identify subjects at high cardiovascular risk before the development of established hypertension. Whether increased arterial stiffness and sympathetic activation found in prehypertensive subjects are possible mechanisms underlying the increased risk of death from cardiovascular events needs to be addressed in larger clinical studies.
An increase in body weight over the longer term has been shown to be a critical contributor to the development of sustained hypertension in patients with prehypertension.[Citation11,Citation41] In the present study, weight gain at 8 years of follow-up was directly related only to DBP level, but not to SBP or MSNA increases. In addition, sympathetic activation seemed to be related to several components of the metabolic syndrome including abdominal obesity, dyslipidaemia and hyperglycaemia.[Citation42] More recently, a close association between the homeostasis model assessment (HOMA) index and MSNA was observed in subjects with high–normal BP.[Citation24] However, despite an increase in waist circumference and MSNA, there were no changes in glycaemic or lipid profiles in prehypertension at follow-up. Our findings corroborate Julius’s concept that the primary increase in sympathetic activation leads to the down-regulation of β-adrenergic responsiveness, causing both elevation in BP and body weight gain, with further augmentation of cardiovascular consequences.[Citation43] In fact, plasma noradrenaline levels predicted subsequent BP elevation and weight gain in lean normotensive men followed annually over 5 years,[Citation44] suggesting a cause-and-effect relationship between sympathetic activation and BP.
Another interesting observation in the present study is the reduction in the VLF component of HRV at follow-up. Previous studies have shown that the VLF power of HRV is influenced by the RAS and the prognostic significance of VLF RR-interval oscillations depends primarily on parasympathetic outflow.[Citation45] In this context, our findings could be of clinical relevance given that among the spectral measures of HRV, VLF power has been shown to be an independent predictor of arrhythmic death, all-cause mortality and cardiac death in patients with myocardial infarction,[Citation46] and cardiovascular events in heart failure patients.[Citation47] Whether reduced VLF power of HRV is associated with increased cardiovascular mortality from vascular events in prehypertension deserves further investigation.
Study limitations
We included a relatively modest number of subjects in the present study and the lack of a control group or female subjects could be viewed as limitations. However, all subjects were recruited from a larger cohort and comprehensively assessed for baseline BP level, thereby eliminating potential confounding factors including smoking, baseline body weight or physical activity. Given that the prehypertensive state is considered normotensive as per guidelines (office and ambulatory BP < 140/90 mmHg), it was not feasible to define matched controls in 2002. In contrast to secondary forms of hypertension, primary essential hypertension occurs more frequently in young males than in females, who predominantly develop hypertension in middle age. Moreover, the potential impact of female reproductive hormone status on sympathetic neural control [Citation48] or the use of contraception (i.e. drugs) over the course of the study could be considered as confounding factors. Secondly, the arterial stiffness at baseline and follow-up was assessed with different devices (Complior vs SphygmoCor). Given that the values of PWV obtained using the Complior are significantly higher than those obtained using the SphygmoCor,[Citation49] we have not provided the comparison between these two measures. Clearly, carotid–femoral PWV was significantly related to MSNA in subjects with prehypertension after 8 years, indicating the development of target organ damage over time. Likewise, the use of different Spacelabs recorders at baseline and follow-up may influence the 24 h BP results. However, irrespective of ambulatory BP levels, the prehypertensive state is associated with an increase in MSNA and arterial stiffness over time, both of which are relevant mechanisms underlying the transition from prehypertension to hypertension and acute cardiac events. Finally, we did not assess other potential mechanisms that are likely to be implicated in the prehypertensive state, including the RAS, insulin sensitivity, endothelial function and inflammatory markers, which could be further explored in this cohort.
Perspectives
This is the first study to demonstrate tracking of BP and MSNA for 8 years in middle-aged subjects who met prehypertension criteria. Prehypertension is associated with chronic sympathetic activation and blunted sympathetic responsiveness to physical and mental stressors over time, in addition to an increase in arterial stiffness and a reduction in VLF power HRV that are not accompanied by concomitant changes in glycaemic and lipid profiles. Several relevant findings derived from this study may inform and guide the application of PWV, HRV and night–day BP measurements into clinical practice, and potentially aid in identifying subjects with prehypertension at high cardiovascular risk. Randomized clinical studies with prolonged observation are needed to elucidate whether this prehypertensive subgroup will benefit from anti-hypertensive therapy and thereby contribute to improved outcomes in this condition.
Declaration of interest
No potential conflict of interest was reported by the authors. The authors take responsibility for all aspects of the reliability and freedom from bias of the data presented and their discussed interpretation.
This study was supported by European Regional Development Fund, project FNUSA-ICRC [no. CZ.1.05/1.1.00/02.0123] and by project ICRC-ERA-Human Bridge [no. 316345] funded by the European Commission.
References
- Egan BM, Stevens-Fabry S. Prehypertension – prevalence, health risks, and management strategies. Nat Rev Cardiol. 2015;12:289–300.
- Vasan RS, Larson MG, Leip EP, et al. Impact of high–normal blood pressure on the risk of cardiovascular disease. N Engl J Med. 2001;345:1291–1297.
- Lewington S, Clarke R, Qizilbash N, et al. Age-specific relevance of usual blood pressure to vascular mortality: a meta-analysis of individual data for one million adults in 61 prospective studies. Lancet. 2002;360:1903–1913.
- Liszka HA, Mainous AG III, King DE, et al. Prehypertension and cardiovascular morbidity. Ann Fam Med. 2005;3:294–299.
- Julius S, Jamerson K, Mejia A, et al. The association of borderline hypertension with target organ changes and higher coronary risk. Tecumseh Blood Pressure study. JAMA. 1990;264:354–358.
- Greenlund KJ, Croft JB, Mensah GA. Prevalence of heart disease and stroke risk factors in persons with prehypertension in the United States, 1999–2000. Arch Intern Med. 2004;164:2113–2118.
- Nesbitt SD, Julius S, Leonard D, et al. Is low-risk hypertension fact or fiction? Cardiovascular risk profile in the TROPHY study. Am J Hypertens. 2005;18:980–985.
- Qureshi AI, Suri MF, Kirmani JF, et al. Is prehypertension a risk factor for cardiovascular diseases? Stroke. 2005;36:1859–1863.
- Levy R, Hillman C, Stroud W, White P. Transient hypertension: its significance in terms of later development of sustained hypertension and cardiovascular–renal diseases. JAMA. 1944;126:829–833.
- Leitschuh M, Cupples LA, Kannel W, et al. High–normal blood pressure progression to hypertension in the Framingham Heart Study. Hypertension. 1991;17:22–27.
- Vasan RS, Larson MG, Leip EP, et al. Assessment of frequency of progression to hypertension in non-hypertensive participants in the Framingham Heart Study: a cohort study. Lancet. 2001;358:1682–1686.
- Mancia G, Fagard R, Narkiewicz K, et al. 2013 ESH/ESC Guidelines for the management of arterial hypertension: the Task Force for the management of arterial hypertension of the European Society of Hypertension (ESH) and of the European Society of Cardiology (ESC). J Hypertens. 2013;31:1281–1357.
- Esler M, Julius S, Zweifler A, et al. Mild high-renin essential hypertension. Neurogenic human hypertension? N Engl J Med. 1977;296:405–411.
- Julius S, Krause L, Schork NJ, et al. Hyperkinetic borderline hypertension in Tecumseh, Michigan. J Hypertens. 1991;9:77–84.
- Julius S, Nesbitt SD, Egan BM, et al. Feasibility of treating prehypertension with an angiotensin-receptor blocker. N Engl J Med. 2006;354:1685–1697.
- Grassi G, Trevano FQ, Seravalle G, et al. Baroreflex function in hypertension: consequences for antihypertensive therapy. Prog Cardiovasc Dis. 2006;48:407–415.
- Wallin BG, Sundlof G. A quantitative study of muscle nerve sympathetic activity in resting normotensive and hypertensive subjects. Hypertension. 1979;1:67–77.
- Somers VK, Mark AL, Abboud FM. Potentiation of sympathetic nerve responses to hypoxia in borderline hypertensive subjects. Hypertension. 1988;11:608–612.
- Floras JS, Hara K. Sympathoneural and haemodynamic characteristics of young subjects with mild essential hypertension. J Hypertens. 1993;11:647–655.
- Esler M, Jennings G, Biviano B, et al. Mechanism of elevated plasma noradrenaline in the course of essential hypertension. J Cardiovasc Pharmacol. 1986;8 Suppl 5:S39–S43.
- Schlaich MP, Lambert E, Kaye DM, et al. Sympathetic augmentation in hypertension: role of nerve firing, norepinephrine reuptake, and angiotensin neuromodulation. Hypertension. 2004;43:169–175.
- Hering D, Lambert EA, Marusic P, et al. Substantial reduction in single sympathetic nerve firing after renal denervation in patients with resistant hypertension. Hypertension. 2013;61:457–464.
- Greenwood JP, Stoker JB, Mary DA. Single-unit sympathetic discharge: quantitative assessment in human hypertensive disease. Circulation. 1999;100:1305–1310.
- Seravalle G, Lonati L, Buzzi S, et al. Sympathetic nerve traffic and baroreflex function in optimal, normal, and high–normal blood pressure states. J Hypertens. 2015;33:1411–1417.
- Hering D, Kara T, Kucharska W, et al. High–normal blood pressure is associated with increased resting sympathetic activity but normal responses to stress tests. Blood Press. 2013;22:183–187.
- Chobanian AV, Bakris GL, Black HR, et al. Seventh report of the Joint National Committee on prevention, detection, evaluation, and treatment of high blood pressure. Hypertension. 2003;42:1206–1252.
- Hering D, Kucharska W, Kara T, et al. Resting sympathetic outflow does not predict the morning blood pressure surge in hypertension. J Hypertens. 2011;29:2381–2386.
- Hering D, Kucharska W, Kara T, et al. Effects of acute and long-term slow breathing exercise on muscle sympathetic nerve activity in untreated male patients with hypertension. J Hypertens. 2013;31:739–746.
- Asmar R, Benetos A, Topouchian J, et al. Assessment of arterial distensibility by automatic pulse wave velocity measurement. Validation and clinical application studies. Hypertension. 1995;26:485–490.
- Staessen J, O’Brien E, Atkins N, et al. The increase in blood pressure with age and body mass index is overestimated by conventional sphygmomanometry. Am J Epidemiol. 1992;136:450–459.
- Fagard RH, Thijs L, Staessen JA, et al. Night-day blood pressure ratio and dipping pattern as predictors of death and cardiovascular events in hypertension. J Hum Hypertens. 2009;23:645–653.
- Sundlof G, Wallin BG. Human muscle nerve sympathetic activity at rest. Relationship to blood pressure and age. J Physiol. 1978;274:621–637.
- Narkiewicz K, Phillips BG, Kato M, et al. Gender-selective interaction between aging, blood pressure, and sympathetic nerve activity. Hypertension. 2005;45:522–525.
- Palatini P. Role of elevated heart rate in the development of cardiovascular disease in hypertension. Hypertension. 2011;58:745–750.
- Grassi G, Mark A, Esler M. The sympathetic nervous system alterations in human hypertension. Circ Res. 2015;116:976–990.
- Laurent S, Cockcroft J, Van Bortel L, et al. Expert consensus document on arterial stiffness: methodological issues and clinical applications. Eur Heart J. 2006;27:2588–2605.
- Yambe M, Tomiyama H, Yamada J, et al. Arterial stiffness and progression to hypertension in Japanese male subjects with high normal blood pressure. J Hypertens. 2007;25:87–93.
- Swierblewska E, Hering D, Kara T, et al. An independent relationship between muscle sympathetic nerve activity and pulse wave velocity in normal humans. J Hypertens. 2010;28:979–984.
- Hering D, Mahfoud F, Walton AS, et al. Renal denervation in moderate to severe CKD. J Am Soc Nephrol. 2012;23:1250–1257.
- Hering D, Lambert EA, Marusic P, et al. Renal nerve ablation reduces augmentation index in patients with resistant hypertension. J Hypertens. 2013;31:1893–1900.
- Markus MR, Stritzke J, Siewert U, et al. Variation in body composition determines long-term blood pressure changes in pre-hypertension: the MONICA/KORA (Monitoring Trends and Determinants on Cardiovascular Diseases/Cooperative Research in the Region of Augsburg) cohort study. J Am Coll Cardiol. 2010;56:65–76.
- Grassi G, Dell’Oro R, Quarti-Trevano F, et al. Neuroadrenergic and reflex abnormalities in patients with metabolic syndrome. Diabetologia. 2005;48:1359–1365.
- Julius S, Valentini M, Palatini P. Overweight and hypertension: a 2-way street? Hypertension. 2000;35:807–813.
- Masuo K, Kawaguchi H, Mikami H, et al. Serum uric acid and plasma norepinephrine concentrations predict subsequent weight gain and blood pressure elevation. Hypertension. 2003;42:474–480.
- Taylor JA, Carr DL, Myers CW, Eckberg DL. Mechanisms underlying very-low-frequency RR-interval oscillations in humans. Circulation. 1998;98:547–555.
- Bigger JT Jr, Fleiss JL, Steinman RC, et al. Frequency domain measures of heart period variability and mortality after myocardial infarction. Circulation. 1992;85:164–171.
- Hadase M, Azuma A, Zen K, et al. Very low frequency power of heart rate variability is a powerful predictor of clinical prognosis in patients with congestive heart failure. Circ J. 2004;68:343–347.
- Charkoudian N. Influences of female reproductive hormones on sympathetic control of the circulation in humans. Clin Auton Res. 2001;11:295–301.
- Rajzer MW, Wojciechowska W, Klocek M, et al. Comparison of aortic pulse wave velocity measured by three techniques: Complior, SphygmoCor and Arteriograph. J Hypertens. 2008;26:2001–2007.