It is with great enthusiasm that we present to the scientific community our annual thematic issue, entitled: “Regulatory Myeloid Cells in Neoplasia.” As Guest Editor, it has been a true privilege to oversee the assembly of 11 comprehensive, dynamic and insightful chapters by highly regarded investigators in this explosive field of tumor immunology and immunotherapy. Special thanks to Dr. Kate Rittenhouse-Olson, Editor-in-Chief, who offered me the opportunity and honor to serve as Guest Editor, to Dr. Paul Wallace who provided critical expertise throughout this project and, most importantly, to all authors that made this issue possible. Altogether, the theme focuses on immune suppressive myeloid cells, namely myeloid-derived suppressor cells (MDSCs) and tolerogenic dendritic cells (DCs). Both myeloid populations are strongly considered influential players of tumor-induced immune suppression during host immune surveillance and immunotherapy (Gabrilovich et al., Citation2012).
It has become clear that a major barrier to effective immunotherapy is not the inability to create powerful immunization strategies, but rather the ability of the neoplastic process to disrupt fundamental mechanisms crucial for productive immune activation in response to such strategies. This is achieved largely through a tumor-mediated myeloid differentiation block, resulting in the accumulation of dysfunctional antigen-presenting cells (APCs) (Cheng et al., Citation2008; Sinha et al., Citation2008). Ordinarily as APCs, DCs and macrophages supply all the necessary signals, contact-dependent and contact-independent (via cytokines), to drive productive immune interactions.
However, tumor-mediated secretion of diverse soluble factors, broadly characterized as tumor-derived factors (TDFs), have the potential to compromise normal myelopoiesis and the formation of competent APCs. The types of TDFs, such as VEGF, IL-10, IL-6, PGE2 and gangliosides (GD2, GM3) are potent facilitators of myeloid dysfunction which, in turn, antagonize adaptive immunity. Thus, the neoplastic process has essentially “hijacked” bone marrow-derived myeloid progenitors to serve the needs of the tumor, rather than the needs of the host.
Each chapter of this issue tackles specific angles of MDSC- or DC-tumor biology. Although some overlap in background information is unavoidable in projects such as these, it does serve an important purpose and places each author’s work in a unique context. This body-of-work covers an array of basic, translational and clinical principles within this field of myeloid-tumor biology. The chapters on MDSC biology encompass several major themes, including: broad overviews and perspectives, in-depth reviews of MDSC-mediated mechanisms of tumor progression or therapeutic interventions, and emerging concepts to the field. The final two chapters cover equally provocative scientific and applied principles in DC-tumor biology.
Mechanisms of tumor-induced MDSC development. Aberrant myelopoiesis from bone marrow progenitors is initiated by circulating tumor-derived factors (TDFs), many of which function through STAT3 or STAT5 activation. Activated STAT3 or STAT5 then translocates to the nucleus where it binds to specific elements of myelopoietic target genes that in turn alter normal myeloid cell differentiation. Several STAT3 or STAT5 target genes have been previously described (reviewed in (Gabrilovich et al., Citation2012). A few examples of relevant TDFs, as well as mechanisms of granulocytic or monocytic MDSC-mediated tumor progression are shown.
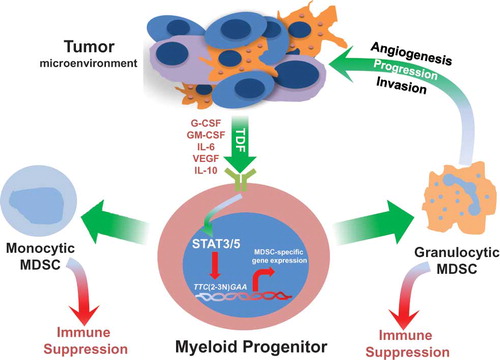
Because all articles cover varying amounts of these fundamental elements, I would like to highlight major conceptual and scientific advances unique to each laboratory, which are summarized in their respective contribution.
The article by Qu et al. (“Negative Regulation of Myeloid-Derived Suppressor Cells in Cancer”) (Qu et al., Citation2012) summarizes key findings that MDSC can facilitate tumor angiogenesis by increasing the bioavailability of VEGF through a MMP9-dependent mechanism. This work was one of the first to show that MDSCs are multifunctional and can mediate tumor progression beyond the level of immune suppression. The article by Haile et al. (“Immune Suppression: The Hallmark of Myeloid Derived Suppressor Cells”) (Haile et al., Citation2012) summarizes their novel finding that MDSC subsets can be distinguished phenotypically on the basis of differential expression of the CD49d cell surface marker. Consequently, this work illuminated a more refined way to delineate and track monocytic from granulocytic subsets. The article by Monu and Frey (“Myeloid-Derived Suppressor Cells and Anti-Tumor T cells: a Complex Relationship”) (Monu and Frey, Citation2012) supplies new and provocative preclinical data that MDSC-mediated immunosuppression essentially takes place at the induction phase, rather than the effector phase of the adaptive immune response. Although more work is needed, this article offers new insights into the stages of which MDSC-mediated immunosuppression is likely to be most relevant during neoplasia.
The article by Raber et al. (“Metabolism of L-Arginine by Myeloid-Derived Suppressor Cells in Cancer: Mechanisms of T Cell Suppression and Therapeutic Perspectives”) (Raber et al., 2012) discusses in detail how arginine depletion, a major mechanism of MDSC-mediated immunosuppression, compromises T cell activation and promotes T cell tolerance. Moreover, studies by this laboratory were the first to demonstrate that MDSCs in cancer patients employ an arginase I-based mechanism of immunosuppression.
The article by Obermajer et al. (“PGE2-Driven Induction and Maintenance of Cancer-Associated Myeloid-Derived Suppressor Cells”) (Obermajer et al., Citation2012) summarizes new insights into how tumor-associated PGE2 drives MDSC development and recruitment to the tumor microenvironment. These findings are of particular importance, since major efforts within the oncology community have focused on the clinical value of non-steroidal anti-inflammatory drugs (NSAIDs) in cancer prevention and therapy. Indeed, this work also provides an additional explanation as to how NSAIDs carry out their antitumor activities.
The article by Kohanbash et al. (“Myeloid-Derived Suppressor Cells (MDSCs) in Gliomas and Glioma-Development”) (Kohanbash and Okada, Citation2012) summarizes key insights into the role of MDSCs in driving glioma development. Moreover, their work illuminates a novel role for type 1 interferon-based interventions to reduce MDSC accumulation in glioma settings, with potentially broader implications in other cancer types. The article by Cohen et al. (“Myeloid-Derived Suppressor Cells Adhere to Physiologic STAT3- vs STAT5-Dependent Hematopoietic Programming, Establishing Diverse Tumor-Mediated Mechanisms of Immunologic Escape”) (Cohen et al., Citation2012) summarizes key discoveries reflecting the therapeutic potential of tyrosine kinase inhibitors (TKIs), namely sunitinib, to compromise MDSC accumulation. Interestingly, in cases of sunitinib resistance, Cohen and colleagues identified a novel TDF (e.g., GM-CSF)-driven STAT5-dependent mechanism undermining TKI potential. The identification of this mechanism offers new insights into the design of therapeutic interventions that overcome TKI resistance.
The article by Manjili’s group (“Phenotypic Plasticity of MDSC in Cancers”) (Manjili, Citation2012) introduces new concepts in MDSC biology, namely the concept of ‘plasticity’ which has been often applied to macrophage functionality (Sica and Mantovani, Citation2012). The notion of ‘plasticity’ implies that tumor-promoting MDSCs can be reprogrammed for therapeutic purposes. Here, Manjili’s group posits that MDSC plasticity can be achieved through novel NK- or NKT cell-dependent mechanisms.
The article by Solito et al. (“Highlights on Molecular Mechanisms of MDSC-Mediated Immune Suppression: Paving the Way for New Working Hypotheses”) (Solito et al., Citation2012) summarizes key findings reflecting the identification of the immature myeloid cell type most responsible for MDSC activity. In a human system, Solito and colleagues elegantly identified ‘promyelocytic-like’ cells within the bone marrow as a relevant component of suppressive activity. It is interesting to point out that promyelocytes represent an immature population of the granulocytic lineage, whereas promonocytes represent the counterpart population of the monocytic lineage (Rosenbauer and Tenen, Citation2007). Whether the promonocyte has suppressive activity remains unclear. Nonetheless, the discovery of a specific myeloid subset(s) will advance our understanding of MDSC biology and, furthermore, refine ways to track or target MDSCs in cancer therapy.
Lastly, the articles by Harden and Egilmez (“Indoleamine 2,3-dioxygenase and Dendritic Cell Tolerogenicity”) (Harden and Egilmez, Citation2012) and Johnson and Munn (“Host Indoleamine 2,3-Dioxygenase: Contribution to Systemic Acquired Tumor Tolerance”) (Johnson and Munn, Citation2012) focus on how DCs mediate immune suppression and T cell tolerance under neoplastic conditions, with emphasis on the enzyme indoleamine 2,3-dioxygenase (IDO). IDO is known to catabolize the essential amino acid tryptophan, which in T cell biology, is critical for immune activation.
The mechanism by which IDO promotes immune suppression is conceptually akin to the role of arginase I in MDSC biology, which catabolizes the amino acid arginine. Harden and Egilmez discovered a novel role for IDO production by DCs reflecting the ability of IDO to impede cytokine-based tumor immunotherapy in animal models. This work provides a fundamental mechanistic basis for the conundrum of why such types of immunotherapies start off quite effective, but then lose their potency. The article by Johnson and Munn provides in-depth coverage of IDO in cancer as well as in other pathologies. In fact, Munn and colleagues were the first to demonstrate that subsets of DCs represent a prominent source of IDO (Munn et al., Citation2002). This seminal discovery laid the framework for the development of IDO inhibitors in cancer therapy.
The work from our laboratory focuses on how MDSCs develop in the first place (Stewart et al., Citation2009a; Stewart et al., Citation2009b), and recently we identified a previously undescribed role of tumor-derived G-CSF in granulocytic MDSC development (Waight et al., Citation2011). We observed abundant amounts of G-CSF in vivo, which correlated with robust granulocytic MDSC responses in multiple mammary tumor models. Using loss- and gain-of-function approaches we demonstrated that: 1) abrogating tumor-derived G-CSF production significantly attenuated granulocytic MDSC generation and neoplastic growth; 2) enforced expression of the G-CSF gene in G-CSF-negative tumors significantly enhanced granulocytic MDSC generation and neoplastic growth; and 3) treatment of non-tumor-bearing mice with recombinant G-CSF protein led to the formation granulocytic-like MDSCs remarkably similar to those induced under tumor-bearing conditions. Depleting Gr-1+ cells in mice bearing G-CSF-producing, but not non-G-CSF-producing tumors facilitated a significant reduction in neoplastic growth, supporting a causal link between G-CSF-induced granulocytic MDSC accumulation and tumor progression.
Collectively, we demonstrated that tumor-derived G-CSF enhances tumor growth through granulocytic MDSC-dependent mechanisms. These data imply that high concentrations of G-CSF acting in a systemic and chronic manner can impair normal myeloid cell development or differentiation, leading to the accumulation of granulocytic MDSC. Current MDSC-based pharmacologic approaches include vitamin D3, retinoic acid (ATRA), KIT-specific antibody, VEGF blockade, sunitinib and gemcitabine (reviewed in (Gabrilovich et al., Citation2012). Thus, therapeutic targeting of TDFs that initiate granulocytic MDSC development may offer additional ways to abrogate MDSC-mediated mechanisms of tumor growth, thereby enhancing immunotherapy efficacy.
Although much knowledge in the field has been gained, more questions remain, a number of which are elegantly raised and deliberated throughout this issue. I would like to take this opportunity to re-emphasize a few of them:
1. | Because MDSCs comprise multiple myeloid subsets, reflecting monocytic and granulocytic lineages, it becomes very important to determine which subset or subsets are important in a given pathologic setting. Therefore, it would seem appropriate to target the relevant MDSC subset, since the rest of the cell mixture may be irrelevant to therapeutic outcome. Furthermore, unintentional targeting of uninvolved myeloid populations may have unwanted consequences. In that regard, it may be reasonable to determine whether MDSC subset(s) express specific cell surface antigens. The identification of MDSC-specific antigens may then be exploited as potential therapeutic targets for novel vaccine strategies, conceptually in much the same manner as with tumor-associated macrophages (Luo et al., Citation2006) or cancer-associated fibroblasts (Kraman et al., Citation2010). | ||||
2. | Little is known about the mechanisms that govern MDSC subset trafficking (Sawanobori et al., Citation2008), either to secondary lymphoid tissues or the tumor microenvironment. Are they fundamentally distinct between monocytic and granulocytic MDSC subsets? | ||||
3. | In contrast to solid tumor biology, much less is known regarding the role and relevance of MDSC subsets in the pathogenesis or progression of hematologic malignancies (Tadmor et al., Citation2011). It would seem reasonable that exploration of this understudied cancer subgroup may lead to important advances, as with solid tumor systems. | ||||
4. | Considerable interest is dedicated to the possibility that changes in peripheral (systemic) MDSC subset frequencies can serve as part of a biomarker signature of response to immunotherapy or disease relapse. If so, this raises important questions; for example, do such changes in MDSC frequencies effectively precede changes in disease status to warrant justification of an early biomarker? Does peripheral blood analysis carry the same prognostic merit or implications compared to the analysis of the tumor microenvironment? | ||||
5. | In the broad scheme of things, is there a unifying relationship among MDSCs, immature DCs, and even tumor-associated macrophages or tumor-associated neutrophils? Are they truly distinct populations or is there significant overlap among them at differentiation and/or functional levels? |
Although answers to these and other poignant questions/issues remain the scope of ongoing and future work, what remains certain is the drive to expand our understanding of the complexity of the host-tumor interaction in the “war against cancer.” We hope that this issue collectively brings to light compelling evidence of significant progress toward this great clinical challenge.
Declaration of Interest: Funding: R01CA140622 from the NCI/NIH (to S.I.A).
Scott Abrams
REFERENCES
- Cheng, P., Corzo, C. A., Luetteke, N., Yu, B., Nagaraj, S., Bui, M. M., Ortiz, M., Nacken, W., Sorg, C., Vogl, T., (2008). Inhibition of dendritic cell differentiation and accumulation of myeloid-derived suppressor cells in cancer is regulated by S100A9 protein. J Exp Med 205: 2235–2249.
- Cohen, P. A., Ko, J. S., Storkus, W. J., Spencer, C. D., Bradley, J. M., Gorman, J. E., McCurry, D. B., Zorro-Manrique, S., Dominguez, A. L., Pathangey, L. B., (2012). Myeloid-derived suppressor cells adhere to physiologic STAT3- vs STAT5-dependent hematopoietic programming, establishing diverse tumor-mediated mechanisms of immunologic escape. Immunol Invest, this issue.
- Gabrilovich, D. I., Ostrand-Rosenberg, S., Bronte, V. (2012). Coordinated regulation of myeloid cells by tumours. Nat Rev Immunol 12: 253–268.
- Haile, L., Greten, T., and Korangy, F. (2012). Immune suppression: the hallmark of myeloid-derived suppressor cells. Immunol Invest, this issue.
- Harden, J., Egilmez, N. (2012). Indoleamine 2,3-dioxygenase and dendritic cell tolerogenicity. Immunol Invest, this issue.
- Johnson, T., Munn, D. H. (2012). Host indoleamine 2,3-dioxygenase: contribution to systemic acquired tumor tolerance. Immunol Invest, this issue.
- Kohanbash, G., Okada, H. (2012). Myeloid-derived suppressor Cells (MDSCs) in gliomas and glioma-development. Immunol Invest, this issue.
- Kraman, M., Bambrough, P. J., Arnold, J. N., Roberts, E. W., Magiera, L., Jones, J. O., Gopinathan, A., Tuveson, D. A., Fearon, D. T. (2010). Suppression of antitumor immunity by stromal cells expressing fibroblast activation protein-alpha. Science 330: 827–830.
- Luo, Y., Zhou, H., Krueger, J., Kaplan, C., Lee, S. H., Dolman, C., Markowitz, D., Wu, W., Liu, C., Reisfeld, R. A., Xiang, R. (2006). Targeting tumor-associated macrophages as a novel strategy against breast cancer. J Clin Invest 116:2132–2141.
- Manjili, M. H. (2012). Phenotypic plasticity of MDSC in cancers. Immunol Invest, this issue.
- Monu, N. R., Frey, A. B. (2012). Myeloid-derived suppressor cells and anti-tumor T cells: a complex relationship. Immunol Invest, this issue.
- Munn, D. H., Sharma, M. D., Lee, J. R., Jhaver, K. G., Johnson, T. S., Keskin, D. B., Marshall, B., Chandler, P., Antonia, S. J., Burgess, R., (2002). Potential regulatory function of human dendritic cells expressing indoleamine 2,3-dioxygenase. Science 297:1867–1870.
- Obermajer, N., Wong, J. L., Edwards, R. P., Odunsi, K., Moysich, K., Kalinski, P. (2012). PGE2-driven induction and maintenance of cancer-associated myeloid-derived suppressor cells. Immunol Invest, this issue.
- Qu, P., Boelte, K. C., Lin, P. C. (2012). Negative regulation of myeloid-derived suppressor cells in cancer. Immunol Invest, this issue.
- Raber, P., Ochoa, A., Rodriguez, P. (2012). Metabolism of L-arginine by myeloid-derived suppressor cells in cancer: mechanisms of T cell suppression and therapeutic perspectives. Immunol Invest, this issue.
- Rosenbauer, F., Tenen, D. G. (2007). Transcription factors in myeloid development: balancing differentiation with transformation. Nat Rev Immunol 7:105–117.
- Sawanobori, Y., Ueha, S., Kurachi, M., Shimaoka, T., Talmadge, J. E., Abe, J., Shono, Y., Kitabatake, M., Kakimi, K., Mukaida, N., Matsushima, K. (2008). Chemokine-mediated rapid turnover of myeloid-derived suppressor cells in tumor-bearing mice. Blood 111:5457–5466.
- Sica, A., Mantovani, A. (2012). Macrophage plasticity and polarization: in vivo veritas. J Clin Invest 122:787–795.
- Sinha, P., Okoro, C., Foell, D., Freeze, H. H., Ostrand-Rosenberg, S., Srikrishna, G. (2008). Proinflammatory S100 proteins regulate the accumulation of myeloid-derived suppressor cells. J Immunol 181:4666–4675.
- Solito, S., Pinton, L., Damuzzo, V., Mandruzzato, S. (2012). Highlights on molecular mechanisms of MDSC-mediated immune suppression: paving the way for new working hypotheses. Immunol Invest, this issue.
- Stewart, T. J., Greeneltch, K. M., Reid, J. E., Liewehr, D. J., Steinberg, S. M., Liu, K., Abrams, S. I. (2009a). Interferon regulatory factor-8 modulates the development of tumour-induced CD11b+Gr-1+ myeloid cells. J Cell Mol Med 13:3939–3950.
- Stewart, T. J., Liewehr, D. J., Steinberg, S. M., Greeneltch, K. M., Abrams, S. I. (2009b). Modulating the expression of IFN regulatory factor 8 alters the protumorigenic behavior of CD11b+Gr-1+ myeloid cells. J Immunol 183:117–128.
- Tadmor, T., Attias, D., Polliack, A. (2011). Myeloid-derived suppressor cells–their role in haemato-oncological malignancies and other cancers and possible implications for therapy. Br J Haematol 153:557–567.
- Waight, J. D., Hu, Q., Miller, A., Liu, S., Abrams, S. I. (2011). Tumor-derived G-CSF facilitates neoplastic growth through a granulocytic myeloid-derived suppressor cell-dependent mechanism. PLoS One 6: e27690.