Abstract
Dry eye is a common ocular surface inflammatory disease that significantly affects quality of life. Dysfunction of the lacrimal function unit (LFU) alters tear composition and breaks ocular surface homeostasis, facilitating chronic inflammation and tissue damage. Accordingly, the most effective treatments to date are geared towards reducing inflammation and restoring normal tear film. The pathogenic role of CD4+ T cells is well known, and the field is rapidly realizing the complexity of other innate and adaptive immune factors involved in the development and progression of disease. The data support the hypothesis that dry eye is a localized autoimmune disease originating from an imbalance in the protective immunoregulatory and proinflammatory pathways of the ocular surface.
THE PROBLEM
Dry eye was traditionally considered a condition of reduced tear volume. It is now recognized in a broader context as a condition of abnormal tear composition that no longer adequately supports the ocular surface. Tear dysfunction occurs when the lacrimal functional unit (LFU) (), composed of the tear secreting glands (lacrimal glands, conjunctival goblet cells, and meibomian glands) and their neural and immunological components [Citation1–3], is no longer able to maintain a stable precorneal tear layer. Altered tear film is a consequence of disease or dysfunction of one or more components of the LFU. The etiology of LFU dysfunction is obscure; environmental, microbial and endogenous stress, antigen localization, and genetic factors may provide the trigger for an acute inflammatory event that initiates the inflammatory circle of chronic dry eye originally published by our labs in The Ocular Surface in 2005 ()[Citation4].
FIGURE 1. The Lacrimal Functional Unit. The LFU unifies the complex reflex network connecting the sensory tissues and secretory glands that provide homeostasis on the ocular surface, and is composed of the ocular surface tissues (cornea, corneal limbus, conjunctiva, conjunctival blood vessels, and eyelids), the tear secreting machinery (main and accessory lacrimal glands, meibomian glands, conjunctival goblet, and epithelial cells), and their neural connections. The LFU is tightly controlled by neural input from the ocular surface tissues. Subconscious stimulation of the corneal nerve endings triggers afferent impulses through the ophthalmic branch of the trigeminal nerve (V), which integrate in the central nervous system and the paraspinal sympathetic tract, in turn generating efferent secretomotor impulses that stimulate secretion of the healthy tear film. Any one of several sensory stimuli, e.g., pain, microbial/environmental insult, and emotion can stimulate the tear secreting reflex. Illustration from Beuerman et al. The Lacrimal Functional Unit in Dry Eye and Ocular Surface Disorders (eds. Pflugfelder SC, Beuerman RW, and Stern ME) (Marchel Dekker, Inc., New York, 2004) 11–39.
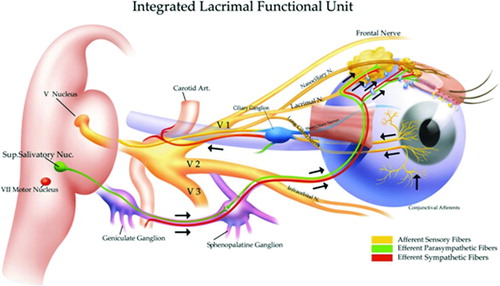
FIGURE 2. Inflammatory circle of chronic dry eye. Stress to the ocular surface triggers the initial events leading to localized autoimmunity. Acute response cytokines, such as TNF-α, IL-1α, IL-1β, and IL-6 further enhance proinflammatory cytokine/chemokine production, adhesion molecule expression required for innate cell infiltration, and also activate resident antigen presenting cells (APCs). Mature APCs home to the regional lymph nodes to activate Th1 and Th17 cells. Autoreactive T cells traffic to the ocular surface tissues where they potentiate the chronic autoimmune response and cause pathology. For example, IFN-γ alters mucins on corneal epithelial cells and is linked to epithelial cell apoptosis, goblet cell loss, and squamous metaplasia. IL-17 increases MMP3/9 expression and induces corneal epithelial barrier dysfunction. In addition, recent data suggest that autoantibodies bind to antigens expressed in the LFU to cause complement-dependent tissue destruction.
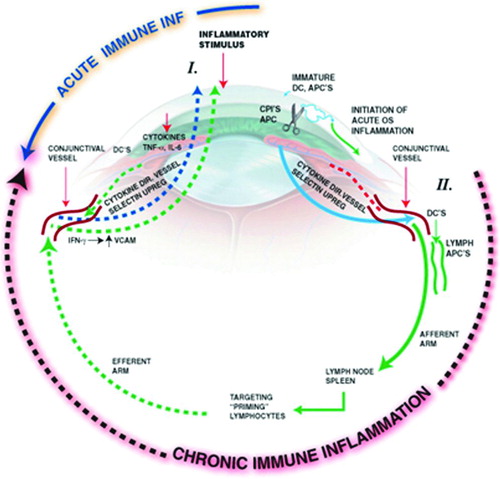
Tear dysfunction is one of the most prevalent eye conditions. Epidemiological studies performed worldwide on different populations and using a variety of diagnostic criteria have reported a prevalence ranging from 2%–14.4% [Citation5–10]. This translates to dry eye prevalence in the United States of 6 to 43.2 million people. A number of risk factors for dry eye have been identified. Age is perhaps the biggest risk factor with the prevalence increasing in both men and women with every decade of life over the age of 40, with a greater prevalence in women than men at every age [Citation9,10]. Other risk factors identified include contact lens wear [Citation11], high dietary consumption of n-6 polyunsaturated essential fatty acids [Citation12], diabetes mellitus [Citation9,10], cigarette smoking [Citation10,Citation13], prolonged video display viewing [Citation11], and low-humidity environments [Citation14]. Recently, ocular surface wetness was shown to be regulated by corneal TRPM8-dependent cold thermoreceptors [Citation15], and it is possible that these fibers, along with other nerve fibers [Citation16], may be reduced with aging, drawing a link between aging, corneal innervation, and tearing.
CLINICAL MANIFESTATIONS OF DRY EYE
Patients with tear dysfunction typically experience intermittent-to-constant eye irritation, photophobia, and blurred and fluctuating vision. These symptoms are often exacerbated by prolonged visual effort or a low-humidity environment, such as an airplane cabin. Chronic eye irritation may decrease quality of life in afflicted patients. In fact, the impact of tear dysfunction on quality of life was rated to be equivalent to unstable angina using utility assessments [Citation17]. In some cases, the consequences of tear dysfunction can be devastating and result in functional and occupational disability.
Ocular surface pain and discomfort is a major symptom of chronic dry eye and is frequently the primary reason patients seek an ophthalmologist. Clinically, there is disparity in the extent of tearing, corneal innervation, sensitivity, and pain among the patient population [Citation18–24]. Although not confirmed, ocular surface discomfort may be a sensory neuropathy caused by repeated stimulation of peripheral corneal nerve fibers from the ophthalmic branch of the trigeminal nerve. Certainly, small diameter myelinated and unmyelinated axons are present in the cornea and are potential targets for peripheral nerve disorders. Inflammatory mediators released from the tissues, and the damaged nerves, may overstimulate pain fibers, ultimately leading to the development of central sensitization; factors associated with inflammatory pain, including neuropeptides [Citation25,26], proinflammatory cytokines [Citation27], ganglioside-specific antibodies [Citation28,29], and infiltrating inflammatory cells [Citation30] are well documented during dry eye. Using the desiccating stress-induced murine model, we recently demonstrated that dry eye mice developed tactile allodynia indicative of sensory neuropathy (Schaumburg and Stern, unpublished observations). Dry Eye mice displayed tactile allodynia in the infraorbital branch of the maxillary nerve (V2 sensory region), which was associated with increased levels of neuropeptides, e.g., calcitonin-gene-related peptide (CGRP) and substance P in the trigeminal ganglia. Neuropathic pain associated with tear dysfunction may also be more common in patients with tear dysfunction than is currently recognized. It is not uncommon for patients with chronic tear instability to develop irritation symptoms out of proportion to the severity of their corneal epithelial disease. In some cases, agents approved for treatment of neuropathic pain, such as pregabalin may improve these symptoms.
IMMUNOREGULATION OF THE OCULAR MUCOSA
Like other mucosal tissues, the eye contains an exquisite immunoregulatory network designed to limit bystander tissue damage during microbial insults and maintain tolerance to self-antigens and commensal microbes. Like the gut, the eye contains its own local lymphoid tissues, i.e., conjunctiva-associated lymphoid tissue (CALT), situated to sample antigens and maintain tolerance to commensal flora [Citation31–35]. Using two-photon microscopy, Steven et al. showed that topical stimulation of the ocular surface with microbe/microbial products (Chlamydia trachomatis, cholera toxin B) or a ubiquitous antigen (ovalbumin) increased the number of intraepithelial lymphocytes and CALT follicles consisting of lymphocytes, dendritic cells (DC), and macrophages in the nictitating membrane [Citation33]; use of this novel mouse model will lead to a greater understanding of the functional role of CALT in homeostasis and disease. We are also now realizing that the eye, like other mucosal sites, has a diverse microbiome [Citation36] whose composition may be regulated by the antimicrobial and immunomodulatory factors in tears. Together, the immune cells that reside in the ocular surface and the factors they produce regulate immune homeostasis on the ocular surface.
Tears contain a biochemically complex mixture of factors that are produced by the lacrimal glands and ocular surface epithelium that function to maintain corneal clarity by lubricating, supporting, and healing the cornea, as well as suppressing inflammation, microbial invasion, and tissue destruction () [Citation37–51]. Reflex tear secretion, blinking, and drainage of tears into the nasolacrimal drainage system normally clear potentially pathogenic inflammatory mediators that are produced by the resident epithelial and inflammatory cells. Additionally, tears contain a variety of anti-inflammatory factors, such as transforming growth factor beta (TGF-β), interleukin-1-receptor antagonist (IL-1RA), tissue inhibitor of matrix metalloproteinase (TIMP-1), decay accelerating factory (DAF; CD55), membrane inhibitor of active lysis (MIRL; CD59), and programmed death ligand (PD-L1) [Citation27,Citation52–55]. For example, TGF-β [Citation40,Citation56) and the IL-1 receptor antagonist (IL-1RA) [Citation57,58] can suppress resident DC maturation, required for T-cell activation during experimental dry eye [Citation59], by direct and indirect mechanisms, respectively. DAF (CD55) and MIRL (CD59) are present in the cornea and conjunctival tissues/tears and may limit complement-mediated tissue damage during ocular insults [Citation54,55,Citation60]; the potential role of complement in experimental dry eye [Citation61] will be discussed later. In addition, PD-L1 protects the ocular surface from T-cell-mediated damage in murine models of corneal transplant and dry eye disease [Citation62–65]. However, decreased tear production and clearance during disease of the LFU or in the closed eye during sleep cause the levels of inflammatory mediators in tears to increase, which may compromise ocular surface homeostasis [Citation53,Citation66,Citation67].
TABLE 1. Tear components supporting corneal health.
Intraepithelial lymphocytes (e.g., CD8+, γδ, and NKT cells) and CD4+ T regulatory cells (Tregs) on the ocular surface may provide protection against autoimmunity, as demonstrated at other mucosal sites [Citation68]. For example, CD8+ T cells predominate the ocular surface epithelium [Citation69], and we recently demonstrated that a subset of CD8+ suppressor cells exert regulatory function during desiccating stress-induced Dry Eye (Zhang, De Paiva, and Pflugfelder, unpublished observations). We have also demonstrated that CD4+CD25hiFoxp3+ Tregs protect mice exposed to desiccating stress from developing disease. When the Tregs were depleted mice developed full-blown disease [Citation70]; by contrast, co-transfer of CD4+CD25hiFoxp3+ cells dampened the capacity of dry eye-specific pathogenic CD4+ T cells to cause disease in T-cell-deficient recipient mice [Citation71]. Current studies are focused on identifying additional regulatory cell types and specific mechanisms used by these cells to inhibit T-cell activation, differentiation, proliferation, and effector function of pathogenic immune cells.
INNATE IMMUNE RESPONSE
What Triggers Inflammation in the LFU?
The immunological factors that initiate the development of dry eye disease are unknown. Based on our current understanding, it is conceivable that acute inflammatory episodes caused by environmental and/or microbial stress, in the context of hormone imbalance and/or genetic predisposition are sufficient to break immunological tolerance and set the stage for LFU dysfunction and abnormal tear film. Increased tear osmolarity in dry eye has been recognized for decades. Clinical studies have reported a 10%–20% mean increase in tear osmolarity of the inferior tear meniscus [Citation72,73]; ocular surface epithelial cells underlying areas of marked thinning or frank break-up of the tear layer may be subjected to much greater osmotic stress [Citation74]. In a murine model of dry eye, sodium ion concentration increased in tear washings compared to controls and accounted for a doubling in tear osmolarity [Citation75].
Stress to the ocular surface activates signaling pathways in a variety of cell types, including the ocular surface epithelia. Desiccating or osmotic stress to the ocular surface epithelium is sufficient to activate MAPK and nuclear factor (NF)-κB [Citation76–80]. We reported that exposure of cells to increased osmolarity in vivo or in vitro activates mitogen-activated protein kinase (MAPK) pathways, particularly p38 and c-Jun N-terminal kinases (JNK), and NF-κB in the ocular surface epithelia [Citation77,78,Citation80,81]. These pathways regulate transcription of a wide variety of genes involved in the inflammatory/immune response. We found desiccating and osmotic stress, through activation of MAPKs, stimulates production of a variety of inflammatory mediators by the ocular surface epithelium, including interleukin (IL)-1β, tumor necrosis factor (TNF)-α, and IL-8, as well as a number of matrix metalloproteinases (MMPs; MMP-1, -3, -9, -10, and -13) [Citation76,77,Citation81], which create an inflammatory milieu on the ocular surface (). Epithelial-derived factors, such as IL-1β and TNF-α can activate immature resident corneal DCs, and mediate recruitment into the cornea through upregulation of CC chemokine receptor 5 (CCR5) [Citation82]. Dry eye also induces loss of conjunctival goblet cells that produce and secrete the immunoregulatory molecule transforming growth factor-β2 (TGF-β2) reported to suppress activation of ocular surface DCs [Citation38]. However, the underlying mechanisms that trigger disease in humans are likely more complex than induction of a general stress response [Citation83].
TABLE 2. Stress-specific expression of proinflammatory mediators in corneal and conjunctival epithelium.
Activation of pattern recognition receptors (PRRs), such as the membrane-bound Toll-like receptors (TLRs), and/or the NOD-like receptor family (NLR) and AIM2 (absent in melanoma 2) cytosolic inflammasome mediators have been implicated in early induction of proinflammatory factors and innate immune activation in virtually every mucosal inflammatory disease. Dry eye is no exception. Many of the known TLRs are expressed within the ocular surface tissues (reviewed in [Citation84]), and there is evidence to suggest a functional role in the pathogenesis of dry eye. For example, stimulation with the dsRNA mimetic polyI:C resulted in production of IL-1β and IL-6 in corneal epithelial cells [Citation85]. More recently, TLR4 signaling was important for inducing upregulation of IL-1β, IL-6, and TNF-α, and subsequent accumulation of infiltrating CD11b+ monocytes during the development of desiccating stress-induced dry eye [Citation86]. While the data are intriguing, the ligands that contribute to the initiation of autoimmune-based inflammation during dry eye are still unknown.
In absence of pathogenic challenge, host-derived DNA and/or RNA from apoptotic and necroptotic cells can activate nucleic acid-sensing endolysosomal TLR3 (dsRNA), TLR7/8 (ssRNA), and TLR9 (dsDNA) in the context of systemic autoimmune diseases, such as systemic lupus erythematosus and Sjögren's Syndrome [Citation87,88]. With regard to both Sjögren's and non-Sjögren's dry eye, elevated epithelial apoptosis was noted during the early stages of environmentally induced experimental disease [Citation79,Citation89], in dogs with spontaneous keratoconjunctivis sicca [Citation90] and in human disease [Citation91]. Nuclear complexes containing RNA derived from dying cells in Sjögren's patients were sufficient stimulators of first line anti-viral sensors, plasmacytoid dendritic cells (pDC), even though there was no virus present [Citation92]. Along these lines, type I interferon signaling pathways were enhanced in Sjögren's patients [Citation93], and recent data from our lab suggests desiccating stress stimulates activation and accumulation of pDCs within the conjunctiva during the immunopathogenesis of environmentally induced experimental dry eye disease (Stern and Schaumburg, unpublished observations). Collectively, the data imply that endogenous nucleic acids may trigger TLRs and result in induction of mucosal autoimmunity during the development of dry eye disease.
Early Innate effectors
Acute response cytokines
Early expression of proinflammatory factors, namely IL-1β, IL-6, and TNF-α, amplify the innate inflammatory response by enhancing proinflammatory cytokine/chemokine production, adhesion molecule expression required for innate cell infiltration, and by activating resident antigen presenting cells (APCs). In response to stress, ocular surface epithelial cells are a prominent source of acute response cytokines and chemokines [Citation70,Citation76,Citation78,Citation80,Citation85,Citation94]. The observation that IL-1 receptor knock-out mice displayed an attenuated proinflammatory cytokine response, including TNF-α and IL-6, suggests a critical role of IL-1 in initiating the early stages of environmentally induced disease [Citation95]. IL-1, together with TNF-α, can amplify the innate inflammatory response by several mechanisms, for example, by driving endothelial adhesion molecule expression, such as ICAM-1 [Citation96,97], chemokine production (e.g., CCL3, CCL4, CCL5, CXCL9, CXCL10, and CX3CL1) [Citation98–101], and by upregulating costimulatory molecules (CD80/86), MHC class II and CCR7 on resident APCs [Citation59,Citation102–106]. IL-1β and TNF-α also upregulated MMP-9 in corneal epithelial cells [Citation107], which destabilized the tear film and directly contributed to corneal barrier dysfunction by breaking down tight junctions and facilitating inflammatory cell migration [Citation42,Citation76,Citation107,108]. Blocking IL-1 signaling using an IL-1 receptor antagonist decreased the number of CD11b+ cells and reduced corneal epithelial barrier dysfunction during desiccating stress-induced dry eye [Citation109]. In addition to its anit-inflammatory properties, epithelial cell-derived TGF-β (85) can also induce MMP-9 production [Citation110–112] during desiccating stress, and may also contribute to fibrosis on the ocular surface. Furthermore, there are several types of resident cells, including macrophages, DCs, γδ T cells, and infiltrating innate cells, such as natural killer (NK) cells and monocytes that act in concert to potentiate the innate response, cause direct tissue damage, and coordinate the chronic antigen-driven autoimmune response [Citation106,Citation113,Citation114]. The pathogenic role of these innate cell types in dry eye is now being realized.
γδ T cells
γδ T cells are a small subset of innate lymphocytes that produce cytokines and exert cytotoxic effector function in both health and disease. Resident intraepithelial γδ T cells are important regulators of tissue homeostasis in the skin and mucosal tissues [Citation115]. Pathologically, γδ T cells are an important source of IL-17 during antigen-induced autoimmunity, including experimental autoimmune encephalomyelitis (EAE) [Citation116], collagen-induced arthritis [Citation117], and uveitis [Citation118]. Indeed, resident γδ T cells are present in the ocular surface tissues [Citation106,Citation119,Citation120] and IL-17 expression was seen early in the ocular surface tissues and tears during the development of desiccating stress-induced dry eye, before infiltrating T cells were present (). The observation that T and B cell-deficient RAG mice also produced high levels of IL-17 in response to IL-23 stimulation suggests that there are other innate immune cells that produce IL-17 during the development and progression of disease [Citation121]. In support, NK/NK T cells purified from ocular surface cells of dry eye mice upregulated IL-17 by 1 day of desiccating stress [Citation106]. Moreover, ocular surface cells from dry eye mice depleted of NK/NK T cells expressed significantly higher levels of IL-17 than purified NK/NK T cells (), suggesting γδ T cells, and possibly other innate cell types, are the primary reservoir of IL-17, before Th17 cells arrive on the ocular surface.
FIGURE 3. γδ T cells and upregulation of IL-17 on the ocular surface early during the immunopathogenesis of desiccating stress (DS)-induced dry eye disease. (A) Immunohistochemistry on sagittal sections of whole eyes showed positive staining for γδ T cells using purified hamster anti-mouse γδ T cell receptor (8.75 μg/ml; BD Pharmingen). Images captured at 40×. (B) Reproduced from Zhang et al. [Citation106] mRNA levels in NK/NKT positive (+) and NK/NKT negative (–) ocular surface cells isolated from nonstressed (NS) spleen and ocular surface (OS) and at different time points after DS. Unfractionated spleen was used as a calibrator. *indicates p <0.05, ***indicates p < 0.001 comparison versus NS control NK/NKT+. ∧ indicates p < 0.05, ∧∧∧ indicates p < 0.001 comparison versus NS control NK/NKT. (C) Tear levels of IL-17. Relative levels of IL-17 in the tears of dry eye using Luminex analysis as previously described [Citation71]. (B and C) DS; DS1 = DS for 1 day, DS3 = DS for 3 days, DS5 = DS for 5 days, DS10 = DS for 10 days.
![FIGURE 3. γδ T cells and upregulation of IL-17 on the ocular surface early during the immunopathogenesis of desiccating stress (DS)-induced dry eye disease. (A) Immunohistochemistry on sagittal sections of whole eyes showed positive staining for γδ T cells using purified hamster anti-mouse γδ T cell receptor (8.75 μg/ml; BD Pharmingen). Images captured at 40×. (B) Reproduced from Zhang et al. [Citation106] mRNA levels in NK/NKT positive (+) and NK/NKT negative (–) ocular surface cells isolated from nonstressed (NS) spleen and ocular surface (OS) and at different time points after DS. Unfractionated spleen was used as a calibrator. *indicates p <0.05, ***indicates p < 0.001 comparison versus NS control NK/NKT+. ∧ indicates p < 0.05, ∧∧∧ indicates p < 0.001 comparison versus NS control NK/NKT. (C) Tear levels of IL-17. Relative levels of IL-17 in the tears of dry eye using Luminex analysis as previously described [Citation71]. (B and C) DS; DS1 = DS for 1 day, DS3 = DS for 3 days, DS5 = DS for 5 days, DS10 = DS for 10 days.](/cms/asset/f544acd1-3acb-481f-81f7-3bd3ebebb46f/iiri_a_748052_f0003_b.jpg)
NK cells
Recent evidence suggests NK cells are important innate effectors during the immunopathogenesis of desiccating stress-induced dry eye. Accumulation of NK cells within the ocular surface tissues by 1 day of desiccating stress correlated with increased levels of IL-6, IL-23, IFN-γ, and IL-17 [Citation106,Citation122]. Chen et al. showed that NK cells are an early source of IFN-γ, and that NK cell depletion reduced costimulatory molecule and MHCII expression on CD11b+ and CD11c+ APCs in the draining lymph nodes, along with overall disease severity [Citation122]. Studies from our lab went on to show that NK cells from dry eye mice also produced IL-6, IL-23, and IL-17, and NK cell-depleted dry eye mice displayed a significant reduction in pathogenic Th17 cells and reduced corneal barrier dysfunction [Citation106].
Beyond APC modulation, cytokines produced by NK cells are also linked to tissue pathology. IFN-γ is associated with reduced goblet cell density, altered corneal epithelial mucins, and/or squamous metaplasia [Citation123–125], and IL-17 was shown to activate MMPs and promote corneal epithelial barrier dysfunction [Citation126]. In addition to infiltrating NK cells, resident NK/NK T cells are present on the ocular surface [Citation106,Citation113,Citation127]. Resident NK/NK T cells secrete IL-13 to protect goblet cells and maintain ocular surface homeostasis [Citation127], and it is likely that these cells are a proinflammatory reservoir under stress [Citation106,Citation113,Citation127]. To date, it is evident that NK cells are a prominent source of key inflammatory mediators, which exert direct pathogenic effects on the ocular surface and enhance APC maturation required for subsequent activation of autoreactive Th1 and Th17 cells during desiccating stress [Citation106,Citation122]. However, the role of NK cells in human dry eye disease is unknown.
Monocytes
Infiltrating monocytes/macrophages deliver a variety of proinflammatory cytokines and act as APCs during autoimmunity. Infiltration of monocyte/macrophages, using markers such as CD11b and CD14, was demonstrated in both human and the desiccating stress-induced model of dry eye, and appear to be critical mediators of full-blown experimental disease [Citation103,Citation113,Citation128]. For example, blocking CD11b+ monocyte infiltration using a CCR2 antagonist dampened proinflammatory cytokine expression (e.g., IL-1β), pathogenic T-cell infiltration, and the overall severity of desiccating stress-induced dry eye disease [Citation128]. Our recent studies also indicate that CD11b+ (monocytes/macrophages) and CD11c+ (DCs) cells play a pivotal role as APCs during the development and progression of dry eye disease: i) by bridging the innate and adaptive immune response to activate autoreactive T cells and ii) by maintaining primed and targeted T cells at the ocular surface [Citation59].
Bridging the Innate Immune Response
Antigen presenting cells
APCs, most notably resident DCs, provide the fundamental link between the relatively nonspecific innate immune response and development of the antigen-specific adaptive response in the regional lymph nodes [Citation129,130]. We previously demonstrated that mice exposed to desiccating stress develop clinical and histopathological similarities to the human disease, including a robust CD4+ T cell infiltrate into the LFU [Citation70,Citation131]; these CD4+ T cells were sufficient to mediate full-blown dry eye disease when adoptively transferred to athymic T-cell-deficient nude-recipient mice [Citation70] (). Now, there is convincing evidence that APCs are necessary for activation of autoreactive T cells during the development and progression of dry eye. APCs are present in the healthy corneal stroma [Citation132–134] and were shown to increase in frequency and/or number, and upregulate expression of costimulatory molecules CD80/86, MHC II required for antigen presentation to CD4+ T cells, and the chemokine receptor CCR7 [Citation59,Citation102–106]. Trafficking of APCs from the ocular surface to the draining cervical lymph nodes (CLN) was dependent on CCR7:CC21 signaling [Citation133], and was enhanced by corneal lymphangiogenesis during desiccating stress-induced experimental dry eye [Citation135]. Indeed, accumulation of mature CD11c+ DCs in the draining CLN occurred by 24 hours of desiccating stress, and correlated with subsequent activation of CD4+ T cells with the kinetics indicative of an antigen-driven T-cell response [Citation59].
FIGURE 4. Desiccating stress-induced model of dry eye. Female C57BL/6 mice (6–8 weeks old) exposed to desiccating stress (DS: subcutaneous scopolamine (1 mg/0.2 ml) three times per day, humidity <40%, and sustained airflow) display similar clinical and histopathological features to patients with dry eye disease. Moreover, CD4+ T cells isolated from the cervical lymph nodes and spleen of dry eye mice are sufficient to cause disease in T-cell-deficient nude-recipient mice. Desiccating stress-induced model of dry eye was originally described in Dursun et al. 2002. IOVS. 43[Citation3]:632–638 and Niederkorn et al. 2006. J. Immunol. 176: 3950–3957 (70;131).
![FIGURE 4. Desiccating stress-induced model of dry eye. Female C57BL/6 mice (6–8 weeks old) exposed to desiccating stress (DS: subcutaneous scopolamine (1 mg/0.2 ml) three times per day, humidity <40%, and sustained airflow) display similar clinical and histopathological features to patients with dry eye disease. Moreover, CD4+ T cells isolated from the cervical lymph nodes and spleen of dry eye mice are sufficient to cause disease in T-cell-deficient nude-recipient mice. Desiccating stress-induced model of dry eye was originally described in Dursun et al. 2002. IOVS. 43[Citation3]:632–638 and Niederkorn et al. 2006. J. Immunol. 176: 3950–3957 (70;131).](/cms/asset/ad5f55e1-1ff9-4356-b299-fae9a63c255e/iiri_a_748052_f0004_b.jpg)
APCs are necessary for both the generation and maintenance of ocular-specific autoreactive CD4+ T cells during desiccating stress-induced disease supporting the long-standing hypothesis that dry eye is a local self-antigen-driven autoimmune disease [Citation59]. Van Rooijen et al. previously showed liposome-encapsulated clodronate induces apoptosis of macrophages [Citation136]. In the context of the ocular surface, subconjunctival injection of clodronate-loaded liposomes depleted CD11b+ and CD11c+ APCs, which was associated with a reduction in the number of infiltrating CD4+ T cells and preservation goblet cells in mice exposed to desiccating stress [Citation59]. Surgical lymphadenectomy also attenuated CD4+ T-cell infiltration and protected goblet cell density in dry eye mice. Functionally, CD4+ T cells isolated from the draining CLN of APC-depleted mice were not pathogenic in T-cell-deficient recipient mice, indicating APCs are necessary for antigen-specific activation of dry eye-specific autoreactive lymphocytes (). Furthermore, pathogenic dry-eye-specific CD4+ T cells did not accumulate in the ocular surface tissues of APC-depleted mice that were not exposed to desiccating stress, suggesting that resident ocular surface APCs are required for T-cell maintenance and effector function. Whether or not antigen re-exposure is a requisite for reactivation and maintenance of activated T cells at sites of inflammation (e.g., interaction with DCs and/or macrophages) is still a topic of debate. The observation that MHCII is also upregulated on corneal and conjunctival epithelial cells under inflammatory conditions [Citation30,Citation96,Citation104,Citation113,Citation137] suggests that these non-professional APCs contribute to secondary activation or triggering of pathogenic T cells.
FIGURE 5. Antigen-presenting cell depletion in mice exposed to desiccating stress attenuating the generation of autoreactive CD4+ T cells and blocking the ability to adoptively transfer T-cell-mediated disease to nude-recipient mice. CD4+ T cells were isolated from the CLN of clodronate- or PBS-treated mice exposed to DS and adoptively transferred to nude-recipient mice to determine if the absence of a full repertoire of APCs within the ocular surface tissues inhibits generation of ocular surface-specific autoreactive CD4+ T cells. (A) H&E staining showed a (B) significant decrease in overall inflammatory cell infiltration within the ocular surface tissues of nude recipients of CD4+ T cells from clodronate-treated donor mice compared to PBS controls (3 days postadoptive transfer). Furthermore, (C) IHC confirmed that CD4+ T cells isolated from clodronate-treated donor mice did not readily accumulate within the conjunctiva as there was only trace CD4+ staining, which accounted for a (D) significant decrease in CD4+ T cells and (E, F) protection from the loss of PAS-positive goblet cells. (A) H&E staining; (B) overall inflammatory score ± SEM (scale 0–3); (C) CD4+ T cell staining in the conjunctiva; (D) is average conjunctival (conj.) CD4+ T-cell counts ± SEM; (E) PAS+ goblet cell staining in the conjunctiva; (F) is average conjunctival goblet cell counts ± SEM. The data are representative of three independent experiments, with an n = 5–6 mice/group. Statistically significant values (*p ≤ 0.05, **p ≤ 0.01) are indicated relative to nude recipients of CD4+ T cells from PBS liposome-treated mice. Reproduced from Schaumburg et al. 2011. J Immunol. 187[Citation7]:3653–3662 [Citation59].
![FIGURE 5. Antigen-presenting cell depletion in mice exposed to desiccating stress attenuating the generation of autoreactive CD4+ T cells and blocking the ability to adoptively transfer T-cell-mediated disease to nude-recipient mice. CD4+ T cells were isolated from the CLN of clodronate- or PBS-treated mice exposed to DS and adoptively transferred to nude-recipient mice to determine if the absence of a full repertoire of APCs within the ocular surface tissues inhibits generation of ocular surface-specific autoreactive CD4+ T cells. (A) H&E staining showed a (B) significant decrease in overall inflammatory cell infiltration within the ocular surface tissues of nude recipients of CD4+ T cells from clodronate-treated donor mice compared to PBS controls (3 days postadoptive transfer). Furthermore, (C) IHC confirmed that CD4+ T cells isolated from clodronate-treated donor mice did not readily accumulate within the conjunctiva as there was only trace CD4+ staining, which accounted for a (D) significant decrease in CD4+ T cells and (E, F) protection from the loss of PAS-positive goblet cells. (A) H&E staining; (B) overall inflammatory score ± SEM (scale 0–3); (C) CD4+ T cell staining in the conjunctiva; (D) is average conjunctival (conj.) CD4+ T-cell counts ± SEM; (E) PAS+ goblet cell staining in the conjunctiva; (F) is average conjunctival goblet cell counts ± SEM. The data are representative of three independent experiments, with an n = 5–6 mice/group. Statistically significant values (*p ≤ 0.05, **p ≤ 0.01) are indicated relative to nude recipients of CD4+ T cells from PBS liposome-treated mice. Reproduced from Schaumburg et al. 2011. J Immunol. 187[Citation7]:3653–3662 [Citation59].](/cms/asset/f7d1fac7-0bdd-411e-ad90-592139654620/iiri_a_748052_f0005_b.jpg)
AUTOREACTIVE LYMPHOCYTES
CD4+ T cells play a primary role in the immunopathogenesis of chronic dry eye. As noted, activated CD4+ T cells are localized within the ocular surface tissues of dry eye patients [Citation30,Citation104,Citation138], CD4+ T cells are sufficient to induce dry eye in mice [Citation70], and compounds that inhibit T cells decrease disease severity in both animals and humans [Citation30,Citation139]. Th1 and Th17 cells are essential for development of desiccating stress-induced dry eye and are thought of as the primary pathogenic players during the progression of disease [Citation27,Citation85,Citation140,141].
Th1 Cells
CD4+ Th1 cells were the first pathogenic lymphocyte subset identified in mouse models and patients with dry eye [Citation70,Citation104,Citation131,Citation142]. Our understanding of the mechanisms involved in activation, trafficking, and effector function of Th1 cells is expanding. IL-12 and IFN-γ influence APC-dependent activation and differentiation of autoreactive Th1 cells [Citation143]. As discussed, depletion of conjunctival APCs in dry eye mice blocked generation of autoreactive CD4+ T cells in the draining CLN [Citation59]. Efferent trafficking of Th1 cells is dependent on adhesion molecule and chemokine/chemokine receptor signaling. Adhesion molecule interactions (e.g., LFA-1:ICAM-1) are hypothesized to contribute to homing and diapedesis of autoreactive T cells [Citation104]. Indeed, IFN-γ facilitates diapedesis of targeted lymphocytes to the ocular surface [Citation144]. In addition, Th1-associated chemokine receptors (e.g., CCR5 and CXCR3) are expressed on CD4+ T cells, and cognate ligands (e.g., CCL5 and CXCL10) are expressed in the ocular surface tissues of animal models and patients with dry eye [Citation98–100,Citation142]. Furthermore, CCL5 and CXCL10 expression was upregulated in human conjunctival epithelial cells in response to cytokine stimulation [Citation145]. In humans, Th1-derived IFN-γ correlated with disease severity [Citation27,Citation104,Citation146], and during desiccating stress-induced dry eye, accumulation of infiltrating Th1 cells was associated with increased cytokine production, epithelial cell apoptosis, reduced goblet cell density, altered mucins, and squamous metaplasia [Citation70,Citation89,Citation98,Citation123,Citation124,Citation147]. Importantly, goblet cell density was not affected in IFN-γ knock-out mice exposed to desiccating stress, confirming specificity to IFN-γ-mediated pathology [Citation124].
Th17 Cells
Th17 cells also appear to be key drivers of chronic dry eye disease. In dry eye mice, Th17 cells are present in the draining CLN and within the LFU [Citation126,Citation140,Citation142], and the cytokines required for Th17 cell differentiation are present in mice and humans [Citation27,Citation85,Citation148]. For example, stress (e.g., hyperosmotic media, a variety of TLR ligands, and TNF-α) stimulated the production of Th17 skewing cytokines (e.g., IL-1, IL-6, IL-23, and TGF-β) in primary human corneal epithelial cells (HCEC) [Citation85]. Moreover, coculture of naïve CD4+ T cells with HCECs (stimulated with polyI:C or TNF-α) was sufficient to drive Th17 polarization, indicated by upregulation of IL-17A, IL-17F, IL-22, CCL20, and STAT3 gene expression, elevated levels of IL-17 in cell supernatant, and increased numbers of IL-17-secreting CD4+ cells [Citation85]. Cytokines required for differentiation and effector function of Th17 cells were also significantly elevated in patients [Citation27,Citation148]. The CCR6:CCL20 signaling access is important for Th17 trafficking in mice and humans [Citation149]. On the ocular surface, CCL20 was upregulated in response to stress [Citation106,Citation126], and recent studies from our lab indicated that CCR6:CCL20 signaling is critical for the development of experimental dry eye (Coursey, De Paiva and Pflugfelder, unpublished observations). In the context of desiccating stress, dry eye-specific Th17 cells inhibited Treg-mediated suppression of pathogenic T cells [Citation140]. Anti-IL-17 treatment decreased the severity of disease, and was associated with reduced IL-6 production, reduction in Th17 expansion, and re-establishment of Treg function [Citation140]. Furthermore, IL-17 neutralization dampened MMP3/9 expression and blocked breakdown of corneal epithelial tight junctions in dry eye mice [Citation126]. These data suggest that Th17 cells suppress peripheral tolerance and cause epithelial cell metaplasia and tissue destruction during the progression of chronic disease.
Autoantigen(s)
There is mounting evidence that dry eye is a localized self-antigen driven autoimmune-based inflammatory disease. Stress, or otherwise, may alter expression and/or localization of endogenous autoantigens and break peripheral tolerance to autoreactive lymphocytes. While putative autoantigens were identified in the context of autoreactive sera from animal models and patients with Sjögren's and non-Sjögren's dry eye, the functional contribution of a specific T-cell immunodominant epitope(s) has not been clearly defined. For example, work from the laboratory of the late Dr. Michael Humphreys-Beher and others established a link between autoantibodies to the type 3 muscarinic acetylcholine receptor (anti-M3R Ab) and the secretory response during the immunopathogenesis of Sjögren's syndrome. Anti-M3R Abs were present in sera of patients and animal models [Citation150–155], and passive transfer of IgG from Sjögren's patients or rodent anti-M3R Abs were sufficient to induce exocrine dysfunction in recipient animals [Citation156,157]. In further support, chronic M3R stimulation caused endosomal proteolytic processing of autoantigens [Citation158], and it is possible that these cryptic M3R epitopes are secreted and sampled by APCs. Putative autoantigens were also identified from the Kallikrein family (e.g., Klk1, Klk13) [Citation159,160]. Jiang et al. induced T-cell-mediated Sjögren's-like disease by immunizing rats with Klk1b22 drawing the association between an autoantigen and experimentally induced T-cell-mediated disease [Citation159]. In addition, our group identified the EGF binding protein Klk13 as a putative B-cell autoantigen, discussed in detail in the following section [Citation61]. In theory, identification of an immunodominant epitope(s) would provide a basis for new animal models (e.g., active immunization) and immunological reagents (e.g., MHC tetramers), but from the perspective of human disease the specific self-antigens are still widely unknown among organ-specific autoimmune diseases.
B Cells and Autoantibodies
The role of B cells in various systemic autoimmune diseases, such as systemic lupus erythematosus, rheumatoid arthritis, and Sjögren's syndrome has been demonstrated. As noted, serum from patients and animal models of Sjögren's syndrome were positive for circulating anti-M3R antibodies [Citation150–155]. This makes sense in that tear secretion is known to be a cholinergic process and interference in this portion of the LFU could result in an altered pattern of aqueous secretion. We have previously published data indicating that Klk13 may be one of the putative antigens in the initiation of dry eye. Using PCR, Klk13 was found in the cornea, conjunctiva, and lacrimal glands of dry eye mice after 5 or 10 days of desiccating stress [Citation61]. B cells can play a variety of roles during autoimmunity; it is possible that these cells function as APCs [Citation161–164] pathogenically through the secretion of various cytokines such as IL-2, IL-12, TNF-α, and IFN-γ [Citation165], and/or as terminally differentiated plasma cells that secrete autoantibodies [Citation166]. These autoantibodies can utilize the Fcγ receptor to recruit inflammatory cells via receptor signaling or through the complement activation cascade [Citation167].
Adoptive transfer of autoantibody-containing serum from mice with experimental dry eye, but not naïve controls, was sufficient to induce dry eye in nude-recipient mice, independent of pathogenic T cells [Citation61]. Nude recipients receiving a 1:1 (donor-to-recipient) equivalent of dry-eye-specific serum from mice exposed to desiccating stress for 3 weeks demonstrated an increase of several cytokines including IL-1α, IL-1β, and TNF-α. Serum derived from hen egg lysozyme-specific B-cell receptor transgenic mice exposed to desiccating stress was not pathogenic suggesting that autoantibodies, and not cytokines or contaminating immune cells present in dry-eye-specific serum, were sufficient to mediate inflammation-induced dry eye in the context of the nude-recipient mouse. Furthermore, IgG purified from the dry eye mouse serum, but not from naïve control mice, maintained the ability to transfer ocular surface disease, including an elevated proinflammatory cytokine response and marked Gr1+ neutrophil infiltrate, which was associated with tissue damage, i.e., goblet cell loss [Citation61]. Collectively, the data are consistent with autoantibody-mediated pathology by Fcγ-receptor-mediated activation/recruitment of neutrophils/macrophages and/or activation of the complement system [Citation167].
Induction of ocular surface inflammation in nude-recipient mice following transfer of dry-eye-specific serum or IgG was dependent on complement activation. The role of complement in recruiting effector cells via C3a/C5a, and increasing the pathogenic effects of autoantigens (C3b/C5b) and its ability to eliminate target cells via formation of a membrane attack complex (MAC) has been well documented. Immunohistochemical staining of mice receiving dry eye antibodies showed the presence of C3b in the conjunctiva, but staining was negative if the transfer was from a control mouse [Citation61]. To determine the role of complement in serum/IgG-mediated disease, Cobra Venom Factor (CVF) was used to systemically deplete complement [Citation168–170]. Complement-depleted nude mice receiving dry eye serum or IgG displayed a marked reduction in inflammatory burden, including a significant decrease in neutrophil infiltration and proinflammatory cytokine/chemokine levels compared to recipient mice with intact complement system that were not treated with CVF. Based on these data, we propose a working hypothesis in which autoantibodies contribute to the predominantly CD4+ T cell-mediated disease by complement-dependent recruitment of innate cells (e.g., neutrophils) to the ocular surface ().
FIGURE 6. Working Hypothesis: the contribution of autoreactive B cells during dry eye. 1) Autoreactive B cells recognize putative dry eye autoantigens through B-cell receptor interactions (e.g., M3R, Klk13), [Citation2] intenalize the antigen, and [Citation3] subsequently present the antigen to [Citation4] Th2 cells: cytokines derived from activated Th2 cells promote B-cell differentiation to [Citation5] plasma cells, which [Citation6] produce autoantibodies (e.g., anti-M3R, anti-Klk13) that [Citation7] cause complement-dependent tissue damage.
![FIGURE 6. Working Hypothesis: the contribution of autoreactive B cells during dry eye. 1) Autoreactive B cells recognize putative dry eye autoantigens through B-cell receptor interactions (e.g., M3R, Klk13), [Citation2] intenalize the antigen, and [Citation3] subsequently present the antigen to [Citation4] Th2 cells: cytokines derived from activated Th2 cells promote B-cell differentiation to [Citation5] plasma cells, which [Citation6] produce autoantibodies (e.g., anti-M3R, anti-Klk13) that [Citation7] cause complement-dependent tissue damage.](/cms/asset/a140271c-d15c-4262-ab16-ebd60aa4f0be/iiri_a_748052_f0006_b.jpg)
THERAPEUTIC STRATEGY
There are a handful of different therapies used to treat dry eye patients according to disease severity () [Citation171]. Artificial tears provide a palliative relief of eye irritation in patients with aqueous tear deficiency, but do not prevent the underlying inflammation or reverse conjunctival squamous metaplasia in chronic dry eye. Combinations of artificial tears, oral omega-3 essential fatty acid supplements, mucin secretagogues, short-term steroids, and daily cyclosporine A are used to combat underlying inflammation and restore normal tear film in patients with mild-to-moderate disease (Level 1–2). Use of more aggressive treatment options, such as autologous serum, oral tetracyclines, prosthetic lens, and systemic immunosuppressants are restricted to patients with more severe forms of dry eye (Level 3–4). Surgical intervention, including tarsorrhaphy and amnionic membrane transplant, is also used in patients with the most severe forms of chronic dry eye (Level 4) often associated with systemic disease, such as Sjögren's and Stevens–Johnson syndrome.
TABLE 3. Dry eye disease severity and treatment strategy.
The most effective treatments are geared toward reducing inflammation and restoring normal tear film. Corticosteroids are potent inhibitors of multiple inflammatory mediators (e.g., cytokines/chemokines, MMPs, and adhesion molecules) that effectively reduce the inflammatory cell burden within the ocular surface tissues. Topical corticosteroid use is highly effective, but is limited to short-term use (Citation2–4 weeks) due to a wide range of potential side effects including glaucoma, cataracts, ocular infection, and apoptosis of ocular surface cells. Topical steroids are used to control episodes of exacerbation and as an adjunct therapy to other treatments, such as the fungal-derived T-cell inhibitor cyclosporine (CsA). Topical CsA (0.05% BID) was FDA-approved in 2002 following two pivotal 6-month independent Phase III clinical trials. Topical CsA (0.05% or 0.1%, BID) treatment resulted in a significant (p ≤ 0.05) improvement in corneal fluorescein staining and tear production compared to patients treated with vehicle alone [Citation172]. In addition to clinical improvement, topical CsA also decreased expression of HLA-DR and IL-6, infiltration of T cells, and increased goblet cells in the conjunctiva of patients with chronic dry eye [Citation30,Citation138,Citation173,174].
Several other immunomodulators are in clinical trial for dry eye disease. Among the putative therapies, SAR1118, a 615 Dalton integrin alphaLbeta2 (LFA-1) inhibitor, showed improvements in corneal staining, total ocular surface disease index (OSDI), and visual-related function OSDI scores compared to placebo in Phase II clinical trial conducted in a controlled adverse environment chamber [Citation175]. LFA-1 is a validated target for inhibiting leukocyte adhesion and infiltration into inflamed tissues, and more recently, blocking cell-to-cell interactions and signal transduction of proinflammatory pathways. SAR1118 is designed to mimic the LFA-1 binding epitope on intercellular adhesion molecule 1 (ICAM-1), thereby interfering with cognate LFA-1:ICAM-1 interactions. Indeed, LFA-1:ICAM-1 is implicated in the immunopathogenesis of CD4+ T-cell-mediated dry eye, and may function in promoting T-cell adhesion/infiltration and activation in the ocular surface tissues [Citation97]. The phase III clinical trial was recently completed, but the results are pending.
CONCLUSION
The wealth of new data from animal models and humans have generated a better understanding of the events leading to tear dysfunction and the immunopathogenesis of dry eye disease. While the etiology remains elusive, there is evidence to support a role of ocular surface stress, genetic factors, and/or hormone imbalance underlying inflammation and dysfunction of the LFU. Autoreactive Th1 and Th17 cells are key pathogenic players, and the essential role of APCs in initiating and maintaining chronic activation of desiccating stress-induced CD4+ T cells in mice supports the long-standing hypothesis that dry eye is a localized autoimmune disease. Recent discoveries have uncovered the pathogenic role of other innate and adaptive immune players, such as monocytes [Citation103,Citation113,Citation128], NK cells [Citation106,Citation122], and autoreactive B-cells/autoantibodies [Citation167], and a greater appreciation of the regulatory mechanisms involved in maintaining ocular surface homeostasis. Still, there is a significant gap in understanding, for example: i) disparities in the signs and symptoms of disease, ii) the interactions between the immune and nervous systems and the development of clinical manifestations, such as pain, and iii) differences in the underlying immunopathology among the patient population and the factors that render some patients more susceptible to environmental exacerbation of disease. As the field advances, we are challenged to translate findings from animal models to humans and develop novel therapeutic strategies designed to reduce key inflammatory pathways and restore healthy tear film while maintaining, or even boosting, the protective immunoregulatory mechanisms.
Declaration of Interest
M.E. Stern and C.S. Schaumburg are employees of Allergan, Inc. S.C. Pflugfelder is a consultant for Allergan, Inc. The authors alone are responsible for the content and writing of the article.
REFERENCES
- Beuerman RW, Maitchouk DY, Varnell RJ, Pedroza-Schmidt L. Interactions between lacrimal function and the ocular surface. Curr Opin Kyoto Cornea Club 1998;2:1–10.
- Stern ME, Beuerman RW, Fox RI, The pathology of dry eye: the interaction between the ocular surface and lacrimal glands. Cornea 1998 Nov;17(6):584–589.
- Stern ME, Beuerman RW, Fox RI, A unified theory of the role of the ocular surface in dry eye. Adv Exp Med Biol 1998;438:643–651.
- McDermott AM, Perez V, Huang AJ, et al. Pathways of corneal and ocular surface inflammation: a perspective from the cullen symposium. Ocul Surf 2005 Oct;3(4 Suppl):S131–S138.
- McCarty CA, Bansal AK, Livingston PM, et al. The epidemiology of dry eye in Melbourne, Australia. Ophthalmology 1998 Jun;105(6):1114–1119.
- Moss SE, Klein R, Klein BE. Prevalence of and risk factors for dry eye syndrome. Arch Ophthalmol 2000 Sep;118(9):1264–1268.
- Moss SE, Klein R, Klein BE. Incidence of dry eye in an older population. Arch Ophthalmol 2004 Mar;122(3):369–373.
- Schaumberg DA, Sullivan DA, Buring JE, Dana MR. Prevalence of dry eye syndrome among US women. Am J Ophthalmol 2003 Aug;136(2):318–326.
- Shimmura S, Shimazaki J, Tsubota K. Results of a population-based questionnaire on the symptoms and lifestyles associated with dry eye. Cornea 1999 Jul;18(4):408–411.
- Schaumberg DA, Dana R, Buring JE, Sullivan DA. Prevalence of dry eye disease among US men: estimates from the Physicians’ Health Studies. Arch Ophthalmol 2009 Jun;127(6):763–768.
- Uchino M, Schaumberg DA, Dogru M, Prevalence of dry eye disease among Japanese visual display terminal users. Ophthalmology 2008 Nov;115(11):1982–1988.
- Miljanovic B, Trivedi KA, Dana MR, et al. Relation between dietary n-3 and n-6 fatty acids and clinically diagnosed dry eye syndrome in women. Am J Clin Nutr 2005 Oct;82(4):887–893.
- Altinors DD, Akca S, Akova YA, Smoking associated with damage to the lipid layer of the ocular surface. Am J Ophthalmol 2006 Jun;141(6):1016–1021.
- Uchiyama E, Aronowicz JD, Butovich IA, McCulley JP. Increased evaporative rates in laboratory testing conditions simulating airplane cabin relative humidity: an important factor for dry eye syndrome. Eye Contact Lens 2007 Jul;33(4):174–176.
- Parra A, Madrid R, Echevarria D, Ocular surface wetness is regulated by TRPM8-dependent cold thermoreceptors of the cornea. Nat Med 2010 Dec;16(12):1396–1399.
- Dvorscak L, Marfurt CF. Age-related changes in rat corneal epithelial nerve density. Invest Ophthalmol Vis Sci 2008 Mar;49(3):910–916.
- Schiffman RM, Walt JG, Jacobsen G, et al. Utility assessment among patients with dry eye disease. Ophthalmology 2003 Jul;110(7):1412–1419.
- Benitez-del-Castillo JM, del Rio T, Iradier T, et al. Decrease in tear secretion and corneal sensitivity after laser in situ keratomileusis. Cornea 2001 Jan;20(1):30–32.
- Tuisku IS, Konttinen YT, Konttinen LM, Tervo TM. Alterations in corneal sensitivity and nerve morphology in patients with primary Sjogren's syndrome. Exp Eye Res 2008 Jun;86:879–885.
- Erdelyi B, Kraak R, Zhivov A, In vivo confocal laser scanning microscopy of the cornea in dry eye. Graefes Arch Clin Exp Ophthalmol 2007 Jan;245(1):39–44.
- Hosal BM, Ornek N, Zilelioglu G, Elhan AH. Morphology of corneal nerves and corneal sensation in dry eye: a preliminary study. Eye (Lond) 2005 Dec;19(12):1276–1279.
- Tuominen IS, Konttinen YT, Vesaluoma MH, et al. Corneal innervation and morphology in primary Sjogren's syndrome. Invest Ophthalmol Vis Sci 2003 Jun;44(6):2545–2549.
- Villani E, Galimberti D, Viola F, The cornea in Sjogren's syndrome: an in vivo confocal study. Invest Ophthalmol Vis Sci 2007 May;48(5):2017–2022.
- Zhang M, Chen J, Luo L, Altered corneal nerves in aqueous tear deficiency viewed by in vivo confocal microscopy. Cornea 2005 Oct;24(7):818–824.
- Lambiase A, Micera A, Sacchetti M, Alterations of tear neuromediators in dry eye disease. Arch Ophthalmol 2011 Aug;129(8):981–986.
- Varnell RJ, Freeman JY, Maitchouk D, Detection of substance P in human tears by laser desorption mass spectrometry and immunoassay. Curr Eye Res 1997;28:960–963.
- Lam H, Bleiden L, de Paiva CS, Tear cytokine profiles in dysfunctional tear syndrome. Am J Ophthalmol 2009 Feb;147:198–205.
- Bansal AS, Abdul-Karim B, Malik RA, IgM ganglioside GM1 antibodies in patients with autoimmune disease or neuropathy, and controls. J Clin Pathol 1994 Apr;47(4):300–302.
- Giordano N, Lucani B, Amendola A, IgG and IgM antiganglioside M1 antibodies in primary Sjogren's syndrome with and without peripheral neuropathy. Clin Rheumatol 2003 Sep;22(3): 256–258.
- Kunert KS, Tisdale AS, Stern ME, Analysis of topical cyclosporine treatment of patients with dry eye syndrome: effect on conjunctival lymphocytes. Arch Ophthalmol 2000 Nov;118(11):1489–1496.
- Knop E, Knop N. Lacrimal drainage-associated lymphoid tissue (LDALT): a part of the human mucosal immune system. Invest Ophthalmol Vis Sci 2001 Mar;42(3):566–574.
- Knop N, Knop E. Conjunctiva-associated lymphoid tissue in the human eye. Invest Ophthalmol Vis Sci 2000 May;41(6):1270–1279.
- Steven P, Rupp J, Huttmann G, Experimental induction and three-dimensional two-photon imaging of conjunctiva-associated lymphoid tissue. Invest Ophthalmol Vis Sci 2008 Apr;49(4):1512–1517.
- Steven P, Gebert A. Conjunctiva-associated lymphoid tissue –current knowledge, animal models and experimental prospects. Ophthalmic Res 2009;42(1):2–8.
- Knop E, Knop N. The role of eye-associated lymphoid tissue in corneal immune protection. J Anat 2005 Mar;206(3):271–285.
- Dong Q, Brulc JM, Iovieno A, Diversity of bacteria at healthy human conjunctiva. Invest Ophthalmol Vis Sci 2011 Jul 20;52(8):5408–5413.
- Zhang X, Chen W, de Paiva CS, et al. Desiccating stress induces CD4+ T-cell-mediated Sjogren's syndrome-like corneal epithelial apoptosis via activation of the extrinsic apoptotic pathway by interferon-gamma. Am J Pathol 2011 Oct 17;9(4):1807–1814.
- Pflugfelder SC, de Paiva CS, Villarreal AL, Stern ME. Effects of sequential artificial tear and cyclosporine emulsion therapy on conjunctival goblet cell density and transforming growth factor-beta2 production. Cornea 2008 Jan;27:64–69.
- Zhang X, Chen W, de Paiva CS, Interferon-gamma exacerbates dry eye-induced apoptosis in conjunctiva through dual apoptotic pathways. Invest Ophthalmol Vis Sci 2011 Aug 9;52(9):6279–6285.
- Gupta A, Monroy D, Ji Z, Transforming growth factor beta-1 and beta-2 in human tear fluid. Curr Eye Res 1996;15:605–614.
- Haynes RJ, Tighe PJ, Dua HS. Antimicrobial defensin peptides of the human ocular surface. Br J Ophthalmol 1999 Jun;83:737–741.
- Sobrin L, Liu Z, Monroy DC, Regulation of MMP-9 activity in human tear fluid and corneal epithelial culture supernatant. Invest Ophthalmol Vis Sci 2000 Jun;41(7):1703–1709.
- Govindarajan B, Gipson IK. Membrane-tethered mucins have multiple functions on the ocular surface. Exp Eye Res 2010 Jun;90(6):655–663.
- Spurr-Michaud S, Argueso P, Gipson I. Assay of mucins in human tear fluid. Exp Eye Res 2007 May;84(5):939–950.
- Nava A, Barton K, Monroy DC, Pflugfelder SC. The effects of age, gender, and fluid dynamics on the concentration of tear film epidermal growth factor. Cornea 1997 Jul;16(4):430–438.
- Yamada M, Ogata M, Kawai M, Substance P in human tears. Cornea 2003 Oct;22(7 Suppl):S48–S54.
- Jensen OL, Gluud BS, Birgens HS. The concentration of lactoferrin in tears of normals and of diabetics. Acta Ophthalmol (Copenh) 1986 Feb;64(1):83–87.
- Vinding T, Eriksen JS, Nielsen NV. The concentration of lysozyme and secretory IgA in tears from healthy persons with and without contact lens use. Acta Ophthalmol (Copenh) 1987 Feb;65(1):23–26.
- Zhou L, Huang LQ, Beuerman RW, Proteomic analysis of human tears: defensin expression after ocular surface surgery. J Proteome Res 2004 May;3(3):410–416.
- Sathe S, Sakata M, Beaton AR, Sack RA. Identification, origins and the diurnal role of the principal serine protease inhibitors in human tear fluid. Curr Eye Res 1998 Apr;17(4):348–362.
- Fleming A, Allison V.D. Observations on a bacteriolytic substance (“lysozyme”) found in secretions and tissues. Br J Exp Pathol 1922;3:252–260.
- Carreno E, Enriquez-de-Salamanca A, Teson M, Cytokine and chemokine levels in tears from healthy subjects. Acta Ophthalmol 2010 Nov;88(7):e250–e258.
- Markoulli M, Papas E, Cole N, Holden BA. The diurnal variation of matrix metalloproteinase-9 and its associated factors in human tears. Invest Ophthalmol Vis Sci 2012 Mar 21;53(3):1479–1484.
- Lass JH, Walter EI, Burris TE, Expression of two molecular forms of the complement decay-accelerating factor in the eye and lacrimal gland. Invest Ophthalmol Vis Sci 1990 Jun;31(6):1136–1148.
- Cocuzzi E, Szczotka LB, Brodbeck WG, Tears contain the complement regulator CD59 as well as decay-accelerating factor (DAF). Clin Exp Immunol 2001 Feb;123(2):188–195.
- Shen L, Barabino S, Taylor AW, Dana MR. Effect of the ocular microenvironment in regulating corneal dendritic cell maturation. Arch Ophthalmol 2007 Jul;125(7):908–915.
- Kennedy MC, Rosenbaum JT, Brown J, Novel production of interleukin-1 receptor antagonist peptides in normal human cornea. J Clin Invest. 1995 Jan;95:82–88.
- Solomon A, Dursun D, Liu Z, Pro- and anti-inflammatory forms of interleukin-1 in the tear fluid and conjunctiva of patients with dry-eye disease. Invest Ophthalmol Vis Sci 2001 Sep;42(10):2283–2292.
- Schaumburg CS, Siemasko KF, de Paiva CS, Ocular surface antigen presenting cells are necessary for autoreactive T cell-mediated experimental autoimmune lacrimal keratoconjunctivitis. J Immunol 2011;187(7):3653–3662.
- Bardenstein DS, Dietz Y, Lass JH, Medof ME. Localization of the complement membrane attack complex inhibitor (CD59) in human conjunctiva and lacrimal gland. Curr Eye Res 1994 Dec;13(12):851–855.
- Stern ME, Schaumburg CS, Siemasko KF, Autoantibodies contribute to the immunopathogenesis of experimental dry eye disease. Invest Ophthalmol Vis Sci 2012 Apr 24;53(4):2062–2075.
- Hori J, Wang M, Miyashita M, B7-H1-induced apoptosis as a mechanism of immune privilege of corneal allografts. J Immunol 2006 Nov 1;177:5928–5935.
- Hori J. Role of B7-H1 in immune privilege of the eye. J Nippon Med Sch 2008 Feb;75:56–57.
- Shen L, Jin Y, Freeman GJ, The function of donor versus recipient programmed death-ligand 1 in corneal allograft survival. J Immunol 2007 Sep 15;179:3672–3679.
- El AJ, Goyal S, Zhang Q, et al. Regulation of T-cell chemotaxis by programmed death-ligand 1 (PD-L1) in dry eye-associated corneal inflammation. Invest Ophthalmol Vis Sci 2010 Jul;51(7):3418–3423.
- Stern ME, Gao J, Siemasko KF, et al. The role of the lacrimal functional unit in the pathophysiology of dry eye. Exp Eye Res 2004 Mar;78(3):409–416.
- Thakur A, Willcox MD, Stapleton F. The proinflammatory cytokines and arachidonic acid metabolites in human overnight tears: homeostatic mechanisms. J Clin Immunol 1998 Jan;18(1):61–70.
- Poussier P, Ning T, Banerjee D, Julius M. A unique subset of self-specific intraintestinal T cells maintains gut integrity. J Exp Med 2002 Jun 3;195(11):1491–1497.
- Sacks EH, Wieczorek R, Jakobiec FA, Knowles DM. Lymphocytic subpopulations in the normal human conjunctiva. A monoclonal antibody study. Ophthalmology 1986 Oct;93(10):1276–1283.
- Niederkorn JY, Stern ME, Pflugfelder SC, Desiccating stress induces T cell-mediated Sjogren's syndrome-like lacrimal keratoconjunctivitis. J Immunol 2006 Apr 1;176(7):3950–3957.
- Siemasko KF, Gao J, Calder VL, In vitro expanded CD4+CD25+Foxp3+ regulatory T cells maintain a normal phenotype and suppress immune-mediated ocular surface inflammation. Invest Ophthalmol Vis Sci 2008 Jul 24;49(12):5434–5440.
- Gilbard JP, Farris RL, Santamaria J. Osmolarity of tear microvolumes in keratoconjunctivitis sicca. Arch Ophthalmol 1978 Apr;96(4):677–681.
- Tomlinson A, Khanal S, Ramaesh K, et al. Tear film osmolarity: determination of a referent for dry eye diagnosis. Invest Ophthalmol Vis Sci 2006 Oct;47(10):4309–4315.
- King-Smith PE, Nichols JJ, Nichols KK, Fink BA, Braun RJ. Contributions of evaporation and other mechanisms to tear film thinning and break-up. Optom Vis Sci 2008 Aug;85(8):623–630.
- Stewart P, Chen Z, Farley W, Effect of experimental dry eye on tear sodium concentration in the mouse. Eye Contact Lens 2005 Jul;31(4):175–178.
- Corrales RM, Stern ME, de Paiva CS, Desiccating stress stimulates expression of matrix metalloproteinases by the corneal epithelium. Invest Ophthalmol Vis Sci 2006 Aug;47(8):3293–3302.
- Li DQ, Chen Z, Song XJ, Stimulation of matrix metalloproteinases by hyperosmolarity via a JNK pathway in human corneal epithelial cells. Invest Ophthalmol Vis Sci 2004 Dec;45(12): 4302–4311.
- Luo L, Li DQ, Corrales RM, Pflugfelder SC. Hyperosmolar saline is a proinflammatory stress on the mouse ocular surface. Eye & Contact Lens 2005;31(5):186–193.
- Luo L, Li DQ, Pflugfelder SC. Hyperosmolarity-induced apoptosis in human corneal epithelial cells is mediated by cytochrome c and MAPK pathways. Cornea 2007 May;26:452–460.
- Luo L, Li DQ, Doshi A, Farley W, Experimental dry eye stimulates production of inflammatory cytokines and MMP-9 and activates MAPK signaling pathways on the ocular surface. Invest Ophthalmol Vis Sci 2004 Dec;45(12):4293–4301.
- Li DQ, Luo L, Chen Z, JNK and ERK MAP kinases mediate induction of IL-1beta, TNF-alpha and IL-8 following hyperosmolar stress in human limbal epithelial cells. Exp Eye Res 2006 Apr;82:588–596.
- Yamagami S, Dana MR, Tsuru T. Draining lymph nodes play an essential role in alloimmunity generated in response to high-risk corneal transplantation. Cornea 2002 May;21(4):405–409.
- Johnson GL, Nakamura K. The c-jun kinase/stress-activated pathway: regulation, function and role in human disease. Biochim Biophys Acta 2007 Aug;1773(8):1341–1348.
- Redfern RL, McDermott AM. Toll-like receptors in ocular surface disease. Exp Eye Res 2010 Jun;90(6):679–687.
- Zheng X, Bian F, Ma P, Induction of Th17 differentiation by corneal epithelial-derived cytokines. J Cell Physiol 2010 Jan;222(1):95–102.
- Lee HS, Hattori T, Park EY, Expression of toll-like receptor (TLR) 4 contributes to corneal inflammation in experimental dry eye disease. Invest Ophthalmol Vis Sci 2012 Aug 17;53(9):5632–5640.
- Theofilopoulos AN, Kono DH, Beutler B, Baccala R. Intracellular nucleic acid sensors and autoimmunity. J Interferon Cytokine Res 2011 Dec;31(12):867–886.
- Theofilopoulos AN, Gonzalez-Quintial R, Lawson BR, Sensors of the innate immune system: their link to rheumatic diseases. Nat Rev Rheumatol 2010 Mar;6(3):146–156.
- Yeh S, Song XJ, Farley W, Apoptosis of ocular surface cells in experimentally induced dry eye. Invest Ophthalmol Vis Sci 2003 Jan;44(1):124–129.
- Gao J, Gelber-Schwalb TA, Addeo JV, Stern ME. Apoptosis in the lacrimal gland and conjunctiva of dry eye dogs. Adv Exp Med Biol 1998;438:453–460.
- Reinoso R, Calonge M, Castellanos E, Differential cell proliferation, apoptosis, and immune response in healthy and evaporative-type dry eye conjunctival epithelia. Invest Ophthalmol Vis Sci 2011 Jul 1;52(7):4819–4828.
- Bave U, Nordmark G, Lovgren T, Activation of the type I interferon system in primary Sjogren's syndrome: a possible etiopathogenic mechanism. Arthritis Rheum 2005 Apr;52(4):1185–1195.
- Gottenberg JE, Cagnard N, Lucchesi C, Activation of IFN pathways and plasmacytoid dendritic cell recruitment in target organs of primary Sjogren's syndrome. Proc Natl Acad Sci USA 2006 Feb 21;103(8):2770–2775.
- Higuchi A, Kawakita T, Tsubota K. IL-6 induction in desiccated corneal epithelium in vitro and in vivo. Mol Vis 2011;17:2400–2406.
- Narayanan S, Corrales RM, Farley W, Interleukin-1 receptor-1-deficient mice show attenuated production of ocular surface inflammatory cytokines in experimental dry eye. Cornea 2008 Aug;27(7):811–817.
- Pisella PJ, Brignole F, Debbasch C, Flow cytometric analysis of conjunctival epithelium in ocular rosacea and keratoconjunctivitis sicca. Ophthalmology 2000 Oct;107(10):1841–1849.
- Gao J, Morgan G, Tieu D, ICAM-1 expression predisposes ocular tissues to immune-based inflammation in dry eye patients and Sjogrens syndrome-like MRL/lpr mice. Exp Eye Res 2004 Apr;78(4):823–835.
- Yoon KC, de Paiva CS, Qi H, Expression of th-1 chemokines and chemokine receptors on the ocular surface of C57BL/6 mice: effects of desiccating stress. Invest Ophthalmol Vis Sci 2007 Jun;48(6):2561–2569.
- Yoon KC, Park CS, You IC, Expression of CXCL9, CXCL10, and CXCL11, and CXCR3 in the tear film and ocular surface of patients with dry eye syndrome. Invest Ophthalmol Vis Sci 2009 Oct 22.
- Choi W, Li Z, Oh HJ, Expression of CCR5 and its ligands CCL3, -4, and -5 in the tear film and ocular surface of patients with dry eye disease. Curr Eye Res 2012 Jan;37(1):12–17.
- Enriquez-de-Salamanca A, Castellanos E, Stern ME, Tear cytokine and chemokine analysis and clinical correlations in evaporative-type dry eye disease. Mol Vis 2010 May19;16:862–873.
- Hamrah P, Liu Y, Zhang Q, Dana MR. Alterations in corneal stromal dendritic cell phenotype and distribution in inflammation. Arch Ophthalmol 2003 Aug;121:1132–1140.
- Rashid S, Jin Y, Ecoiffier T, Topical omega-3 and omega-6 fatty acids for treatment of dry eye. Arch Ophthalmol 2008 Feb;126(2):219–225.
- Stern ME, Gao J, Schwalb TA, Conjunctival T-cell subpopulations in Sjogren's and non-Sjogren's patients with dry eye. Invest Ophthalmol Vis Sci 2002 Aug;43(8):2609–2614.
- Chen Y, Chauhan SK, Saban DR, Interferon-{gamma}-secreting NK cells promote induction of dry eye disease. J Leukoc Biol 2011 Mar 14;89(6):965–972.
- Zhang X, Volpe EA, Gandhi NB, NK cells promote Th-17 mediated corneal barrier disruption in dry eye. PLoS One 2012;7(5):e36822.
- Li DQ, Lokeshwar BL, Solomon A, Regulation of MMP-9 production by human corneal epithelial cells. Exp Eye Res 2001 Oct;73(4):449–459.
- Chotikavanich S, de Paiva CS, Li dQ, Production and activity of matrix metalloproteinase-9 on the ocular surface increase in dysfunctional tear syndrome. Invest Ophthalmol Vis Sci 2009 Jul;50(7):3203–3209.
- Okanobo A, Chauhan SK, Dastjerdi MH, Efficacy of topical blockade of interleukin-1 in experimental dry eye disease. Am J Ophthalmol 2012 Jul;154(1):63–71.
- Kim HS, Shang T, Chen Z, TGF-beta1 stimulates production of gelatinase (MMP-9), collagenases (MMP-1, -13) and stromelysins (MMP-3, -10, -11) by human corneal epithelial cells. Exp Eye Res 2004 Aug;79(2):263–274.
- Kim HS, Luo L, Pflugfelder SC, Li DQ. Doxycycline inhibits TGF-{beta}1-induced MMP-9 via Smad and MAPK pathways in human corneal epithelial cells. Invest Ophthalmol Vis Sci 2005 Mar;46(3):840–848.
- Zheng X, de Paiva CS, Rao K, Evaluation of the transforming growth factor-beta activity in normal and dry eye human tears by CCL-185 cell bioassay. Cornea 2010 Sep;29(9):1048–1054.
- Barabino S, Montaldo E, Solignani F, et al. Immune response in the conjunctival epithelium of patients with dry eye. Exp Eye Res 2010 Oct;91(4):524–529.
- Chan JH, Amankwah R, Robins RA, et al. Kinetics of immune cell migration at the human ocular surface. Br J Ophthalmol 2008 Jul;92:970–975.
- Jameson JM, Sharp LL, Witherden DA, Havran WL. Regulation of skin cell homeostasis by gamma delta T cells. Front Biosci 2004 Sep 1;9:2640–2651.
- Sutton CE, Lalor SJ, Sweeney CM, Interleukin-1 and IL-23 induce innate IL-17 production from gammadelta T cells, amplifying Th17 responses and autoimmunity. Immunity 2009 Aug 21;31(2):331–341.
- Roark CL, French JD, Taylor MA, Exacerbation of collagen-induced arthritis by oligoclonal, IL-17-producing gamma delta T cells. J Immunol 2007 Oct 15;179(8):5576–5583.
- Cui Y, Shao H, Lan C, Major role of gamma delta T cells in the generation of IL-17+ uveitogenic T cells. J Immunol 2009 Jul 1;183(1):560–567.
- Bialasiewicz AA, Schaudig U, Ma JX, Alpha/beta- and gamma/delta-T-cell-receptor-positive lymphocytes in healthy and inflamed human conjunctiva. Graefes Arch Clin Exp Ophthalmol 1996 Jul;234(7):467–471.
- Soukiasian SH, Rice B, Foster CS, Lee SJ. The T cell receptor in normal and inflamed human conjunctiva. Invest Ophthalmol Vis Sci 1992 Feb;33(2):453–459.
- Uhlig HH, McKenzie BS, Hue S, Differential activity of IL-12 and IL-23 in mucosal and systemic innate immune pathology. Immunity 2006 Aug;25(2):309–318.
- Chen Y, Chauhan SK, Saban DR, Interferon-gamma-secreting NK cells promote induction of dry eye disease. J Leukoc Biol 2011 Jun;89(6):965–972.
- de Paiva CS, Villarreal AL, Corrales RM, IFN- g promotes goblet cell loss in response to desiccating ocular stress. Invest Ophthalmol Vis Sci 2006;47:E-Abstract 5579.
- de Paiva CS, Villarreal AL, Corrales RM, Dry eye-induced conjunctival epithelial squamous metaplasia is modulated by interferon-{gamma}. Invest Ophthalmol Vis Sci 2007 Jun;48(6):2553–2560.
- Albertsmeyer AC, Kakkassery V, Spurr-Michaud S, Effect of pro-inflammatory mediators on membrane-associated mucins expressed by human ocular surface epithelial cells. Exp Eye Res 2010 Mar;90(3):444–451.
- de Paiva CS, Chotikavanich S, Pangelinan SB, IL-17 disrupts corneal barrier following desiccating stress. Mucosal Immunol 2009 May;2(3):243–253.
- de Paiva CS, Raince JK, McClellan AJ, Homeostatic control of conjunctival mucosal goblet cells by NKT-derived IL-13. Mucosal Immunol 2011 Jul;4(4):397–408.
- Goyal S, Chauhan SK, Zhang Q, Dana R. Amelioration of murine dry eye disease by topical antagonist to chemokine receptor 2. Arch Ophthalmol 2009 Jul;127(7):882–887.
- Steinman RM, Hemmi H. Dendritic cells: translating innate to adaptive immunity. Curr Top Microbiol Immunol 2006;311:17–58.
- Ueno H, Klechevsky E, Morita R, Dendritic cell subsets in health and disease. Immunol Rev 2007 Oct;219:118–142.
- Dursun D, Wang M, Monroy D, A mouse model of keratoconjunctivitis sicca. Invest Ophthalmol Vis Sci 2002 Mar;43(3):632–638.
- Hamrah P, Liu Y, Zhang Q, Dana MR. The corneal stroma is endowed with a significant number of resident dendritic cells. Invest Ophthalmol Vis Sci 2003 Feb;44:581–589.
- Jin Y, Shen L, Chong EM, The chemokine receptor CCR7 mediates corneal antigen-presenting cell trafficking. Mol Vis 2007;13:626–634.
- Dana R. Corneal antigen presentation: molecular regulation and functional implications. Ocul Surf 2005 Oct;3(4 Suppl):S169–S172.
- Goyal S, Chauhan SK, El AJ, Evidence of corneal lymphangiogenesis in dry eye disease: a potential link to adaptive immunity? Arch Ophthalmol 2010 Jul;128(7):819–824.
- van RN, Hendrikx E. Liposomes for specific depletion of macrophages from organs and tissues. Methods Mol Biol 2010;605:189–203.
- Pflugfelder SC, de Paiva CS, Li DQ, Stern ME. Epithelial-immune cell interaction in dry eye. Cornea 2008 Sep;27 (Suppl 1):S9–S11.
- Kunert KS, Tisdale AS, Gipson IK. Goblet cell numbers and epithelial proliferation in the conjunctiva of patients with dry eye syndrome treated with cyclosporine. Arch Ophthalmol 2002 Mar;120(3):330–337.
- Ecoiffier T, El AJ, Rashid S, Modulation of integrin alpha4beta1 (VLA-4) in dry eye disease. Arch Ophthalmol 2008 Dec;126(12):1695–1699.
- Chauhan SK, El AJ, Ecoiffier T, Autoimmunity in dry eye is due to resistance of Th17 to Treg suppression. J Immunol 2009 Feb 1;182(3):1247–1252.
- Reinoso R, Calonge M, Castellanos E, Differential cell proliferation, apoptosis, and immune response in healthy and evaporative-type dry eye conjunctival epithelia. Invest Ophthalmol Vis Sci 2011 Jul 1;52(7):4819–4828.
- El AJ, Chauhan SK, Ecoiffier T, et al. Characterization of effector T cells in dry eye disease. Invest Ophthalmol Vis Sci 2009 Aug;50(8):3802–3807.
- Zhou L, Chong MM, Littman DR. Plasticity of CD4+ T cell lineage differentiation. Immunity 2009 May;30(5):646–655.
- Corrales RM, Villarreal A, Farley W, et al. Strain-related cytokine profiles on the murine ocular surface in response to desiccating stress. Cornea 2007 Jun;26(5):579–584.
- Enriquez-de-Salamanca A, Calder V, Gao J, Galatowicz G, Cytokine responses by conjunctival epithelial cells: an in vitro model of ocular inflammation. Cytokine 2008 Oct;44(1):160–167.
- Massingale ML, Li X, Vallabhajosyula M, Analysis of inflammatory cytokines in the tears of dry eye patients. Cornea 2009 Oct;28(9):1023–1027.
- Pflugfelder SC, Farley W, Luo L, Matrix metalloproteinase-9 knockout confers resistance to corneal epithelial barrier disruption in experimental dry eye. Am J Pathol 2005 Jan;166(1):61–71.
- Katsifis GE, Rekka S, Moutsopoulos NM, Systemic and local interleukin-17 and linked cytokines associated with Sjogren's syndrome immunopathogenesis. Am J Pathol 2009 Sep;175(3):1167–1177.
- Hirota K, Yoshitomi H, Hashimoto M, Preferential recruitment of CCR6-expressing Th17 cells to inflamed joints via CCL20 in rheumatoid arthritis and its animal model. J Exp Med 2007 Nov 26;204(12):2803–2812.
- Humphreys-Beher MG, Brinkley L, Purushotham KR, Characterization of antinuclear autoantibodies present in the serum from nonobese diabetic (NOD) mice. Clin Immunol Immunopathol 1993 Sep;68(3):350–356.
- Yamamoto H, Sims NE, Macauley SP, Alterations in the secretory response of non-obese diabetic (NOD) mice to muscarinic receptor stimulation. Clin Immunol Immunopathol 1996 Mar;78(3):245–255.
- Kovacs L, Marczinovits I, Gyorgy A, Clinical associations of autoantibodies to human muscarinic acetylcholine receptor 3(213-228) in primary Sjogren's syndrome. Rheumatology (Oxford) 2005 Aug;44(8):1021–1025.
- Bacman S, Berra A, Sterin-Borda L, Borda E. Muscarinic acetylcholine receptor antibodies as a new marker of dry eye Sjogren syndrome. Invest Ophthalmol Vis Sci 2001 Feb;42(2):321–327.
- Bacman S, Sterin-Borda L, Camusso JJ, Circulating antibodies against rat parotid gland M3 muscarinic receptors in primary Sjogren's syndrome. Clin Exp Immunol 1996 Jun;104(3): 454–459.
- Borda E, Camusso JJ, Perez LC, Circulating antibodies against neonatal cardiac muscarinic acetylcholine receptor in patients with Sjogren's syndrome. Mol Cell Biochem 1996 Oct;163–164:335–341.
- Nguyen KH, Brayer J, Cha S, Evidence for antimuscarinic acetylcholine receptor antibody-mediated secretory dysfunction in nod mice. Arthritis Rheum 2000 Oct;43(10):2297–2306.
- Robinson CP, Brayer J, Yamachika S, Transfer of human serum IgG to nonobese diabetic Igmu null mice reveals a role for autoantibodies in the loss of secretory function of exocrine tissues in Sjogren's syndrome. Proc Natl Acad Sci U S A 1998 Jun 23;95(13):7538–7543.
- Rose CM, Qian L, Hakim L, Accumulation of catalytically active proteases in lacrimal gland acinar cell endosomes during chronic ex vivo muscarinic receptor stimulation. Scand J Immunol 2005 Jan;61(1):36–50.
- Jiang G, Ke Y, Sun D, A new model of experimental autoimmune keratoconjunctivitis sicca (KCS) induced in Lewis rat by the autoantigen Klk1b22. Invest Ophthalmol Vis Sci 2009 May;50(5):2245–2254.
- Takada K, Takiguchi M, Konno A, Inaba M. Autoimmunity against a tissue kallikrein in IQI/Jic Mice: a model for Sjogren's syndrome. J Biol Chem 2005 Feb 4;280:3982–3988.
- Chan OT, Hannum LG, Haberman AM, A novel mouse with B cells but lacking serum antibody reveals an antibody-independent role for B cells in murine lupus. J Exp Med 1999 May 17;189(10):1639–1648.
- Rodriguez-Pinto D. B cells as antigen presenting cells. Cell Immunol 2005 Dec;238(2):67–75.
- Serreze DV, Fleming SA, Chapman HD, B lymphocytes are critical antigen-presenting cells for the initiation of T cell-mediated autoimmune diabetes in nonobese diabetic mice. J Immunol 1998 Oct 15;161(8):3912–3918.
- O'Neill SK, Shlomchik MJ, Glant TT, Antigen-specific B cells are required as APCs and autoantibody-producing cells for induction of severe autoimmune arthritis. J Immunol 2005 Mar 15;174(6):3781–3788.
- Lund FE. Cytokine-producing B lymphocytes-key regulators of immunity. Curr Opin Immunol 2008 Jun;20(3):332–338.
- Manz RA, Arce S, Cassese G, Humoral immunity and long-lived plasma cells. Curr Opin Immunol 2002 Aug;14(4):517–521.
- Daha NA, Banda NK, Roos A, Complement activation by (auto-) antibodies. Mol Immunol 2011 Aug;48(14):1656–1665.
- Van den Berg CW, Aerts PC, Van DH. In vivo anti-complementary activities of the cobra venom factors from Naja naja and Naja haje. J Immunol Methods 1991 Feb 15;136(2):287–294.
- Cochrane CG, Muller-Eberhard HJ, Aikin BS. Depletion of plasma complement in vivo by a protein of cobra venom: its effect on various immunologic reactions. J Immunol 1970 Jul;105(1):55–69.
- Vogel CW, Fritzinger DC, Hew BE, Recombinant cobra venom factor. Mol Immunol 2004 Jun;41(2–3):191–199.
- Management and therapy of dry eye disease: report of the Management and Therapy Subcommittee of the International Dry Eye WorkShop (2007). Ocul Surf 2007 Apr;5(2):163–178.
- Sall K, Stevenson OD, Mundorf TK, Reis BL. Two multicenter, randomized studies of the efficacy and safety of cyclosporine ophthalmic emulsion in moderate to severe dry eye disease. CsA Phase 3 Study Group. Ophthalmology 2000 Apr;107(4):631–639.
- Turner K, Pflugfelder SC, Ji Z, et al. Interleukin-6 levels in the conjunctival epithelium of patients with dry eye disease treated with cyclosporine ophthalmic emulsion. Cornea 2000 Jul;19(4):492–496.
- Baudouin C, Brignole F, Pisella PJ, Flow cytometric analysis of the inflammatory marker HLA DR in dry eye syndrome: results from 12 months of randomized treatment with topical cyclosporin A. Adv Exp Med Biol 2002;506(Pt B):761–769.
- Semba CP, Torkildsen GL, Lonsdale JD, A phase 2 randomized, double-masked, placebo-controlled study of a novel integrin antagonist (SAR 1118) for the treatment of dry eye. Am J Ophthalmol 2012 Jun;153(6):1050–1060.
- Nava A, Barton K, Monroy DC, Pflugfelder SC. The effects of age, gender, and fluid dynamics on the concentration of tear film epidermal growth factor. Cornea 1997 Jul;16(4):430–438.
- Ambati BK, Nozaki M, Singh N, Corneal avascularity is due to soluble VEGF receptor-1. Nature 2006 Oct 26;443(7114):993–997.
- Cursiefen C, Chen L, Saint-Geniez M, Nonvascular VEGF receptor 3 expression by corneal epithelium maintains avascularity and vision. Proc Natl Acad Sci U S A 2006 Jul 25;103(30):11405–11410.