Abstract
Particulates and crystals stimulate the immune system to induce inflammatory responses. Several nanometer- to micrometer-sized particulates, such as particle matter 2.5 (PM2.5), diesel particles, and sand dust, induce pulmonary inflammation and allergic asthma. Conversely, nanometer- to micrometer-sized crystal, sphere, and hydrogel forms of aluminum salts (referred to as “alum”) have been used as vaccine adjuvants to enhance antibody responses in animals and humans. Although most of these particulates induce type-2 immune responses in vivo, the molecular and immunological mechanisms of action as a vaccine adjuvant are poorly understood. In this review, recent advances in particulate adjuvant research from the standpoint of innate immune responses are discussed.
INTRODUCTION: ADJUVANT AND INNATE IMMUNITY
Immune responses are categorized into two types: innate and adaptive. Innate immunity is mediated by macrophages and dendritic cells (DCs), which engulf and kill microbes. In contrast, adaptive immunity involves antigen-specific responses mediated by T cells, B cells, and memory cells. It had long been believed that the innate immune response functions as a temporal defense system against infection until the adaptive immune response can be elicited. However, recent studies have demonstrated that innate immunity is essential for the effective induction of adaptive immunity [Citation1–3].
Vaccination mimics natural infection and induces pathogen-specific adaptive immunity effectively. Typically, vaccines contain two main components: antigens and adjuvants. An adjuvant is a substance that enhances antigen-specific (adaptive) immune responses when used in combination with a specific antigen. An adjuvant is thought to be an activator of innate immunity. In general, innate immune cells recognize pathogen-derived factors [e.g. pathogen-associated molecular patterns (PAMPs)], through pattern recognition receptors (PRRs) and induce inflammatory responses. There are four classes of PRRs: Toll-like receptors (TLRs), Nod-like receptors (NLRs), RIG-I-like receptors (RLRs), and C-type lectin receptors (CLRs) [Citation4–7]. These receptors “sense” pathogen-derived factors and transduce activating signals into cells, triggering adaptive immunity against pathogens. Therefore, the ligands for PRRs, such as PAMPs and damage-associated molecular patterns (DAMPs), exhibit potent adjuvant properties that elicit adaptive immunity, and PRRs are considered to be receptors for adjuvants [Citation1, Citation8].
However, the molecular and immunological mechanisms of many adjuvants used clinically (or those under development) have yet to be fully elucidated. For example, oil emulsions (e.g. Freund's adjuvant and MF-59) and saponin-based adjuvants (e.g., QS-21 and ISCOM) exhibit strong adjuvant activities and could be promising candidates as adjuvants for new human vaccines [Citation9, 10], yet no specific PRR(s) is identified.
An increasing number of particulates and nanoparticles have been reported to exhibit adjuvant activity. A well-known and widely used particulate adjuvant is aluminum salts, which is referred to as “alum” [Citation11–13]. The mechanisms of induction of adaptive immunity by alum or a particulate adjuvant are also unclear, even though alum has been used as a human vaccine adjuvant for more than 80 years. The induction of adaptive immunity requires innate immunity. Hence, it has been proposed that particulates can activate innate cells, and that this action is accompanied by the induction of cytokines, chemokines, and other factors.
PARTICULATES AND THE ADJUVANT EFFECT
Several particulates are known to exhibit adjuvant effects in immune responses. Alum selectively stimulates humoral immune responses, especially type-2 helper (Th2) immune responses, which are characterized by the production of interleukin (IL)-4 and IL-5 and the induction of immunoglobulin (Ig) E and IgG1 [Citation11–13]. (In the case of mice, IgG1 is categorized into Th2-dependent antibody, but the IgG isotype for human Th2 responses has not been clarified fully.) Similar to alum, crystalline silica (which causes a type of pulmonary fibrosis referred to as “silicosis”) induces Th2 responses and antigen-specific IgE and IgG1 [Citation14]. It has been reported that synthesized particles, such as poly(lactic-co-glycolic acid) (PGLA), polystyrene particles, nickel oxide nanoparticles, and carbon nanotubes, induce humoral immunity, especially antigen-specific production of IgG1 and IgE [Citation15–19]. Several particulate pollutants, such as diesel exhaust particles, have been shown to induce Th2 responses after intratracheal instillation and are thought to be the source of allergic diseases [Citation20, 21]. In addition to artificial particulates, several crystals generated in the body induce inflammatory responses and possess adjuvant activity. Monosodium urate (MSU) crystals are formed if the concentration of uric acid released from damaged cells reaches saturation. MSU crystals act as DAMPs, and are the causative agent of gout. MSU crystals also act as Th2 adjuvants [Citation22–26]. The biocrystalline substance hemozoin is a hemin detoxification byproduct of malaria parasites. Hemozoin exhibits a potent adjuvant effect and induces humoral immune responses [Citation27]. Chitin particles, which are biopolymers of N-acetyl-D-glucosamine found in fungi, helminthes, and insects, induce the accumulation of IL-4-producing eosinophils and basophils, and are associated with allergy [Citation28]. In contrast to PAMPs such as lipopolysaccharide (LPS) and CpG oligodeoxynucleotides, almost all particulates preferentially elicit Th2 responses and the induction of IgE. Therefore, it has been hypothesized that the specific signals evoked by particulates in innate cells are involved in triggering adaptive (Th2) responses.
PARTICLE SIZE AND IMMUNE RESPONSES
Particle size is thought to affect particulate-induced immune responses. Sharp et al. investigated the relationship between particle size and DC activation. They showed that the polystyrene particles measuring between 430 nm and 1 μm activated DCs efficiently to produce IL-1β [Citation15]. Hornung et al. demonstrated that the optimal size of silica crystals engulfed by macrophages was approximately 1 μm [Citation29]. Coban et al. investigated the adjuvanticity of hemozoin of different sizes. They reported that hemozoin particles measuring between 50 nm and 200 nm exhibited a stronger adjuvant effect compared with larger (2–20 μm) and smaller (<50 nm) particles [Citation27, Citation30]. These results suggest that particles measuring between 200 nm and 1 μm are the optimal size for phagocytosis and the stimulation of immune responses.
DEPOT EFFECT
Antigen persistence and prolonged release, an effect referred to as the “depot effect” (first proposed by Glenny et al. in 1926), is believed to be responsible for the adjuvanticity of alum [Citation31]. Harrison verified this hypothesis in 1935 by transferring the alum nodules from one guinea pig into a second guinea pig [Citation32]. However, the depot effect has been questioned in several reports. Holt demonstrated that the antibody responses were normal if alum nodules were excised 2 weeks after immunization [Citation33]. In particular, a recent report by Hutchison et al. demonstrated that the removal of the injection site 2 hours after the administration of antigen/alum had no effect on antigen-specific antibody and T-cell responses [Citation34]. These studies suggest that the antigen depot does not play an important part in alum adjuvanticity, and that alum exhibits additional effects that account for its adjuvant properties.
TH2 CYTOKINES AND IL-4-PRODUCING CELLS
Alum preferentially induces Th2 responses (which are characterized by the production of IgG1 and IgE) and IL-4 is a crucial factor for the induction of such Th2 responses. Alum and several other particulates induce the recruitment of IL-4-producing myeloid cells. Jordan et al. reported that alum induces IL-4-producing Gr-1+ cells, and that these cells and IL-4 are required for the expansion of antigen-specific B cells in vivo [Citation35]. Furthermore, Wang et al. demonstrated that alum-elicited Gr-1+ cells are IL-4-producing eosinophils [Citation36]. As stated above, chitin-induced IL-4-producing cells were eosinophils and basophils. Moreover, eosinophil recruitment is dependent on the leukotriene B4 produced by macrophages [Citation28]. However, it has been reported that the antigen-specific antibody responses are normal in several eosinophil-deficient mice (IL-5-deficient, GATA1Δ, and Phil mice) compared with wild-type (WT) control mice after immunization with ovalbumin (OVA)-alum [Citation37]. In addition, Ohnmacht el al. demonstrated that antigen-specific IgG1 and IgE responses were comparable in WT and basophil-deficient mice immunized with OVA and alum [Citation38]. These studies suggest that IL-4-producing myeloid cells such as eosinophils and basophils do not participate in alum adjuvanticity or Th2 responses. Recently, it has been reported that CD1d-deficient [both type-I and -II natural killer T (NKT) cell-deficient]-mice, but not Jα18-deficient (only type-I NKT cell-deficient)-mice exhibited reduced levels of antigen-specific IgG1 [Citation39]. Type-II NKT cells appear to be required for alum-induced antigen-specific IgG1 responses in the regulation of IL-4-producing T cells.
There are several reports on IL-4 signaling and alum adjuvanticity [Citation40, 41]. Brewer et al. reported on the involvement of IL-4 in the immunization of alum using IL-4-, IL-4Rα-, and STAT6-deficient mice. These strains of mice did not induce the production of IgE and exhibited reduced levels of IgG1. However, T cells from IL-4Rα- and STAT6-deficient mice produced normal or higher amounts of IL-4 and IL-5 in response to a specific antigen. These results indicate that IL-4- and IL-13-mediated signaling is required for Th2-associated antibody production but is dispensable for alum-induced Th2 responses.
Recently, several reports focused on the importance of thymic stromal lymphopoietin (TSLP) on Th2 activation, and Al-Shami et al. demonstrated that TSLP receptor-deficient mice displayed reduced Th2 responses after immunization with OVA and alum [Citation42]. However, allergen (without adjuvant)-induced Th2 responses were also reduced in TSLP receptor-deficient or anti-TSLP antibody-treated mice [Citation43, 44]. These results indicate that TSLP receptor-deficient mice are Th1 prone, and that reduced Th2 responses are not specific to immunization with alum.
PARTICULATES AND MYD88 SIGNALING
All TLR ligands are thought to be potent immune adjuvants through the activation of the adaptor molecules MyD88 and TRIF. Schnare et al. demonstrated that MyD88-deficient mice produced normal levels of OVA-specific IgG and IgE, but that elevated levels of total IgE were produced after immunization with OVA in alum [Citation45]. The excessive amounts of total IgE appeared to be caused by the increased production of IL-13 in MyD88-deficient T cells. Gavin et al. also reported alum adjuvanticity in mice deficient in MyD88 and TRIF, which lack TLR signaling. The antibody responses in these double-knockout (KO) mice were comparable with those in WT mice immunized with trinitrophenol (TNP)-hemocyanin in alum [Citation46]. These results suggest that TLR signaling does not account for the action of alum and indicate that TLRs may acts as negative regulators of IgE production. However, Da Silva et al. demonstrated that MyD88 pathway was required for alum-induced Th2 responses in asthma models [Citation47]. The reason for these discrepant results is unclear. There might be differences in the alum (Imject alum, aluminum hydroxide, aluminum phosphate, or aluminum potassium sulfate) and OVA (endotoxin-free or not) used.
Conversely, hemozoin crystals seem to act as MyD88-dependent adjuvants in natural and synthetic forms [Citation27, Citation30]. The mechanism(s) underlying this dissimilarity between alum and hemozoin particulates remains to be investigated.
NLRP3 INFLAMMASOME
In 2008, several reports focused on the discovery that particulate adjuvants activate the NLRP3 inflammasome [Citation29, Citation48]. The inflammasome is a PRR, and there are four classes of inflammasome: NLRP1, NLRP3, NLRC4, and AIM2 [Citation5]. The NLRP3 inflammasome is one of the best characterized inflammasomes and is activated by particulates and crystals [Citation15, Citation29, Citation48–53]. NLRP3 forms a multiprotein complex with apoptosis-associated speck-like protein containing a caspase recruitment domain (ASC) and caspase-1. The NLRP3 inflammasome promotes the secretion of inflammatory cytokines such as IL-1β and IL-18 as active mature forms cleaved by activated caspase-1. In addition to activation by PAMPs, several reports have demonstrated that particulates such as silica and alum stimulate macrophages and DCs to produce IL-1β and IL-18 through activation of the inflammasome, and that alum-induced antigen-specific IgG1 responses are significantly reduced in NLRP3-, ASC-, and caspase-1-deficient mice [Citation48, Citation54]. Similar to alum, most particulate adjuvants are considered to have an adjuvant effect via inflammasome activation because silica, asbestos, PLGA, and MSU act as activators of the NLRP3 inflammasome. However, other reports have shown that the NLRP3 inflammasome is not required for antibody production in response to vaccination using a particulate adjuvant, including alum [Citation27, Citation37, Citation55, 56]. These contradictory reports on the role of the NLRP3 inflammasome may be because of different experimental conditions. Several studies used Imject alum [Citation48, Citation54, Citation55], whereas other studies used aluminum hydroxide [Citation56]. Differences in genetic background of the animal used, such as C57BL/6 [Citation48, Citation54, Citation55] and mixed C57BL/6–129 [Citation56], might contribute to the contrasting results. The involvement of inflammasome-dependent cytokines in alum adjuvanticity is an important issue. It has been demonstrated that IL-18 plays an important part in alum-mediated Th2 responses [Citation57]. However, IL-1 and IL-18 signaling triggers MyD88-dependent signaling, and MyD88 signaling is dispensable for alum adjuvanticity (as described above). The NLRP3 inflammasome may participate in adjuvant activity through IL-1β- and IL-18-independent mechanisms, but the role of the NLRP3 inflammasome in the induction of adjuvant activity remains unclear ().
FIGURE 1. Proposed mechanisms of particulate adjuvants (alum, MSU, silica) in innate immunity. Alum induces cell death, and the damaged host cells, such as macrophages and neutrophils, release genomic DNA and uric acid as DAMPs. The recognition mechanisms of genomic DNA are still unclear, but the TBK-1-IRF3 axis plays an important part in IgE production and iMono/iDC migration via the IL-12p80 production. The released uric acid forms MSU crystals, which are recognized by lipid sorting on DCs. The engulfed MSU crystals trigger the activation of Syk and PI3Kδ, and induce inflammatory cells or a strong interaction between DCs and CD4+ T cells. However, the released uric acid has not been shown to form crystals at the site of alum injection. Alum and silica stimulate macrophages and DCs to produce NLRP3 inflammasome-dependent IL-1β and IL-18. These cytokines contribute (at least in part) to acute inflammation and Th2 activation. Macrophages and DCs also induce PGE2 in response to alum and silica via Syk activation. PGE2 is involved in IgE production. iMonos: inflammatory monocytes; iDCs: inflammatory DCs.
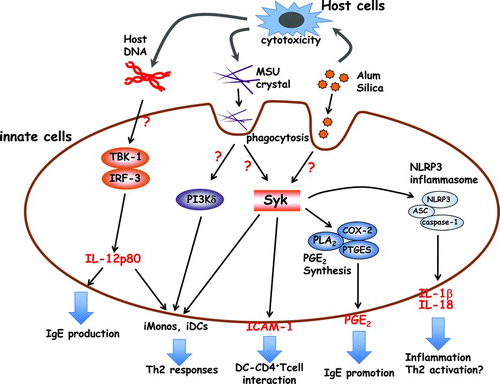
MSU AS A DAMP
Uric acid is a purine catabolite that is released from dying or stressed cells. Uric acid forms MSU crystals if the concentration of uric acid is saturated. Shi et al. demonstrated that uric acid and MSU crystals act as DAMPs and stimulate DCs to induce the maturation and activation of cells [Citation58]. Interestingly, similar to alum, MSU crystals are known to activate Th2 responses preferentially [Citation22–26]. Kool et al. demonstrated that uric acid is released in the peritoneal cavity after the injection of alum, and that antigen-specific T-cell responses were prevented after uricase treatment [Citation22]. Alum is known to induce cell death, and uric acid and MSU crystals induced by alum cytotoxicity appear to contribute to alum adjuvanticity (whether uric acid forms crystal in vivo in alum-injected sites is of considerable interest). In addition, this study demonstrated that uric acid-primed inflammatory monocytes and DCs have an important role in the activation of antigen-specific T cells [Citation22]. However, this study reported that MyD88 signaling was required for this mechanism, which is a controversial proposal. Similar to alum and silica, MSU crystals have been reported to activate the NLRP3 inflammasome [Citation50], and this finding is suspected to be linked to the adjuvant activity through the activation of the NLRP3 inflammasome. However, it has been reported that IL-1β, MyD88, and the NLRP3 inflammasome are dispensable for uric acid-dependent adjuvant activity, and that spleen tyrosine kinase (Syk) and PI3-kinase δ in inflammatory monocytes and DCs are required for Th2 activation by uric acid () [Citation25]. Syk is a nonreceptor tyrosine kinase and a key mediator of immunoreceptor signaling in immune cells. It has been demonstrated that Syk is involved in particulate-mediated innate cell activation [Citation17, Citation51, Citation59]. The relationship between uric acid-induced Th2 activation and Syk is interesting. Although Syk is known to be activated by immunoreceptor tyrosine-based activation motif (ITAM)-bearing receptors [Citation60], the underlying mechanisms of Syk activation by particulates is unclear.
Recently, several studies demonstrated the unique recognition mechanisms of particulates. Ng et al. analyzed the recognition of MSU crystals by DCs using atomic force microscopy. MSU crystals were shown to interact with DCs via receptor-independent mechanisms by directly engaging cell surface lipids (mainly cholesterol) [Citation61]. The aggregation of lipid rafts triggers the recruitment and activation of Syk, and ultimately, Syk activates PI3-kinase, phagocytosis, and cytokine secretion () [Citation61]. Flach et al. reported that alum also binds to the surface of DCs, leading to lipid sorting that is similar to MSU crystal-mediated activation of Syk and PI3-kinase. However, the uptake of alum is not required, and activated DCs interact with DC4+ T cells via binding with intracellur adhesion molecule (ICAM)-1 and leukocyte function-associated antigen (LFA)-1 () [Citation62]. Syk appears to be a key molecule for the activation of DCs via lipid sorting, but the mechanisms of Syk activation by MSU or alum are unclear. In general, Syk is known to be activated by Src family kinases such as Hck, Fgr, and Lyn, and ITAM-containing FcRγ and DNAX-activating protein of 12 kDa (DAP12). However, DCs double-deficient in ITAM-containing FcRγ and DAP12 or triple-deficient in Src family kinases (Hck−/−, Fgr−/−, and Lyn−/−) retain their function after activation by MSU crystals [Citation61].
LIPID MEDIATOR
Recently, we found that Th2-inducing particulate adjuvants have another unique mechanism for the activation of innate immune cells: alum and silica particulates stimulate macrophages to produce prostaglandins (PGs) in a similar way to the secretion of IL-1β and IL-18 via NLRP3 activation [Citation17]. In addition to proinflammatory cytokines, lipid mediators such as PGs are involved in the induction of inflammatory responses. The well-characterized proinflammatory lipid mediator PGE2 is a metabolite of arachidonic acid that is produced by various types of cells, including antigen-presenting cells [Citation63]. Studies have shown that PGE2 suppresses Th1 responses by elevating intracellular concentrations of cyclic adenosine monophosphate (cAMP) in DCs and macrophages, thereby inhibiting the production of IL-12 and interferon [Citation64–66]. In addition, PGE2 enhances IL-23 production by DCs and favors Th17 polarization [Citation67, 68]. More recently, PGE2 has been shown to facilitate the differentiation of Th1 cells in the presence of IL-12 and high doses of the co-stimulatory CD28 antibody via the activation of the PI3-kinase pathway [Citation68]. Thus, PGE2 exhibits various functions in the regulation of immune responses.
Silica and alum stimulate macrophages and DCs to produce IL-1β, IL-18, and PGE2. The PGE2 production induced by silica and alum has been shown to be independent of the NLRP3 inflammasome because inflammasome-deficient (NLRP3−/−, ASC−/−, caspase-1−/−) macrophages produced normal levels of PGE2 in response to silica and alum compared with WT counterparts. Treatment with a Syk inhibitor or the knockdown of Syk using small interfering RNA (siRNA) molecules markedly suppressed the production of PGE2 after stimulation with silica and alum, demonstrating that Syk regulates particulate-induced PGE2 production. In this case, the mechanisms of Syk activation by alum and silica are unclear. However, several reports (including those involving studies on MSU crystals) have demonstrated that particulates stimulate innate immune cells via Syk activation. Therefore, Syk may be a key molecule for particulate-induced immune responses (). PGE2 synthesis is regulated by cyclooxygenase (COX) and PGE synthase (PTGES), and COX-2 and PTGES (also known as mPGES-1) in particular have been reported to regulate stimulation-dependent PGE2 production in macrophages [Citation69]. PTGES-deficient macrophages do not produce detectable amounts of PGE2 after stimulation with silica or alum. In addition, PTGES-deficient mice display reduced amounts of antigen-specific IgE after immunization with alum and silica. In contrast, the levels of antigen-specific IgG are normal in PTGES-deficient mice compared with WT mice. These results indicate that particulate-induced PGE2 is involved in IgE production in vivo () [Citation17]. Several reports have demonstrated that PGE2 facilitates IgE production by the accumulation of increased levels of intracellular cAMP [Citation70, 71]. Interestingly, neuropeptides such as vasoreactive intestinal polypeptide (VIP) and pituitary adenylate cyclase-activating polypeptide (PACAP) preferentially activate Th2 responses and increase intracellular cAMP levels in a receptor-dependent manner [Citation72, 73]. In addition, the soluble extract of birch pollen consists of a lipid that is thought to be the causative agent of allergic asthma and to resemble the structure and function of PGE2. This lipid induces Th2 responses and intracellular cAMP accumulation in DCs [Citation74, 75]. Agents for cAMP elevation may act as Th2 adjuvants.
Many particulates that exhibit adjuvant activity, such as MSU crystals, PLGA, chitin particles, nickel oxide, amorphous silica, and carbon nanotubes, stimulate macrophages to produce inflammasome-dependent IL-1β and inflammasome-independent PGE2. In addition, we have found that, similar to the release of uric acid, increased amounts of PGE2 are released from damaged cells, suggesting that PGE2 also works as a DAMP (Kuroda et al., unpublished data). These findings suggest that PGE2 is a useful marker for the screening of particulate (Th2) adjuvants.
TABLE 1. Summary of the effect of particulate (alum) adjuvant on immune system
RELEASE OF NUCLEIC ACIDS FROM HOST CELLS
Activation of innate immunity by DAMPs appears to be a critical mechanism for adjuvant activity. Recently, it was reported that the DNA released from host cells mediates the adjuvant activity of alum [Citation76]. In this study, alum induced the local accumulation of host DNA at the injection site during alum-induced cell death, and interestingly, treatment with DNase I decreased the antigen-specific antibody responses in mice immunized with OVA in alum. Purified genomic DNA mixed with OVA induced OVA-specific IgG1 and IgE responses as efficiently as the alum adjuvant. These results indicate that the alum-induced release of host DNA triggers initial innate immune responses. These responses are not dependent on TLRs, RLRs, or inflammasomes, and the mechanisms by which the host DNA triggers the immune response are unclear. However, interferon regulatory factor 3 (IRF3) and TANK-binding kinase 1 (TBK1) are required for the adjuvant activity of alum (). It has been reported that antigen-specific IgE responses, but not IgG1 responses, are significantly reduced in IRF3-deficient and TBK1/tumor necrosis factor (TNF)-double-deficient mice. Inflammatory DCs (derived from inflammatory monocytes) were identified as the cells responsible for the induction of Th2 responses. In addition, a reduced number of inflammatory DCs in the draining lymph nodes were observed in IRF3-deficient mice immunized with OVA in alum, and the transfer of WT inflammatory monocytes to IRF3-deficient mice increased Th2 cytokine and IgE production. This study also demonstrated that IL-12p80 (a p40 homodimer) is required for alum-induced migration of inflammatory monocytes, and that treatment with anti-IL-12p80 antibody partially attenuated the IgE responses in alum-treated WT mice (). IL-12p80 is known to induce DC migration and to activate nuclear factor kappa-B (NF-κB) and p38 MAP kinase but not signal transducers and activator of transcription (STAT) proteins [Citation77, 78]. Identifying the IL-12p80-producing cells involved in responses to alum or host DNA would be interesting.
IgG1 and IgE responses are uncoupled, i.e. the TBK1-IRF3 axis is required only for the IgE responses. It is believed that the Th2-related antibodies IgE and IgG1 are regulated by identical mechanisms. As described above, PGE2 is only involved in IgE production, not IgG1 production. Although the mechanisms of the regulation of IgE and IgG1 production and the relationship between IRF3 and PGE2 remain unclear, the investigation of these mechanisms may help to improve the adjuvants currently in use.
FUTURE PROSPECTS AND CONCLUSION
A summary of the effects of particulate adjuvants is shown in . Particulate adjuvants (including alum) induce adaptive immunity. The development and modulation of adaptive immunity is regulated by innate immunity. However, the basis for the adjuvanticity of particulates and the mechanisms by which particulates activate innate immunity are not fully understood. Alum has been used as a safe vaccine adjuvant in humans, but the limitations of alum include local reactions and the augmentation of IgE antibody responses [Citation11, Citation79]. These limitations reflect the need for continuing research, and these limitations may be overcome by elucidation of the mechanisms of the effect of particulate adjuvants on immune responses.
Alum in combination with another adjuvant, an AS04, a combination of alum with monophosphoryl lipid A (MPL), has been licensed. In addition, a combination with potent Th1 stimulator such as IL-12 and CpG oligodeoxynucleotides shows a great promise, with improvement in alum-induced Th2 responses [Citation11, 12, Citation80]. Other adjuvant combinations might be explored further. Thus, advances in adjuvant research could open new possibilities for the treatment of not only infectious diseases but also allergic inflammation and cancer.
Declaration of Interest
C.C. and K.J.I are supported by a Health and Labour Sciences Research Grant “Adjuvant Database Project” of the Japanese Ministry of Health, Labour and Welfare. E.K. was supported in part by the Regional Innovation Strategy Support Program and the Grant-in-Aid for Scientific Research from the Ministry of Education, Culture, Sports, Science and Technology of Japan (grant number 24591145).
ABBREVIATIONS | ||
DC | = | dendritic cell |
PAMP | = | pathogen-associated molecular pattern |
PRR | = | pattern-recognition receptor |
TLR | = | Toll-like receptor |
NLR | = | Nod-like receptor |
RLR | = | RIG-I-like receptor |
CLR | = | C-type lectin receptor |
DAMP | = | damage-associated molecular pattern |
Th2 | = | type-2 helper |
IL | = | interleukin |
Ig | = | immunoglobulin |
PGLA | = | poly(lactic-co-glycolic acid) |
MSU | = | monosodium urate |
LPS | = | lipopolysaccharide |
WT | = | wild-type |
OVA | = | ovalbumin |
NKT | = | natural killer T |
TSLP | = | thymic stromal lymphopoietin |
KO | = | knockout |
TNP | = | trinitrophenol |
ASC | = | apoptosis-associated speck-like protein containing a caspase recruitment domain |
Syk | = | spleen tyrosine kinase |
ITAM | = | immunoreceptor tyrosine-based activation motif |
ICAM | = | intracellular adhesion molecule |
LFA | = | leukocyte function-associated antigen |
DAP | = | DNAX-activating protein of 12 kDa |
PG | = | prostaglandin |
cAMP | = | cyclic adenosine monophosphate |
siRNA | = | small interfering RNA |
VIP | = | vasoreactive intestinal polypeptide |
PACAP | = | pituitary adenylate cyclase-activating polypeptide |
COX | = | cyclooxygenase |
PTGES | = | PGE synthase |
IRF3 | = | interferon regulatory factor 3 |
TBK1 | = | TANK-binding kinase 1 |
TNF | = | tumor necrosis factor |
NF-κB | = | nuclear factor kappa-B |
STAT | = | signal transducers and activator of transcription |
MPL | = | monophosphoryl lipid A |
REFERENCES
- Akira S. Innate immunity and adjuvants. Phil Trans R Soc B 2011;366:2748–2755.
- Iwasaki A, Medzhitov R. Regulation of adaptive immunity by the innate immune system. Science 2010;327:291–295.
- Coquerelle C, Moser M. DC subsets in positive and negative regulation of immunity. Immunol Rev 2010;234:317–334.
- Kawai T, Akira S. Toll-like receptors and their crosstalk with other innate receptors in infection and immunity. Immunity 2011;34:637–650.
- Elinav E, Strowig T, Henao-Mejia J, Flavell Richard A. Regulation of the antimicrobial response by NLR proteins. Immunity 2011;34:665–679.
- Loo Y-M, Gale M. Immune signaling by RIG-I-like receptors. Immunity 2011;34:680–692.
- Osorio F, Reis e Sousa C. Myeloid C-type lectin receptors in pathogen recognition and host defense. Immunity 2011;34:651–664.
- Desmet CJ, Ishii KJ. Nucleic acid sensing at the interface between innate and adaptive immunity in vaccination. Nat Rev Immunol 2012;12:479–491.
- Lambrecht BN, Kool M, Willart MAM, Hammad H. Mechanism of action of clinically approved adjuvants. Curr Opin Immunol 2009;21:23–29.
- O'Hagan DT, Ott GS, De Gregorio E, Seubert A. The mechanism of action of MF59—an innately attractive adjuvant formulation. Vaccine 2012;30:4341–4348.
- Gupta RK. Aluminum compounds as vaccine adjuvants. Adv Drug Deliv Rev 1998;32:155–172.
- Aimanianda V, Haensler J, Lacroix-Desmazes S, Novel cellular and molecular mechanisms of induction of immune responses by aluminum adjuvants. Trends Pharmacol Sci 2009;30:287–295.
- Marrack P, McKee AS, Munks MW. Towards an understanding of the adjuvant action of aluminium. Nat Rev Immunol 2009;9:287–293.
- Mancino D, Buono G, Cusano M, Minucci M. Adjuvant effects of a crystalline silica on IgE and IgG1 antibody production in mice and their prevention by the macrophage stabilizer poly-2-vinylpyridine N-oxide. Int Arch Allergy Appl Immunol 1983;71:279–281.
- Sharp FA, Ruane D, Claass B, Uptake of particulate vaccine adjuvants by dendritic cells activates the NALP3 inflammasome. Proc Natl Acad Sci USA 2009;106:870–875.
- Nygaard UC, Aase A, Lovik M. The allergy adjuvant effect of particles—genetic factors influence antibody and cytokine responses. BMC Immunol 2005;6:11.
- Kuroda E, Ishii KJ, Uematsu S, Silica crystals and aluminum salts regulate the production of prostaglandin in macrophages via NALP3 inflammasome-independent mechanisms. Immunity 2011;34:514–526.
- Inoue K, Koike E, Yanagisawa R, Effects of multi-walled carbon nanotubes on a murine allergic airway inflammation model. Toxicol Appl Pharmacol 2009;237:306–316.
- Nygaard UC, Hansen JS, Samuelsen M, Single-walled and multi-walled carbon nanotubes promote allergic immune responses in mice. Toxicol Sci 2009;109:113–123.
- Ichinose T, Takano H, Miyabara Y, Murine strain differences in allergic airway inflammation and immunoglobulin production by a combination of antigen and diesel exhaust particles. Toxicology 1997;122:183–192.
- Lovik M, Hogseth AK, Gaarder PI, Diesel exhaust particles and carbon black have adjuvant activity on the local lymph node response and systemic IgE production to ovalbumin. Toxicology 1997;121:165–178.
- Kool M, Soullie T, van Nimwegen M, Alum adjuvant boosts adaptive immunity by inducing uric acid and activating inflammatory dendritic cells. J Exp Med 2008;205:869–882.
- Behrens MD, Wagner WM, Krco CJ, The endogenous danger signal, crystalline uric acid, signals for enhanced antibody immunity. Blood 2008;111:1472–1479.
- Willart MAM, Lambrecht BN. The danger within: endogenous danger signals, atopy and asthma. Clin Exp Allergy 2009;39:12–19.
- Kool M, Willart Monique AM, van Nimwegen M, An unexpected role for uric acid as an inducer of T helper 2 cell immunity to inhaled antigens and inflammatory mediator of allergic asthma. Immunity 2011;34:527–540.
- Kool M, Hammad H, Lambrecht B. Cellular networks controlling Th2 polarization in allergy and immunity. F1000 Biol Rep 2012;4:6.
- Coban C, Igari Y, Yagi M, et al.. Immunogenicity of whole-parasite vaccines against Plasmodium falciparum involves malarial hemozoin and host TLR9. Cell Host Microbe 2010;7:50–61.
- Reese TA, Liang H-E, Tager AM, Chitin induces accumulation in tissue of innate immune cells associated with allergy. Nature 2007;447:92–96.
- Hornung V, Bauernfeind F, Halle A, Silica crystals and aluminum salts activate the NALP3 inflammasome through phagosomal destabilization. Nat Immunol 2008;9:847–856.
- Coban C, Yagi M, Ohata K, The malarial metabolite hemozoin and its potential use as a vaccine adjuvant. Allergol Int 2010;59:115–124.
- Glenny AT, Pope CG, Waddington H, Wallace U. Immunological notes XVLL-XXIV. J Pathol Bacteriol 1926;29(1):31–40.
- Harrison WT. Some observations on the use of alum precipitated diphtheria toxoid. Am J Public Health Nations Health 1935;25:298–300.
- Holt LB. Developments in diphtheria prophylaxis. London: Heinemann; 1950.p. xvi; 181.
- Hutchison S, Benson RA, Gibson VB, Antigen depot is not required for alum adjuvanticity. FASEB J 2011;26:1272–1279.
- Jordan MB. Promotion of B cell immune responses via an alum-induced myeloid cell population. Science 2004;304(5678):1808–1810.
- Wang HB, Weller PF. Pivotal advance: eosinophils mediate early alum adjuvant-elicited B cell priming and IgM production. J Leukoc Biol 2008;83:817–821.
- McKee AS, Munks MW, MacLeod MKL, Alum induces innate immune responses through macrophage and mast cell sensors, but these sensors are not required for alum to act As an adjuvant for specific immunity. J Immunol 2009;183:4403–4414.
- Ohnmacht C, Schwartz C, Panzer M, Basophils orchestrate chronic allergic dermatitis and protective immunity against helminths. Immunity 2010;33:364–374.
- Shah HB, Devera TS, Rampuria P, Type II NKT cells facilitate alum-sensing and humoral immunity. J Leukoc Biol 2012;92:883–893.
- Brewer JM, Conacher M, Satoskar A, In interleukin-4-deficient mice, alum not only generates T helper 1 responses equivalent to Freund's complete adjuvant, but continues to induce T helper 2 cytokine production. Eur J Immunol 1996;26:2062–2066.
- Brewer JM, Conacher M, Hunter CA, Aluminium hydroxide adjuvant initiates strong antigen-specific Th2 responses in the absence of IL-4- or IL-13-mediated signaling. J Immunol 1999;163:6448–6454.
- Al-Shami A, Spolski R, Kelly J, A role for TSLP in the development of inflammation in an asthma model. J Exp Med 2005;202:829–839.
- Chen ZG, Zhang TT, Li HT, Neutralization of TSLP inhibits airway remodeling in a murine model of allergic asthma induced by chronic exposure to house dust mite. PLoS ONE 2013;8:e51268.
- Nakajima S, Igyarto BZ, Honda T, Langerhans cells are critical in epicutaneous sensitization with protein antigen via thymic stromal lymphopoietin receptor signaling. J Allergy Clin Immunol 2012;129:1048–1055e6.
- Schnare M, Barton GM, Holt AC, Toll-like receptors control activation of adaptive immune responses. Nat Immunol 2001;2:947–950.
- Gavin AL, Hoebe K, Duong B, Adjuvant-enhanced antibody responses in the absence of toll-like receptor signaling. Science 2006;314:1936–1938.
- Da Silva CA, Pochard P, Lee CG, Elias JA. Chitin particles are multifaceted immune adjuvants. Am J Respir Crit Care Med 2010;182:1482–1491.
- Eisenbarth SC, Colegio OR, O'Connor W, Crucial role for the Nalp3 inflammasome in the immunostimulatory properties of aluminium adjuvants. Nature 2008;453:1122–1126.
- Dostert C, Petrilli V, Van Bruggen R, Innate immune activation through Nalp3 inflammasome sensing of asbestos and silica. Science 2008;320:674–677.
- Martinon F, Petrilli V, Mayor A, Gout-associated uric acid crystals activate the NALP3 inflammasome. Nature 2006;440:237–241.
- Tiemi Shio M, Eisenbarth SC, Savaria M, Malarial hemozoin activates the NLRP3 inflammasome through Lyn and Syk kinases. PLoS Pathol 2009;5:e1000559.
- Dostert C, Guarda G, Romero JF, Malarial hemozoin is a Nalp3 inflammasome activating danger signal. PLoS ONE 2009;4:e6510.
- Griffith JW, Sun T, McIntosh MT, Bucala R. Pure hemozoin is inflammatory in vivo and activates the NALP3 inflammasome via release of uric acid. J Immunol 2009;183:5208–5220.
- Li H, Willingham SB, Ting JP, Re F. Cutting edge: inflammasome activation by alum and alum's adjuvant effect are mediated by NLRP3. J Immunol 2008;181:17–21.
- Kool M, Petrilli V, De Smedt T, Cutting edge: alum adjuvant stimulates inflammatory dendritic cells through activation of the NALP3 inflammasome. J Immunol 2008;181:3755–3759.
- Franchi L, Núñez G. The Nlrp3 inflammasome is critical for aluminium hydroxide-mediated IL-1β secretion but dispensable for adjuvant activity. Eur J Immunol 2008;38:2085–2089.
- Pollock KG, Conacher M, Wei XQ, Interleukin-18 plays a role in both the alum-induced T helper 2 response and the T helper 1 response induced by alum-adsorbed interleukin-12. Immunology 2003;108:137–143.
- Shi Y, Evans JE, Rock KL. Molecular identification of a danger signal that alerts the immune system to dying cells. Nature 2003;425:516–521.
- Shi Y, Mucsi AD, Ng G. Monosodium urate crystals in inflammation and immunity. Immunol Rev 2010;233:203–217.
- Mócsai A, Ruland J, Tybulewicz VLJ. The SYK tyrosine kinase: a crucial player in diverse biological functions. Nat Rev Immunol 2010;10:387–402.
- Ng G, Sharma K, Ward SM, Receptor-independent, direct membrane binding leads to cell-surface lipid sorting and Syk kinase activation in dendritic cells. Immunity 2008;29:807–818.
- Flach TL, Ng G, Hari A, Alum interaction with dendritic cell membrane lipids is essential for its adjuvanticity. Nat Med 2011;17:479–487.
- Narumiya S. Prostanoids and inflammation: a new concept arising from receptor knockout mice. J Mol Med (Berlin) 2009;87:1015–1022.
- Fabricius D, Neubauer M, Mandel B, Prostaglandin E2 inhibits IFN-alpha secretion and Th1 costimulation by human plasmacytoid dendritic cells via E-prostanoid 2 and E-prostanoid 4 receptor engagement. J Immunol 2010;184:677–684.
- Kuroda E, Yamashita U. Mechanisms of enhanced macrophage-mediated prostaglandin E2 production and its suppressive role in Th1 activation in Th2-dominant BALB/c mice. J Immunol 2003;170:757–764.
- Koga K, Takaesu G, Yoshida R, Cyclic adenosine monophosphate suppresses the transcription of proinflammatory cytokines via the phosphorylated c-Fos protein. Immunity 2009;30:372–383.
- Boniface K, Bak-Jensen KS, Li Y, Prostaglandin E2 regulates Th17 cell differentiation and function through cyclic AMP and EP2/EP4 receptor signaling. J Exp Med 2009;206:535–548.
- Yao C, Sakata D, Esaki Y, Prostaglandin E2-EP4 signaling promotes immune inflammation through Th1 cell differentiation and Th17 cell expansion. Nat Med 2009;15:633–640.
- Uematsu S, Matsumoto M, Takeda K, Akira S. Lipopolysaccharide-dependent prostaglandin E(2) production is regulated by the glutathione-dependent prostaglandin E(2) synthase gene induced by the Toll-like receptor 4/MyD88/NF-IL6 pathway. J Immunol 2002;168:5811–5816.
- Fedyk ER, Phipps RP. Prostaglandin E2 receptors of the EP2 and EP4 subtypes regulate activation and differentiation of mouse B lymphocytes to IgE-secreting cells. Proc Natl Acad Sci USA 1996;93:10978–10983.
- Roper RL, Brown DM, Phipps RP. Prostaglandin E2 promotes B lymphocyte Ig isotype switching to IgE. J Immunol 1995;154:162–170.
- Delgado M, Leceta J, Gomariz RP, Ganea D. Vasoactive intestinal peptide and pituitary adenylate cyclase-activating polypeptide stimulate the induction of Th2 responses by up-regulating B7.2 expression. J Immunol 1999;163:3629–3635.
- Delgado M, Gomariz RP, Ganea D. VIP/PACAP preferentially attract Th2 effectors through differential regulation of chemokine production by dendritic cells. FASEB J 2004;18:1453–1455.
- Traidl-Hoffmann C, Mariani V, Hochrein H, Pollen-associated phytoprostanes inhibit dendritic cell interleukin-12 production and augment T helper type 2 cell polarization. J Exp Med 2005;201:627–636.
- Mariani V, Gilles S, Jakob T, Immunomodulatory mediators from pollen enhance the migratory capacity of dendritic cells and license them for Th2 attraction. J Immunol 2007;178:7623–7631.
- Marichal T, Ohata K, Bedoret D, DNA released from dying host cells mediates aluminum adjuvant activity. Nat Med 2011;17:996–1002.
- Cooper AM, Khader SA. IL-12p40: an inherently agonistic cytokine. Trends Immunol 2007;28:33–38.
- Robinson RT, Khader SA, Locksley RM, Yersinia pestis evades TLR4-dependent induction of IL-12(p40)2 by dendritic cells and subsequent cell migration. J Immunol 2008;181:5560–5567.
- Nagel J, Svec D, Waters T, Fireman P. IgE synthesis in man. I. Development of specific IgE antibodies after immunization with tetanus-diphtheria (Td) toxoids. J Immunol 1977;118:334–341.
- Lindblad EB. Aluminium compounds for use in vaccines. Immunol Cell Biol 2004;82:497–505.